Abstract
Despite the remarkable progress recently made to enhance the resolution of white-light endoscopy, detection, and diagnosis of premalignant lesions, such as adenomas and subtle early-stage cancers, remains a great challenge. As for example, although chromoendoscopy, such as endoscopy using indigo carmine, is useful for the early diagnosis of subtle lesions, the technique presents various disadvantages ranging from the time required for spray application of the dye and suctioning of excess dye to the increased difficulty in identifying lesions in the presence of severe inflammation and obstruction of visual field due to the pooling of solution in depressed-type lesions. To overcome these diagnostic problems associated with chromoendoscopy, research has focused on the development of endoscopes based on new optical technologies. Several types of image-enhanced endoscopy methods have recently been presented. In particular, image-enhanced endoscopy has emerged as a new paradigm for the diagnosis of gastrointestinal disorders. Image-enhanced endoscopes provide high-contrast images of lesions by means of optical or electronic technologies, including the contrast enhancement of the mucosal surface and of blood vessels. Chromoendoscopy, narrow-band imaging, i-SCAN, and flexible spectral imaging color enhancement are representative examples of image-enhanced endoscopy discussed in this paper.
Gastrointestinal (GI) cancer is one of the leading causes of cancer-related deaths worldwide. Early detection and treatment of malignant lesions can enhance the survival rates of patients with GI cancer. To promote early detection of GI cancers, it is essential to expand the population of asymptomatic individuals receiving periodic screening, as well as to use diagnostic tools that have high sensitivity and specificity to detect subtle lesions. Endoscopy plays an important role in improving the early detection rate of GI cancer, providing an easy and accurate method of diagnosis. Although the recent development of high-resolution electronic endoscopy has facilitated the diagnosis of subtle cancers, differentiating between inflamed and neoplastic tissues by visual inspection remains a barrier in chromoendoscopy (CE), with biopsy being required to confirm diagnoses. The need and wish for improving the differentiation of malignant and inflammatory lesions by endoscopy has fueled research to accelerate the development of novel types of endoscopy systems based on new optical technologies. The recently developed ‘image-enhanced endoscopy’ (IEE) encompasses various means of enhancing contrast during endoscopy using dye, optical, and/or electronic methods. Therefore, IEE provides easier assessment and specificity of the morphology of a lesion by highlighting the mucosal microstructure and microvasculature features that are needed for adequate and accurate GI cancer diagnosis and treatment.
IEE can be classified into dye-based CE and electronic CE methods, with narrow-band imaging (NBI), i-SCAN, and flexible spectral imaging color enhancement (FICE) being examples of electronic-based CE. The aim of this article is to give a brief history of the development endoscopy systems, to describe the principles of currently available IEE methods, including their advantages and disadvantages, and to estimate the potential for further development. This review of IEE, however, will not include critical appraisal of the currently available evidence of the clinical outcomes of IEE for the identification and treatment of GI diseases.
In 1957, Hirchowitz et al. used a flexible, fiber-optic, endoscope to visually inspect the GI tract for the first time [1]. In fiber-optic endoscopes, various optical viewing bundles are used to transmit images. Images transmitted to the top of the bundles by each optical fiber yield an image through a focusing lens, with the resulting image viewed through an eyepiece. Optical fibers are prone to damage and damaged optical fiber bundles affect the clarity of the image. To overcome this problem, video-endoscopy was developed in 1990s, using charge-coupled devices (CCDs) [1]. CCDs consist of an array of individual photocells, called picture elements or pixels, that receive photons reflected back from the mucosal surface and produce electrons in proportion to the light reflected on the CCDs. The variable charge thus generated is electronically transmitted to a video processor and converted into a digital image. Pixel density is particularly important as it is directly associated with the capacity to distinguish two adjacent points, a property known as image resolution. The resolution of images depends on the number of pixels in the array, with higher the pixel density providing a higher resolution for detailed images of a lesion. Standard definition endoscopes are equipped with CCD chips that produce image signals with a resolution of 100,000 to 400,000 pixels. High-resolution, or high-definition (HD), endoscopes produce signal images with resolutions of up to million pixels [2], which has the same effect as visualizing a surface at a 30- to 35-fold magnification (Fig. 1). Images can be further magnified by moving the motor-driven optimal lens at the tip of the endoscope very close to the mucosal surface, controlling for the focal distance. The magnification achieved by moving the optical lens closer to the area of interest is termed optical zooming, and provides an optical magnification quality that is distinctly superior to electronic magnification, which simply enlarges the screen size without improving resolution. In other words, optical zooming produces genuinely magnified close-up images of lesions without losing pixel density. A translucent cap on the tip of the endoscope may be used to stabilize the focal length between the lens and the target tissue to improve image quality [3]. HD endoscopy is categorized as IEE because it allows the visualization of lesions at a 30- to 35-fold magnification; thus, allowing examination of subtle changes in lesions, which was not previously possible. In contrast to HD endoscopes, magnifying endoscopes (ME) can be used to examine changes in the mucosal microstructure and microvasculature by providing a 150-fold image magnification, making histological diagnosis of a lesion possible by visual inspection (Fig. 2). As such, ME provides a step forward towards a final goal of high-end endoscopy for reliable and specific screening of general populations.
CE is an endoscopy technology that consists of spray application of dyes, which are harmless to the human body, onto the mucosal surface of interest. The application of dye improves visualization of the microstructure and vascular patterns of lesions under investigation. Therefore, the main purpose of CE in the screening of malignant and premalignant lesions is to enhance diagnostic accuracy by clearly delineating the boundaries of a lesion to determine the adequate region for biopsy [4]. The CE is equipped with only a spray catheter, providing a relatively simple and cost-effective method of dye application. Despite these advantages, the application of CE to screening programs remains limited due to absence of standardized methods and analysis, resulting in uncertainty in lesion identification. Stains are used to enhance the tissue resolution of CE. Stains are classified according to their mechanism of action into absorptive (or vital) stains, contrast stains and reactive stains. Absorptive stains are absorbed into the cells, with selective absorption of specific stains by different cell types. Common stains used for CE include Lugol’s solution, methylene blue, toluidine blue, cresyl violet, and crystal violet. Lugol’s solution, which is the most commonly used stain for absorptive CE, was first used by Schiller to diagnose cervical cancer. Voegeli used Lugol’s solution in 1966 for the first time to diagnose esophageal cancer [4]. Lugol’s solution contains potassium iodine, and the iodine reacts with the glycogen in the non-keratinized squamous epithelium, with normal esophageal mucosa being stained deeply brown. In the presence of malignancy or inflammation of the esophagus, no staining occurs due to the low glycogen level in the squamous epithelium. Therefore, any unstained area of the esophagus after spraying of Lugol’s solution can be identified as a premalignant lesion, cancer, inflammation, or fibrous tissue. Lugol’s solution has been used to delineate the extent of Barrett’s esophagus [5], and to screen for squamous cell cancer in the esophagus in high risk populations (Fig. 3) [6].
