Abstract
Real-time visualization of the molecular signature of cells can be achieved with advanced targeted imaging techniques using molecular probes and fluorescence endoscopy. This molecular optical imaging in gastrointestinal endoscopy is promising for improving the detection of neoplastic lesions, their characterization for patient stratification, and the assessment of their response to molecular targeted therapy and radiotherapy. In inflammatory bowel disease, this method can be used to detect dysplasia in the presence of background inflammation and to visualize inflammatory molecular targets for assessing disease severity and prognosis. Several preclinical and clinical trials have applied this method in endoscopy; however, this field has just started to evolve. Hence, many problems have yet to be solved to enable the clinical application of this novel method.
Gastrointestinal (GI) endoscopy for the diagnosis of neoplasia in the digestive tract has evolved over the last decade. Although white light endoscopy (WLE) has a pivotal role in the early diagnosis of GI lesions, the rate of missed detection of GI lesions has not yet decreased, as reported in many studies.1-3 Recent technological and scientific developments in GI endoscopy have resulted in new imaging devices such as chromoendoscopy and virtual chromoendoscopy techniques, narrow band imaging (NBI), autofluorescence imaging (AFI), confocal laser endomicroscopy (CLE), and Raman spectroscopy, that enhance the detection and characterization of early neoplastic lesions.4 Despite these advances, conventional endoscopy has some diagnostic limitations because it is based on morphological changes.
Interest in molecular imaging in the field of GI endoscopy has increased with the potential for diagnosis and therapy in the early stages of GI neoplasia.5 Molecular imaging has been a pivotal technique for diagnosis and therapy in the field of oncology. It typically includes modalities that enable noninvasive visualization, characterization, and measurement of biological processes at the molecular and cellular levels in humans and other living organisms. Molecular imaging shows great promise for detecting diseases in the early stages and rapidly evaluating tumor responses to chemotherapy and/or radiotherapy. Molecular imaging technology in GI endoscopy has been rapidly undergoing a major paradigm shift. This review discusses advances in endoscopic molecular imaging and endoscopic devices for imaging intestinal diseases.
Molecular imaging in the field of GI endoscopy aims to evaluate cellular perturbations rather than morphological changes and/or discolorations. Disease-specific biomarkers are used as molecular probes in these approaches. It is important to consider several aspects for appropriately selecting exogenous probes, such as target specificity, rapid binding kinetics, deep tissue penetration, low immunogenicity, and the ease at which labeling with fluorescent agents can be performed. Recent molecular imaging studies have used fluorescence-labeled exogenous molecular probes such as antibodies, aptamers, peptides, affibodies, nanoparticles, and activatable probes.5
For molecular imaging in the GI field, imaging devices should be able to distinguish molecular changes in the early stages of GI neoplasia and detect the molecular probes at a concentration that binds to the region of interest in a minimally invasive manner. The proper choice of device for endoscopic molecular imaging focuses on the molecular signature of cells. For the detection of cellular details during GI endoscopy, the use of highly sensitive wide-field endoscopes is mandatory. Recently, customized or experimental endoscopes for the detection of GI lesions, such as AFI, fluorescence endomicroscopy, optical coherence tomography, and CLE have been developed for endoscopic molecular imaging.4
AFI visualizes lesions, including neoplasms, based on the differences in fluorescence emission among various tissue types after excitation by a short-wavelength light source. In initial AFI, the tissue is sequentially illuminated with blue (395 to 475 nm), green (540 to 560 nm), and red (600 to 620 nm) spectra to excite AFI and green reflectance imaging.6 The sequential images of AFI and green reflectance are integrated into a pseudo-colored image. AFI can be used for cancer screening tests without the administration of fluorescence probes. Advances in AFI systems were recently achieved with the development of trimodal imaging video endoscopes that were combined with high-definition white light imaging and NBI.7 AFI has been used to study Barrett's esophagus, gastric neoplasia, colon polyps, and colitis-associated neoplasia.6 Although endoscopic trimodal imaging overcomes the problems of prototype AFI, the current technique lacks sufficient specificity for application in larger clinical trials.7
For distinguishing neoplastic from nonneoplastic tissue using endoscopic imaging, direct biopsies are usually performed to obtain a histological diagnosis. Although histological diagnosis is highly accurate, it has a few limitations, including diagnostic delay, increased costs in pathology procedures, and lower and specificity. In addition, the presence of ulcers can frequently alter the mucosal structure and give false negative/positive histological results. CLE shows great promise as a replacement for biopsies and histological diagnosis, enabling the next therapeutic steps in clinical practice without diagnostic delay.8-10
Two devices are currently registered in clinical trials of CLE for molecular imaging.11-13 The two CLE systems are also accessible for laboratory and/or animal use in translational studies. In endoscope-integrated CLE, a scanner is equipped with the distal tip of a flexible WLE for dual imaging in clinical use, an excitation wavelength via a single optical fiber, and an emission filter.14 The device offers a high spatial resolution of 0.7 µm, imaging depth from the surface of 250 µm, and serial optical sections with a high resolution of 1,024×1,024 pixels using a single optical fiber functioning as both the illumination and detection pinholes. However, the drawback of this device is its lower temporal resolution of approximately 1 frame per second. The other system is probe-based CLE, which provides faster image acquisition of 12 frames per seccond.15 This confocal probe is available with diameters compatible with the working channel of most endoscopes used clinically in GI endoscopy. However, the spatial resolution is lower, and the field of view is smaller. In CLE systems, cells can be visualized based on their molecular signature using fluorescence-labeled exogenous molecular probes. Clinical trials have been performed using these systems; however, most of these used fluorescein for fluorescent staining in morphological imaging.16
As described in the Introduction, the adenoma missed detection rate remains at approximately 22% (range, 15 to 32),2 and flat and depressed lesions are frequently overlooked. Moreover, the specificity of white light imaging for distinguishing neoplastic from nonneoplastic lesions is poor and depends on visible mucosal changes. The histological analysis of small and possibly less relevant lesions significantly increases workload and cost.17 Therefore, benign lesions are often removed, increasing both cost and risk.3
Imaging devices and fluorescent probes should be chosen according to the molecular imaging goal. For lesions to be detected at a high frequency, high sensitivity is mandatory. Therefore, wide-field endoscopes and high-sensitivity probes are needed. However, trials aimed at characterizing lesions might instead opt for high specificity by the resolving cellular details.18 In clinical practice, this technique may improve the detection rate of missed polyps, flat lesions, and residual neoplasia after endoscopic mucosal resection.8-10
Tumor-specific activation of molecular beacons is desired. In a study with Apcmin mice, a cathepsin-sensing near-infrared probe was used for fiberoptic endoscopic detection of colorectal adenomas,19 demonstrating that tumor-specific dequenching of smart probes can be used for endoscopic imaging.
Fluorescence probes for molecular imaging can be applied topically or systemically during GI endoscopy. For safety, topical administration of probes is preferred over intravenous injection. Topical administration is only possible when the target is directly accessible and the region of interest is limited. Injection into a vein is used for systemic administration when the probes cannot pass through the healthy epithelial barrier.
In vivo molecular imaging in the field of GI endoscopy has been used in various preclinical and clinical trials. For the diagnosis of high-grade dysplasia in Barrett esophagus, Li and Wang,20 one of the leading groups in the field of endoscopic molecular imaging, performed optical imaging after topical administration of fluorescence-labeled peptides. To uncover small peptides with a strong affinity for high-grade dysplasia in Barrett esophagus, a phage display technique was employed. For example, this group identified a seven-residue peptide, ASYNYDA, which specifically binds to high-grade dysplasia in Barrett esophagus using the M13 phage display. The fluorescein-labeled ASYNYDA peptide was topically administered over a region of intestinal metaplasia in the distal esophagus. After a 5-minute incubation period, the unbound peptides were rinsed off. Fluorescent images using a wide-field endoscope sensitive to fluorescence revealed peptide homing to a region of high-grade dysplasia that was histologically confirmed. In a recent study, Wang's group also identified peptides that bind to adenomas in the CPC;Apc mouse model using an in vivo phage display (Fig. 1).21,22 Fluorescence-labeled peptides bound to dysplastic epithelium in the CPC;Apc mice were observed using a wide-field endoscope. Using multispectral peptides, colorful images can be obtained that demonstrate a tumor's molecular profile.23,24