Methylene blue was first used by Kida et al. [7] as an enhanced diagnostic method to detect early malignant lesions in gastric cancer. Methylene blue is absorbed by tissues of the small intestine or colon and, therefore, can be used as an absorptive stain for these organs. Although methylene blue is not absorbed by tissues of the esophagus and stomach, it can be used as a contrast agent to reveal the surface profile of lesions. Canto et al. [8] used methylene blue to diagnose Barrett’s esophagus, based on evidence of its selectively blue staining of some specialized intestinal metaplastic cells. A concentration of 0.1% to 0.5% of methylene blue solution can positively identify 75% of Barrett’s esophageal lesions [8].
Contrast staining is the most commonly used staining method. Instead of being absorbed into cells, contrast stains highlight the surface profile of lesions by accumulating in pits and valleys between cells. Indigo carmine stain is most commonly used for contrast staining. Indigo carmine stain was originally used to assess kidney function. Although not absorbed by the cells, indigo carmine stain, sprayed onto regions of interest, highlights the topography of the mucosal surface with its blue coloring. Generally used at a concentration around 0.2%, indigo carmine stain is useful as a screening method for diagnosing minute lesions, to differentiate between benign and malignant lesions, as well as to facilitate application of ME to observe and analyze the surface structure of a lesion, delineate boundaries of early stage malignant lesions and estimate the invasion depth of cancer. It has been used to diagnose Barrett’s esophagus [9], evaluate villous atrophy [10], diagnose and discriminate polypoid and non-polypoid lesions in the colon [11], and diagnose gastric adenoma and cancer (Fig. 4). Reactive stains change colors based on their chemical reaction with gastric acid. Therefore, reactive stains are useful for determining the presence/absence of Helicobacter pylori and used in the diagnosis of hypochlorhydria. Phenol red and Congo red are commonly used reactive stains, with the red being expressed in an alkaline environment indicative of H. pylori infection.
Unlike fiber endoscopes that directly capture optical signals, electronic endoscopes obtain images in the form of electronic signals that can be analyzed using various image-processing techniques. Image enhancement is an image processing technique commonly used to improve diagnostic accuracy by converting color and structure-based diagnostic information into more objective and quantitative indicators. Electronic CE, therefore, eliminate the need for time-consuming methods of CE, including spraying and suctioning of dyes and the disadvantage of solution pooling in depressed-type lesions that obstruct visual inspection. Various electronic CE are currently used, including the NBI, which selectively transmits a specific range of light using an optical filter, and the i-SCAN and FICE systems, which implement this process using software-driven post-image processing.
The basic physical principle underlying NBI is that the depth of light penetration into a tissue is proportional to the wavelength used: blue bandwidth for superficial tissues, red bandwidth for deep tissue, and green bandwidth for intermediate tissues [12]. The blue shortwave visible light penetrates only superficially into the mucosa; it is not reflected as it is absorbed by the hemoglobin of the red blood cells in blood vessels. Early GI cancer typically originates in the mucosa and the lesions are well supplied by blood vessels. This associated angiogenesis is considered an important factor in cancer formation and metastasis. Therefore, differentiation of even small differences in the vascular pattern of normal tissue and mucosal lesions would be important in GI screening for cancer [13]. The capillary bed of the mucosal surface can be observed more clearly when illuminated with narrow-band blue (415±15 nm) and green (540±15 nm) wavelengths.
NBI was first developed by Olympus Medical Systems using these characteristics. The NBI system (EVIS LUCERA SPECTRUM system, CV-260SL; Olympus Medical Systems Co. Ltd., Tokyo, Japan) has a filter that transmits only wavelength of specific bandwidths: 415±15 and 540±15 nm. In application, the change from white light endoscopy to NBI is easily activated by a ‘switch’ that inserts the fixed bandwidth filter to the end of the endoscope without disrupting visual assessments of the upper GI system. Images are produced by the difference in amount and wavelength of the light reflected back to the monochromic CCD chip by contrasting areas. As black-and-white images have low resolutions, the patterns of light reflected into the monochromic CCD are resynthesized as red, green, blue (RGB) colors to generate color images. Therefore, the main imaging colors are brown and green, and the lesions can be observed at a higher resolution (Fig. 5). However, the images produced by NBI systems are darker and coarser than white-light images. Although sharp images can be obtained with the dark light source of shortwave visible light (400 to 500 nm) of the NBI in the esophagus or colon, which have a narrow lumen, images in organs with a wide lumen, such as the stomach, will be coarse and dark, making NBI unsuitable for identification and diagnosis in these situations. To overcome this drawback, the next-generation of NBI systems, the Evis Exera III (United States and Europe) and Evis Lucera Elite (Korea and Japan), entered the market in 2012. These NBI systems use an upgraded xenon lamp as a light source in combination with two filters transmitting blue light and one filter transmitting green light, compared to one filter each for blue and green light in basic NBI systems. With the combination of upgraded light source and an additional blue light filter, these new NBI scopes can achieve near-focus and a high-resolution macrofunction, producing brighter and clearer images. Near-focus yields a low intensity zoom magnification, improving the capacity of NBI endoscopes to detect lesions and to discern the fine details of the mucosa, especially the mucosal microsurface and microvascular patterns (Fig. 6).
Clinically, NBI provide a method of optical or digital CE and can be used to perform optical biopsies, enabling diagnosis via visual assessment only, without the need to perform tissue biopsy [14]. The NBI overcome the drawbacks associated with staining methods, such as time-consuming spraying and rinsing and the pooling of staining solution in depressed-type lesions that obstruct visualization, simply by switching to NBI mode. The diagnostic performance of NBI has been shown to be comparable to the performance of CE. Moreover, use of NBI in combination with ME improves the clarity and accuracy of visually based assessment of mucosal microvasculature and microstructure. As examples, pitting surfaces of colon polyps can be observed with more accuracy, enabling the clinician to differentiate between adenomatous polyps, which require removal, and inflammatory or hyperplastic polyps, which do not need to be removed, without biopsy. Similarly, NBI has greatly facilitated visualization of the boundaries of Barrett’s esophagus and of the gastroesophageal junction, improving the diagnosis of gastroesophageal reflux disease, which cannot easily be discerned by conventional white-light endoscopy. However, NBI-based assessment of organs that have a large lumen, such as the stomach, is not possible with the dark light source. Furthermore, the single-use applicability of NBI systems in the assessment of gastric lesions has not yet been validated. However, the recent use of NBI in combination with ME has replaced the need for stain spraying to differentiate the microstructural and microvascular patterns of gastric mucosa, reducing the time of endoscopic examination and upgrading conventional ME [15].
i-SCAN is a software-based digital post-processing image-enhanced technology developed by PENTAX (Tokyo, Japan) [16]. i-SCAN performs three functions: surface enhancement (SE), contrast enhancement (CE), and tone enhancement (TE). Of these three functions, SE enhances light-dark contrast on the basis of luminance intensity data obtained from each pixel. This SE function is useful for visual inspection of subtle changes in the mucosa and improving the identification and examination of lesions. This function is comparable to the structure enhancement feature of Olympus endoscopes (Tokyo, Japan), and can yield similar outcomes to spraying of acetic acid in CE, which highlights changes in the mucosal surface by adding blue hue to darker regions based on the luminance intensity data obtained from each pixel.
TE divides the images obtained using normal white light into its RGB components, and subsequently converts and resynthesizes them into a new image to produce images in which subtle hue differences highlight vascular patterns or subtle changes in the mucosa. TE can be implemented in six different modes (TE p, TE v, TE b, TE e, TE g, and TE c) that vary the degree of conversion of each color component to optimize imaging to target organs. TE v and TE p were the original main modes of TE, with TE b, TE e, TE g, and TE c modes more recently added to the TE suite. TE p highlights relatively dark tone red to distinguish the mucosal surface and, therefore, is suitable for observing pit pattern. TE v in contrast is tailored to the observation of vessel morphology by highlighting lesser red regions with the use of faded red color. Therefore, TE p and v perform similarly to NBI in filtering red coloring. TE b is similar to TE p in that it highlights dark red but it was designed specifically to facilitate detection of Barrett’s esophagus. TE e is the best-suited mode for esophagus observation as it highlights reddish and whitish mucosal regions by fading the red color. TE g is similar to TE e in that it highlights dark red and white mucosal regions by fading the red color, with specific adjustments for gastric observations (Fig. 7). While TE c is similar to TE g, it has a broader visible red light band than TE g which facilitates observation of the mucosal structure of polyps, as well as the pitting pattern of the mucosal surface, in the assessment of the colon. Although there are only a small number of studies that have investigated the usability of i-SCAN systems for GI cancer diagnosis to date, it is expected that further research will prove i-SCAN systems to be as useful as other IEE technologies [17].
The color images obtained from electronic endoscopy are ultimately converted into digital format. Recent advances in image processing technology has made it possible to estimate the specific wavelengths of light forming images, a process known as spectral estimation technology. FICE is a proprietary digital imaging post-processing system of Fujinon (Tokyo, Japan). As FICE uses a relatively wide range of spectral combinations, it also often called multi-band imaging or optimal band imaging (Fig. 8). FICE enhances the visualization of mucosal structure and microcirculation by the selection of spectral transmittance with a dedicated wavelength [18,19].
The human eye can detect visible light in the frequency range of 400 and 700 nm. Comparatively, FICE can produce 60 types of spectral images in the visible light band of 400 to 695 nm at 5-nm intervals, with the possibility of further refining each spectral image into five intensities. Therefore, FICE can produce 300 types of spectral images of five different intensities and 60 different wavelengths. Theoretically, FICE systems can, therefore, produce 3003 (or 2,700×104) images from one endoscopic image.
FICE systems at present, provides 10 presettings, numbered from 0 to 9, as default values on their board. These factory default modes were determined by configuring the spectral images to optimize bandwidth for the following four applications: (1) absorption band for vascular regions (around 400 to 420 nm); (2) reflection band for vascular regions (around 470 nm); (3) reflection band for mucosal surfaces (around 500 nm); and (4) absorption band for arterial blood, specifically oxyhemoglobin (550 nm). Use of FICE is extremely simple. In the same manner as the NBI conversion of the Olympus system, white-light images can be instantaneously converted to FICE images by pressing a switch on the endoscope. For ease of use, the 10 preset FICE default values can be selected by pressing the number keys (F1–F10). This allows change in the bandwidth of RGB imaging signals to be implemented in real time.
The limited research currently available on FICE devices indicates the superiority of FICE images over white-light images in terms of resolution and adaptability to different tissues. The optimal FICE presets for tissue diagnosis or differentiation have not been established [20]. In fact, the FICE system has been shown to be limited in producing high-contrast images of the microvasculature on the mucosal surface. The blue laser imaging (BLI) system (Fujifilm Co., Kanagawa, Japan) was recently developed to address this problem [21]. BLI is the first endoscopic system to use laser as the light source, allowing the system to produce white-light images as well as NBI, based on the properties of laser light. Compared to the conventional xenon lamp source, laser light also has low power consumption and extremely low heat generation.
The BLI system uses two laser beams of different wavelengths, allowing the light source that is best suited for a target tissue to be selected by adjusting the ratio of the two laser intensities: white-light laser and BLI modes. The whitelight mode laser (peak wavelength, 450±10 nm) produces a wide spectrum light source suitable for normal observation, with the shortwave narrow-band laser light source (BLI laser, peak wavelength, 410±10 nm) producing a narrow spectrum to highlight the microvasculature of the mucosal surface to identify subtle changes in the mucosal surface, key information for cancer diagnosis.
The efficacy of IEE for the diagnosis and treatment of early-phase esophageal squamous carcinoma and the treatment of early gastric cancer (Figs. 9, 10) and colonic tumors have been reported in various studies. Factors potentially impeding a wide distribution of IEE are perceived inefficiencies in technology and application, heavy economic burden combined with inadequate compensation for application, absence of standardized training modules, and absence of high-quality comparative studies. To address these problems, future studies will have to focus on the following issues: (1) justifying the cost-benefit tradeoff of IEE by providing objective data to determine if IEE can increase diagnostic accuracy and reduce the need for biopsies; (2) developing useful training modules for NBI, FICE, and i-SCAN systems; (3) optimizing FICE and i-SCAN settings to adapt imaging to the location and structure of lesions; (4) validating the clinical usability of the next-generation of electronic CEs; and (5) establishing consensus in application, image processing and image analysis based on multicenter trials and developing disease-specific classification systems.
Early detection and treatment of GI cancer is the key factor for improving patients’ prognosis and increasing his/her quality of life. Consequently, the early detection and treatment of GI cancer is an important duty of physicians offering endoscopic screening and diagnosis. In clinical studies, IEE outperformed conventional white-light endoscopy in diagnosing lesions and estimating their morphologies. However, identification remains difficult, even impossible, for selected lesions in certain organs. These limitations will improve the continued enhancement of image resolution and it is hoped that useful results will be derived from large-scale studies on various organs and lesions. It is also hoped that the technology of IEE will continue to advance towards the clinical goal of using IEE to perform “optical biopsies” capable of predicting pathological outcomes and, thereby, eliminating the need for tissue biopsies.
REFERENCES
1. Subramanian V, Ragunath K. Advanced endoscopic imaging: a review of commercially available technologies. Clin Gastroenterol Hepatol. 2014; 12:368.e1–376.e1.