Very recently, Urano's group successfully developed a novel activatable fluorescence probe based on the concept of intramolecular spirocyclization.25 The novel spirocyclic rhodamine-based fluorescence probe for γ-glutamyltranspeptidase, called γ-glutamyl hydroxymethyl rhodamine green (gGlu-HMRG), has been successfully developed, and is upregulated in cancer cells (Fig. 2). In mouse models of peritoneal ovarian cancer, the highly fluorescent product HMRG was produced within 1 minute after topical spraying of gGlu-HMRG onto tissue surfaces with a high signal contrast between the tumor and the background. Use of the gGlu-HMRG probe can aid surgeons in detecting cancerous nodules by enabling accurate biopsy and tumor resection as well as delineating the tumor borders to ensure complete tumor removal.26
Although research is promising, several hurdles must still be addressed before optical molecular imaging is ready for clinical use. With regard to the optical molecular probes, the greatest problem to overcome is the clinical approval of fluorochromes and conjugates that have been used as optical molecular imaging probes in animal studies.
Despite the problems of integrating optical molecular imaging into colorectal cancer (CRC) clinical trials, several groups have advanced targeted optical molecular probe imaging strategies for the clinical translation of optical molecular imaging in the field of GI endoscopy in humans. Smart activatable probes are promising technologies for the optical molecular imaging of CRC. These probes are substrates of enzymes that are upregulated at the targeted mucosal region. Enzymatic cleavage of these probes leads to increased fluorescence intensity, which is inhibited by fluorescence energy transfer in the native state.27,28 Yoon et al.29,30 performed near-infrared fluorescence (NIRF) imaging using an matrix metalloproteinase-activatable probe to detect colon tumors at the early disease stage (Fig. 3). They observed diffuse inflammation within the tumors at the early stage of the azoxymethane/dextran sulfate sodium model. NIRF signal intensities were strongly correlated with each tumor stage from adenoma to adenocarcinoma. NIRF imaging also distinguished tumors from inflamed mucosa. This strategy allowed for the earlier assessment of the effects of therapy prior to the occurrence of morphological alterations in the underlying disease. NIRF imaging combined with a polymeric nanoparticle-based probe allows for enhanced tumor detection and estimates the malignant potential of the lesion. Thus, this approach could translate to improved accuracy of endoscopic diagnosis and lesion characterization without the need for invasive procedures such as biopsies.
CRC is a serious potential complication of inflammatory bowel disease (IBD), and the incidence of cancer in IBD is increasing in Korea.31 Regular surveillance colonoscopy is currently recommended for the early detection of dysplasia.32 Traditional surveillance recommendations include interval random biopsy throughout the colon because patients with IBD have a propensity for developing flat and subtle neoplasms that may evade detection and rapidly progress to advanced CRC.33,34 However, random biopsies are difficult to perform, and target biopsy is impossible because underlying inflammatory lesions are irregular. Previously, most dysplasia detected microscopically using random biopsies was thought to be invisible by white light colonoscopy.33,34 Recently, Gounaris et al.33 reported that cathepsin substrate-based probe imaging correctly identifies dysplastic foci within chronically inflamed colons. Therefore, they suggested that combined WLE and NIRF endoscopy has unique advantages that may increase sensitivity and specificity of surveillance colonoscopy in patients with colitis-associated cancer (CAC).33 In the future, molecular imaging might help in the early detection of CAC and dysplasia.35,36
Defects in intestinal barrier function are associated with IBD.37 Applying the concept that increased intestinal permeability is also a prognostic indicator of relapse, Kiesslich et al.37 reported that cell shedding and barrier loss detected by CLE predicts IBD relapse and has the potential to become a diagnostic tool for its management. In the future, molecular imaging targeting many inflammatory cells or mediators may be used to monitor disease severity and predict disease prognosis.
Molecular imaging can be applied to targeted therapies in oncology. In CRC, autonomous growth is considered a multi-step process that includes the upregulation of growth factors and their receptors such as epidermal growth factor receptor (EGFR). Cetuximab, a chimeric monoclonal antibody against the extracellular domain of EGFR, is a component of multimodal chemotherapy for metastatic CRC.38 Goetz et al.39 recently demonstrated that molecular imaging using fluorescence imaging could predict the cetuximab response. These results indicate that in the future, molecular imaging may be used for predicting patient responses to targeted therapy for CRC.