2. ASGE Technology Committee, Kwon RS, Adler DG, et al. High-resolution and high-magnification endoscopes. Gastrointest Endosc. 2009; 69(3 Pt 1):399–407.


3. Bruno MJ. Magnification endoscopy, high resolution endoscopy, and chromoscopy; towards a better optical diagnosis. Gut. 2003; 52 Suppl 4:iv7–iv11.


4. Peitz U, Malfertheiner P. Chromoendoscopy: from a research tool to clinical progress. Dig Dis. 2002; 20:111–119.


5. Woolf GM, Riddell RH, Irvine EJ, Hunt RH. A study to examine agreement between endoscopy and histology for the diagnosis of columnar lined (Barrett’s) esophagus. Gastrointest Endosc. 1989; 35:541–544.


6. Inoue H, Rey JF, Lightdale C. Lugol chromoendoscopy for esophageal squamous cell cancer. Endoscopy. 2001; 33:75–79.
7. Kida M, Kobayashi K, Saigenji K. Routine chromoendoscopy for gastrointestinal diseases: indications revised. Endoscopy. 2003; 35:590–596.


8. Canto MI, Setrakian S, Petras RE, Blades E, Chak A, Sivak MV Jr. Methylene blue selectively stains intestinal metaplasia in Barrett’s esophagus. Gastrointest Endosc. 1996; 44:1–7.


9. Stevens PD, Lightdale CJ, Green PH, Siegel LM, Garcia-Carrasquillo RJ, Rotterdam H. Combined magnification endoscopy with chromoendoscopy for the evaluation of Barrett’s esophagus. Gastrointest Endosc. 1994; 40:747–749.


10. Siegel LM, Stevens PD, Lightdale CJ, et al. Combined magnification endoscopy with chromoendoscopy in the evaluation of patients with suspected malabsorption. Gastrointest Endosc. 1997; 46:226–230.


11. Axelrad AM, Fleischer DE, Geller AJ, et al. High-resolution chromoendoscopy for the diagnosis of diminutive colon polyps: implications for colon cancer screening. Gastroenterology. 1996; 110:1253–1258.


12. Sano Y, Muto M, Tajiri H, Ohtsu A, Yoshida S. Optical/digital chromoendoscopy during colonoscopy using narrow-band imaging system. Dig Endosc. 2005; 17 Suppl 1:S43–S48.


13. Muto M, Katada C, Sano Y, Yoshida S. Narrow band imaging: a new diagnostic approach to visualize angiogenesis in superficial neoplasia. Clin Gastroenterol Hepatol. 2005; 3(7 Suppl 1):S16–S20.


14. Cho WY, Jang JY, Lee DH; Endoscopic Technology and Investigation Study Group. Recent advances in image-enhanced endoscopy. Clin Endosc. 2011; 44:65–75.


15. Tajiri H, Matsuda K, Fujisaki J. What can we see with the endoscope? Present status and future perspectives. Dig Endosc. 2002; 14:131–137.


16. Kodashima S, Fujishiro M. Novel image-enhanced endoscopy with i-scan technology. World J Gastroenterol. 2010; 16:1043–1049.


17. Lee BI. EPK-i Endoscopy. Korean J Gastrointest Endosc. 2009; 39(Suppl 1):184–186.
18. Yoshizawa M, Osawa H, Yamamoto H, et al. Diagnosis of elevated-type early gastric cancers by the optimal band imaging system. Gastrointest Endosc. 2009; 69:19–28.


19. Osawa H, Yoshizawa M, Yamamoto H, et al. Optimal band imaging system can facilitate detection of changes in depressed-type early gastric cancer. Gastrointest Endosc. 2008; 67:226–234.


Fig. 1.
The resolution of endoscopic image. (A) Standard definition (SD) endoscopic image of gastroesophageal (GE) junction, (B) high definition endoscopic image of GE junction can be observed more clearly compared to the SD endoscopic image.
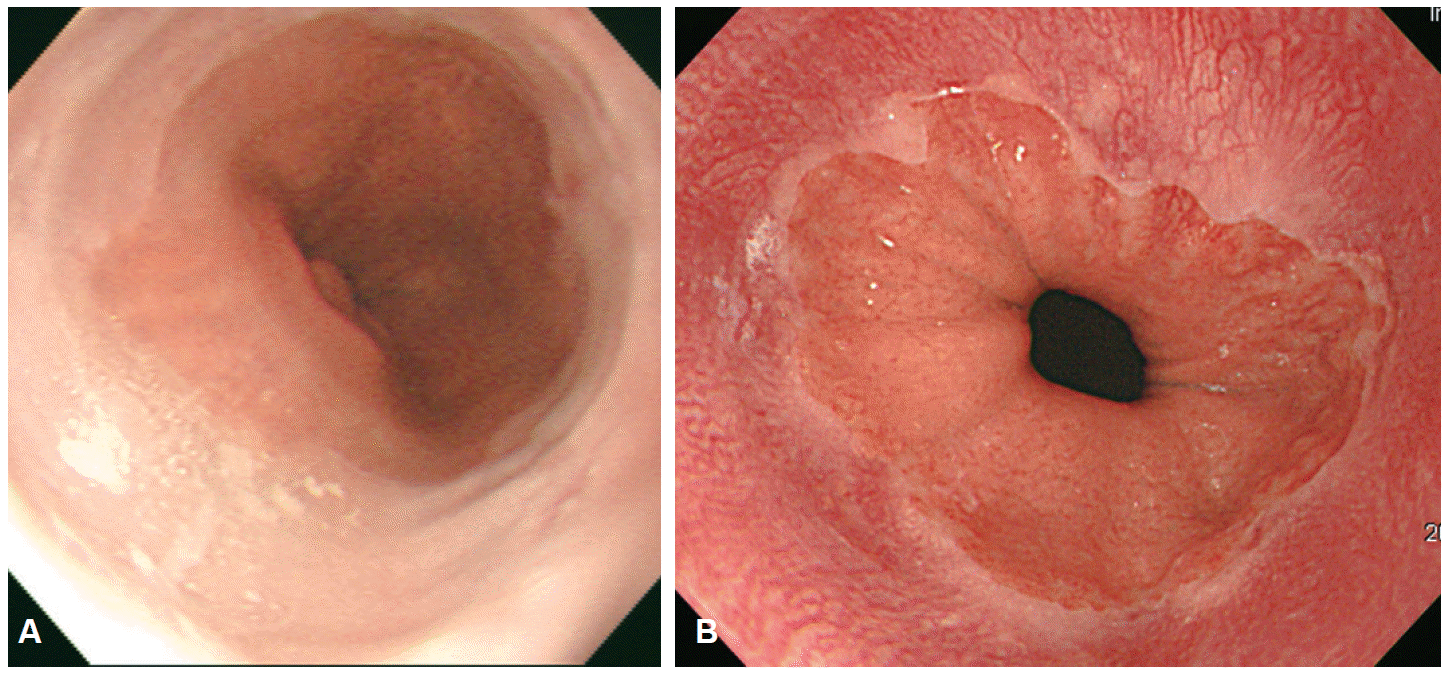
Fig. 2.
Magnifying endoscopic finding of normal gastric mucosa. (A) In the gastric antrum, microvascular architecture is a coil-shaped. The pits demonstrate a linear or reticular patterns. (B) In the gastric body, microvascular architecture is a honeycomb shaped, and the pits demonstrate a round or oval shape.
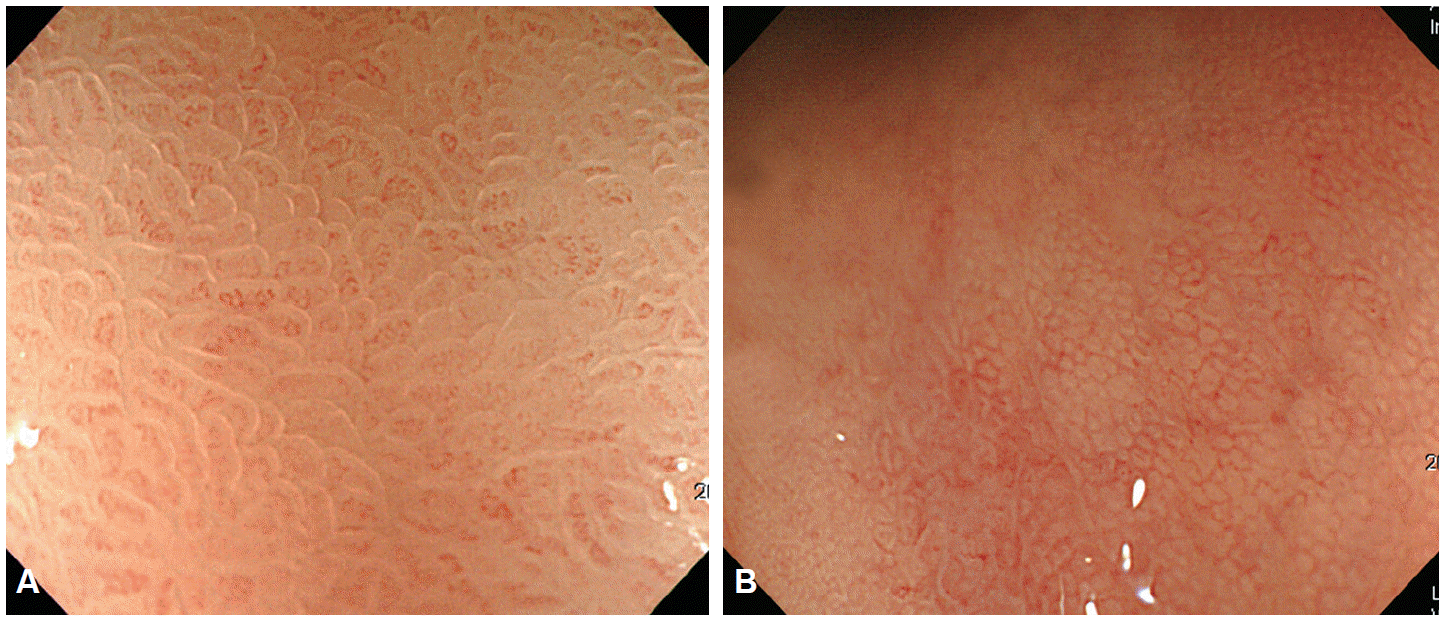
Fig. 3.
Esophageal squamous cell carcinoma. (A) A depressed lesion with irregular nodularity and redness is noted at the mid esophagus. (B) With iodine staining, it is shown as an iodine-void area with a well-defined boundary.