The development of apoptosis imaging agents is another translational research area in optical molecular imaging in the field of GI endoscopy. Molecular imaging of apoptosis is a useful tool for evaluating the response to chemotherapy and radiotherapy. During apoptosis, phosphatidylserine (PS) is exposed on the apoptotic cell surface. PS is a phospholipid that is normally expressed on the inner leaflet of the bilamellar cell membrane and is invariably translocated to the outer surface as an early event in apoptosis. Therefore, apoptosis imaging probes are based on PS recognition.
Using an M13 phage display, Thapa and coworkers40 identified a nine-residue phosphatidylserine-binding peptide (PSP), CLSYYPSYC. The fluorescein-labeled CLSYYPSYC peptide specifically binds to apoptotic cells, and optical imaging after systemic administration of this peptide to tumor-bearing nude mice (H460 cell xenograft model) treated with a single dose of an anticancer drug (camptothecin) revealed peptide homing to the tumor. This peptide-based specific probe has several advantages over proteins and antibodies, such as superior stability, nonimmunogenicity, low cost, easy labeling, and rapid clearance and tissue penetration. Moreover, the optical probe Cy5.5, which emits in the near-infrared range rather than the visible range, facilitates the detection of signals in vitro, ex vivo, and in vivo. Therefore, apoptotic imaging using PSP-Cy5.5 may be useful for clinical purposes.
One major challenge in radiobiology is predicting tumor radioresistance and determining normal tissue radiosensitivity for providing patients with personalized treatment. Optical imaging may more efficiently evaluate the radiation treatment response because it can be used to detect local signals from an irradiated lesion. Moreover, optical molecular imaging is more versatile than positron emission tomography molecular imaging because of its ability to intervene in the GI tract while the mucosal lesion is being identified. This is enabled because fluorescence imaging devices are coupled to the endoscopes and can provide real-time in vivo image information; thus, optical imaging can be more easily used for molecularly targeted procedure guidance.41 Optical imaging coupled with endoscopy is a good imaging modality choice for assessing the response to radiotherapy of intraluminal neoplasms, including rectal, cervical, bladder, or head and neck cancer. Using optical imaging coupled with endoscopy, the response to radiotherapy can be predicted promptly, facilitating more rapid therapeutic decisions. In this regard, the apoptosis optical imaging with fluorescence dye approach presented in the previously mentioned study may be applicable to functional endoscopy in the future.
Sumiyama et al.42 reported that they could examine enteric neuronal networks using endomicroscopy after colonic polyp removal. Although their observation suggests the future possibility of enteric neural network imaging using endoscopy, it is not clinically applicable at this time because current techniques, including CLE and AFI, have observation depth limitations.
Endoscopy is a crucial instrument for gastroenterologists and is continuously evolving with advances in technology. Molecular imaging in endoscopy is an attractive method that might be used to visualize the molecular profiles and morphologic characteristics of GI lesions. Endoscopy can be used for early detection of GI tumors, especially flat and small lesions, dysplasia in IBD, therapy monitoring, and prediction of therapeutic responsiveness. In addition, with the development of novel optical imaging devices, further imaging of GI mucosal and submucosal structures might be possible in the future. However, at this point, numerous hurdles, including safety issues, have to be overcome. Another limitation of molecular imaging in endoscopy is that most molecular endoscopic imaging methods are useful for only one molecular factor. In an attempt to overcome this limitation, methodology that allows for multispectral endoscopic imaging has been introduced. This addresses the heterogeneity of cancer and allows for detection of the disease with high sensitivity and specificity. Considerable work will be required to integrate molecular imaging into the clinical diagnostic algorithm. Integration of the chemical and mechanical aspects of research into the clinical setting is crucial to allow application of this novel method to clinical endoscopy.
Acknowledgments
This research was supported by the Bio & Medical Technology Development Program of the National Research Foundation funded by the Korean government (MEST) (no. 2011-0019632) and the Asan Institute for Life Sciences (no. 2013-261 and 2013-559).
References
1. Yalamarthi S, Witherspoon P, McCole D, Auld CD. Missed diagnoses in patients with upper gastrointestinal cancers. Endoscopy. 2004; 36:874–879. PMID: 15452783.