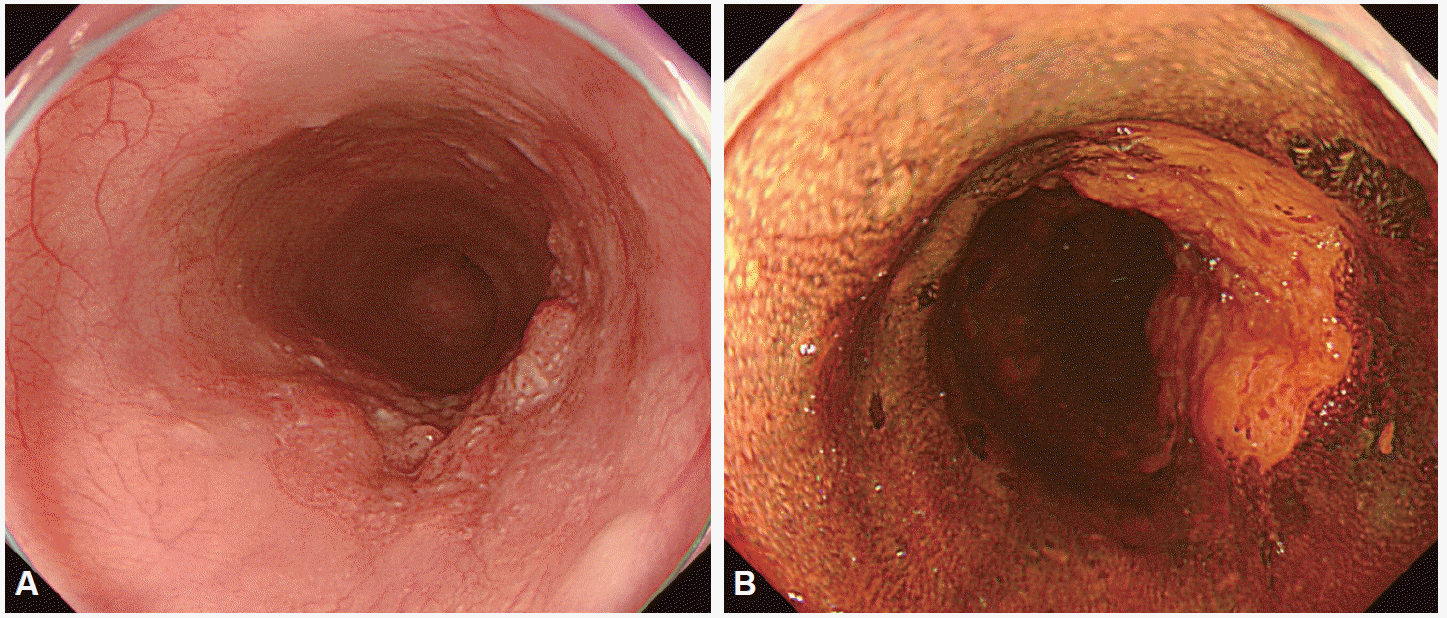
Fig. 4.
Early gastric cancer. (A) Indistinct margined gastric cancer due to marked atrophy and intestinal metaplasia around the lesions are often observed. (B) Indigo carmine chromoendoscopy delineated the lesion more clearly.
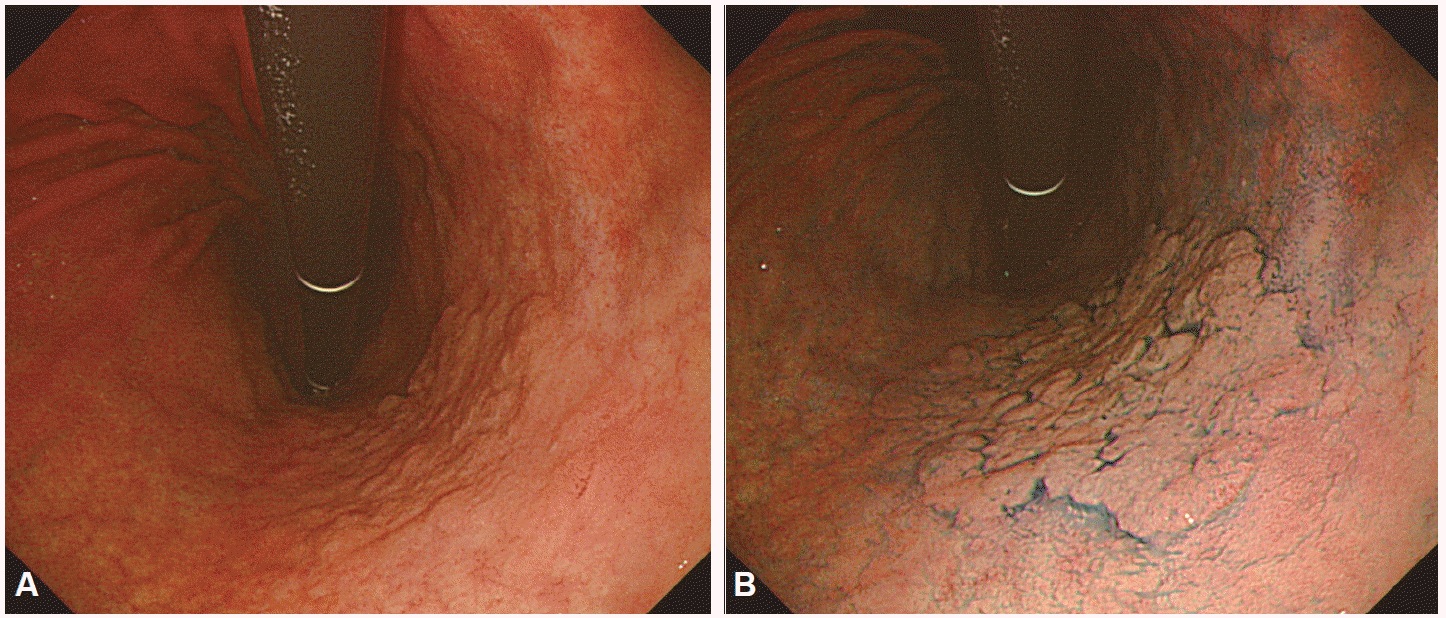
Fig. 5.
Esophageal squamous cell carcinoma. (A) A depressed lesion with irregular nodularity and redness is noted at the lower esophagus. (B) In narrow-band imaging mode, is seen as dark brown lesion due to its copious vascularity.
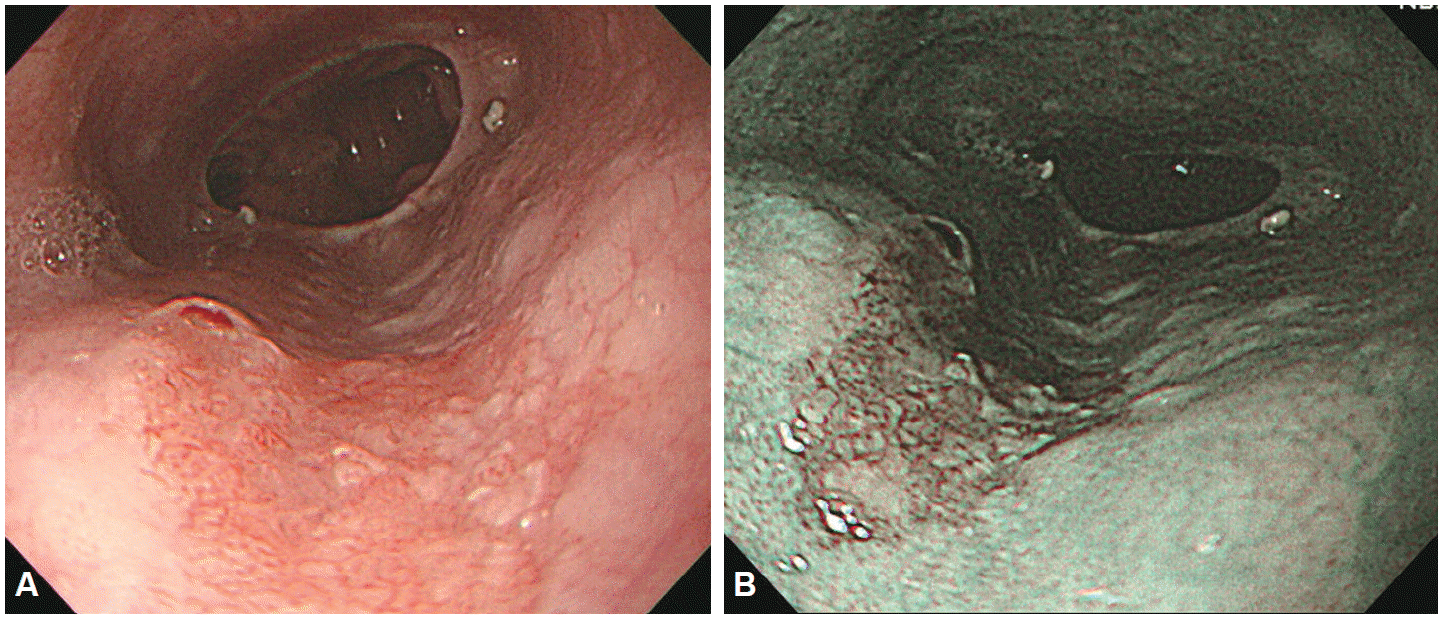
Fig. 6.
Early gastric cancer. (A) About 0.6-cm sized slightly depressed lesion was noted at the lesser curvature side of mid body in white light imaging. (B) Well delineated margin, loss of pit, and irregular microvessels were noted in near focus.
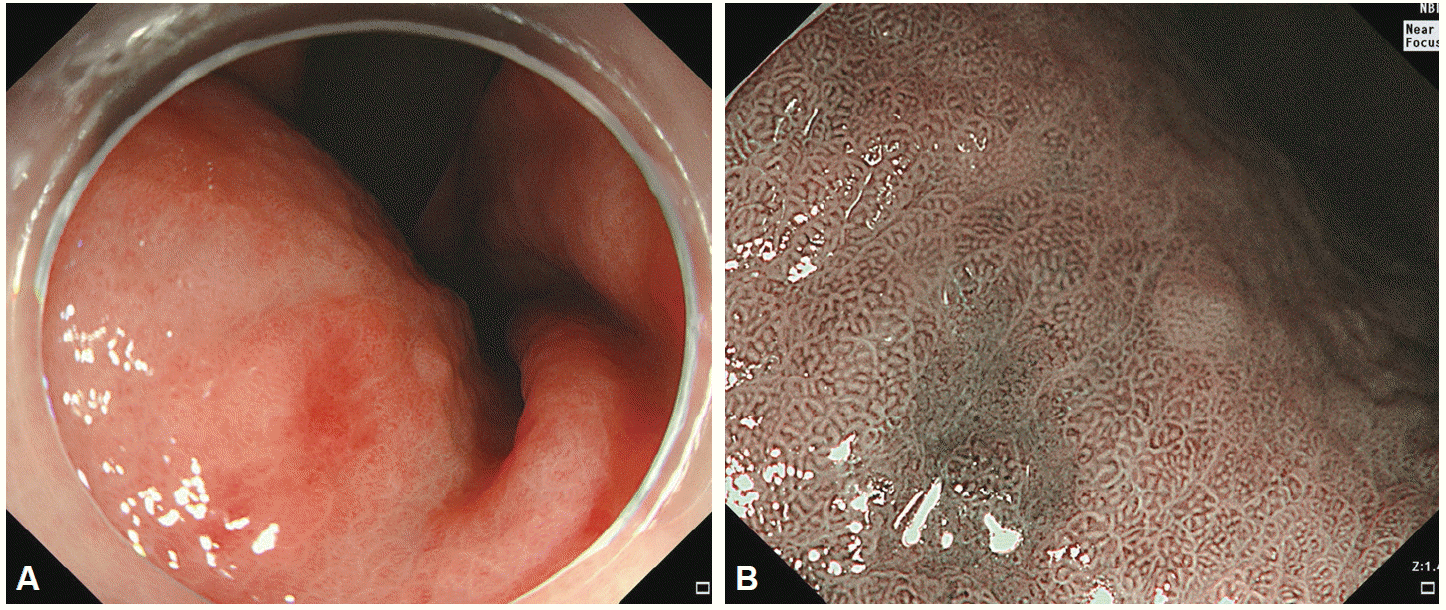
Fig. 7.
i-SCAN image of depressed typed early gastric cancer. (A) Small depressed lesion is noted at antrum. (B) i-SCAN TE-g makes it more clear delineation of the tumor.
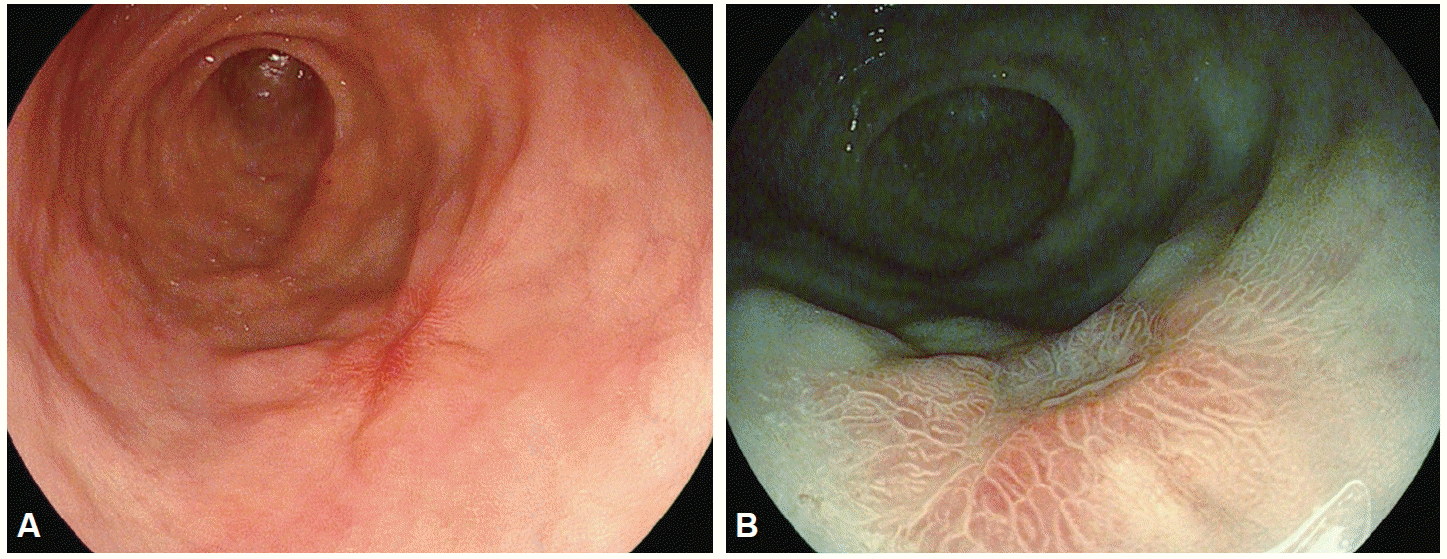
Fig. 8.
Reflux esophagitis. (A) Gastroesophageal junction and a mucosal break are noted in white light imaging. (B) Flexible spectral imaging color enhancement imaging enhanced the visualization of mucosal structure and vessels.
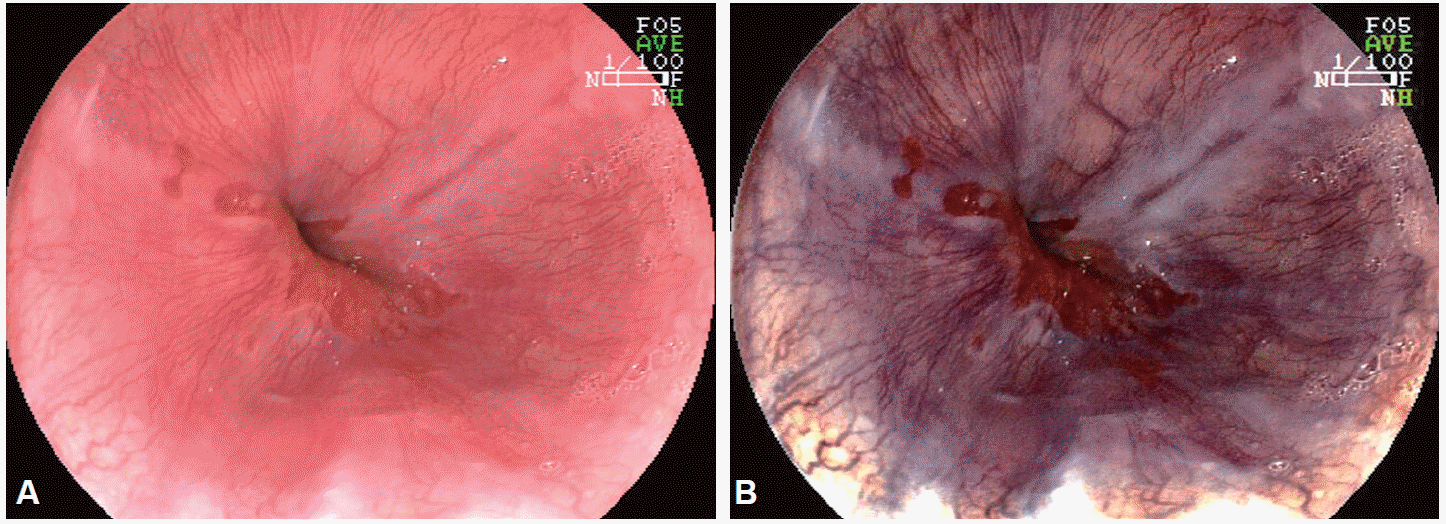
Fig. 9.
Early gastric cancer. (A) A depressed lesion was noted at the antrum. (B) Magnifying narrow-band imaging demonstrated demarcation line, loss of fine mucosal structure, and presence of an irregular microvascular pattern. The lesion was suspected to mucosal well differentiated gastric adenocarcinoma. Endoscopic
submucosal dissection was performed.
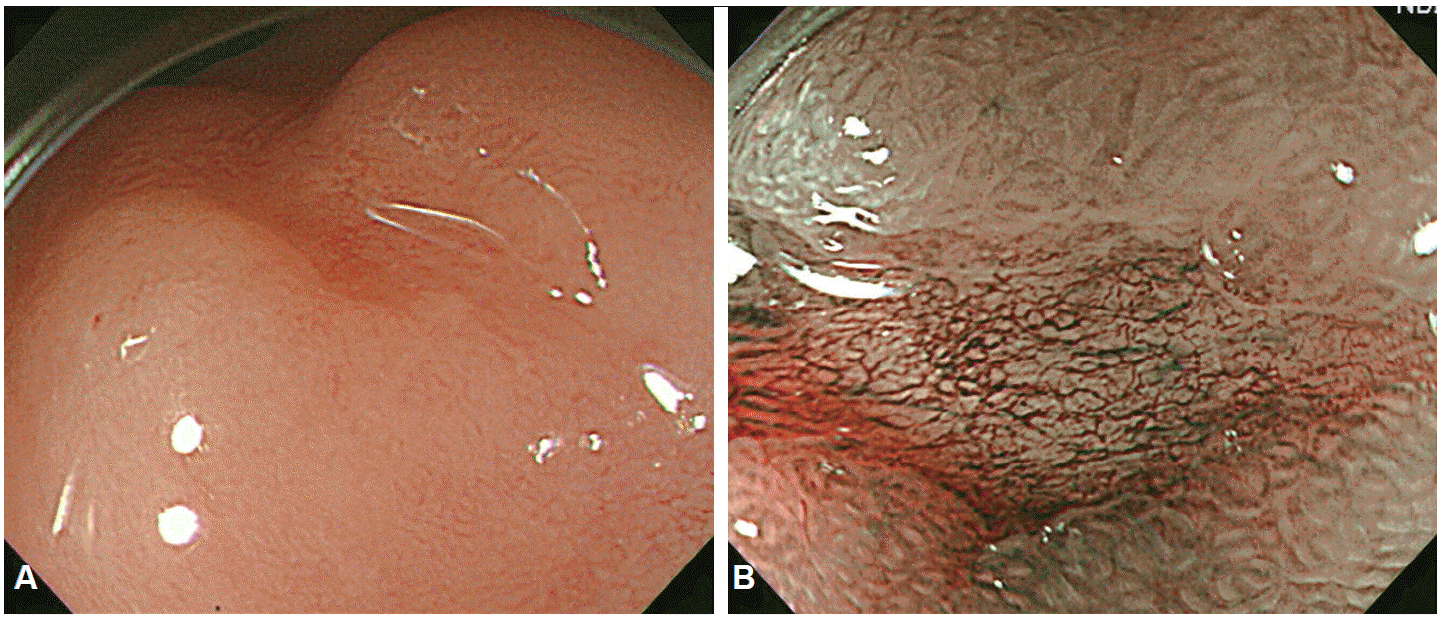
Fig. 10.
Early gastric cancer. (A) Ill defined, pale mucosal lesion with central nodule is noted on the antrum. (B) Magnifying narrow-band imaging findings of that pale mucosa showed loss of the regular microvascular pattern and corkscrew pattern. The lesion was suspected to poorly differentiated gastric adenocarcinoma. Gastrectomy with lymph node dissection was performed.
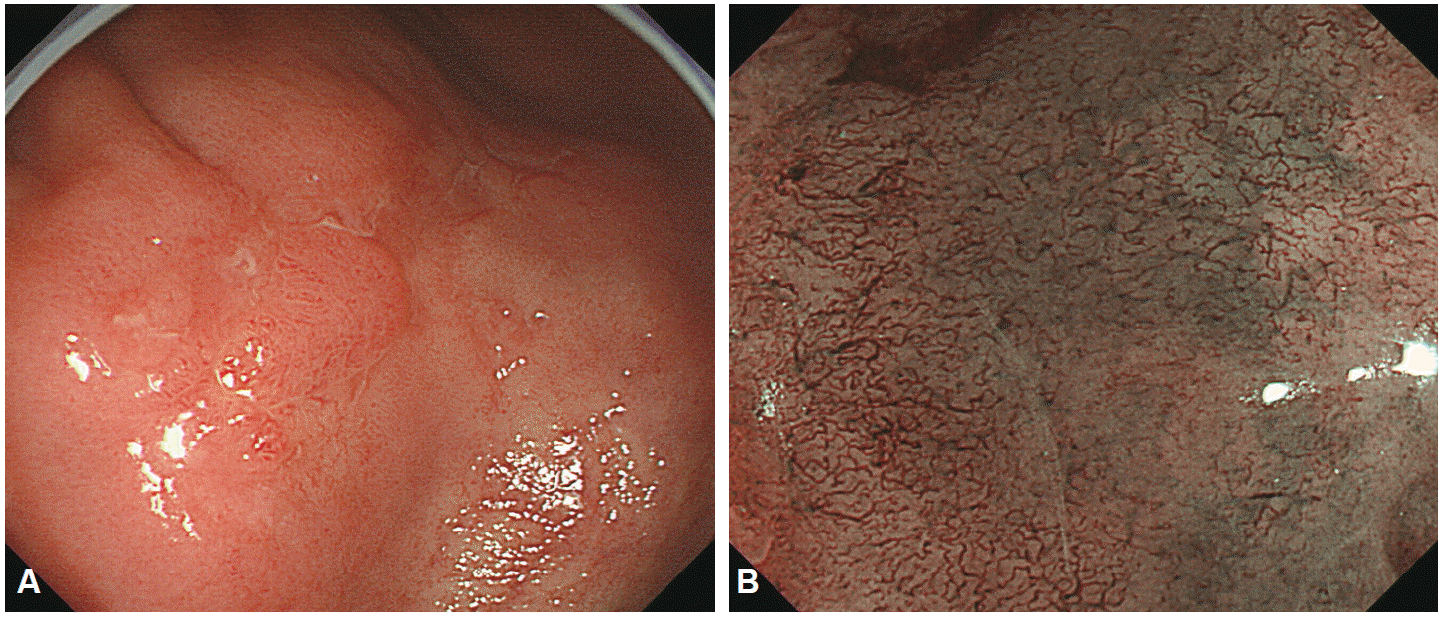