2. van Rijn JC, Reitsma JB, Stoker J, Bossuyt PM, van Deventer SJ, Dekker E. Polyp miss rate determined by tandem colonoscopy: a systematic review. Am J Gastroenterol. 2006; 101:343–350. PMID: 16454841.


3. Matloff JL, Abidi W, Richards-Kortum R, Sauk J, Anandasabapathy S. High-resolution and optical molecular imaging for the early detection of colonic neoplasia. Gastrointest Endosc. 2011; 73:1263–1273. PMID: 21628019.


4. Dacosta RS, Wilson BC, Marcon NE. New optical technologies for earlier endoscopic diagnosis of premalignant gastrointestinal lesions. J Gastroenterol Hepatol. 2002; 17(Suppl):S85–S104. PMID: 12000596.


5. Goetz M, Wang TD. Molecular imaging in gastrointestinal endoscopy. Gastroenterology. 2010; 138:828–833. PMID: 20096697.


6. Uedo N, Iishi H, Tatsuta M, et al. A novel videoendoscopy system by using autofluorescence and reflectance imaging for diagnosis of esophagogastric cancers. Gastrointest Endosc. 2005; 62:521–528. PMID: 16185965.


7. Curvers WL, van Vilsteren FG, Baak LC, et al. Endoscopic trimodal imaging versus standard video endoscopy for detection of early Barrett's neoplasia: a multicenter, randomized, crossover study in general practice. Gastrointest Endosc. 2011; 73:195–203. PMID: 21168835.


8. Shahid MW, Buchner AM, Coron E, et al. Diagnostic accuracy of probe-based confocal laser endomicroscopy in detecting residual colorectal neoplasia after EMR: a prospective study. Gastrointest Endosc. 2012; 75:525–533. PMID: 22051243.


9. Shahid MW, Buchner AM, Heckman MG, et al. Diagnostic accuracy of probe-based confocal laser endomicroscopy and narrow band imaging for small colorectal polyps: a feasibility study. Am J Gastroenterol. 2012; 107:231–239. PMID: 22068663.


10. Shahid MW, Buchner AM, Raimondo M, Woodward TA, Krishna M, Wallace MB. Accuracy of real-time vs. blinded offline diagnosis of neoplastic colorectal polyps using probe-based confocal laser endomicroscopy: a pilot study. Endoscopy. 2012; 44:343–348. PMID: 22382851.


11. Goetz M, Malek NP, Kiesslich R. Microscopic imaging in endoscopy: endomicroscopy and endocytoscopy. Nat Rev Gastroenterol Hepatol. 2013; 7. 30. Epub. DOI: 10.1038/nrgastro.2013.134.


12. Goetz M, Watson A, Kiesslich R. Confocal laser endomicroscopy in gastrointestinal diseases. J Biophotonics. 2011; 4:498–508. PMID: 21567975.


13. Wallace MB, Fockens P. Probe-based confocal laser endomicroscopy. Gastroenterology. 2009; 136:1509–1513. PMID: 19328799.


14. Kiesslich R, Burg J, Vieth M, et al. Confocal laser endoscopy for diagnosing intraepithelial neoplasias and colorectal cancer in vivo. Gastroenterology. 2004; 127:706–713. PMID: 15362025.


15. Meining A, Saur D, Bajbouj M, et al. In vivo histopathology for detection of gastrointestinal neoplasia with a portable, confocal miniprobe: an examiner blinded analysis. Clin Gastroenterol Hepatol. 2007; 5:1261–1267. PMID: 17689297.


16. Wallace MB, Meining A, Canto MI, et al. The safety of intravenous fluorescein for confocal laser endomicroscopy in the gastrointestinal tract. Aliment Pharmacol Ther. 2010; 31:548–552. PMID: 20002025.


17. Schachschal G, Mayr M, Treszl A, et al. Endoscopic versus histological characterisation of polyps during screening colonoscopy. Gut. 2013; 6. 28. Epub. DOI: 10.1136/gutjnl-2013-304562.


18. Atreya R, Goetz M. Molecular imaging in gastroenterology. Nat Rev Gastroenterol Hepatol. Epub 2013 Jul 16. DOI: 10.1038/nrgastro.2013.125.


19. Marten K, Bremer C, Khazaie K, et al. Detection of dysplastic intestinal adenomas using enzyme-sensing molecular beacons in mice. Gastroenterology. 2002; 122:406–414. PMID: 11832455.


20. Li M, Wang TD. Targeted endoscopic imaging. Gastrointest Endosc Clin N Am. 2009; 19:283–298. PMID: 19423025.


21. Miller SJ, Joshi BP, Feng Y, Gaustad A, Fearon ER, Wang TD. In vivo fluorescence-based endoscopic detection of colon dysplasia in the mouse using a novel peptide probe. PLoS One. 2011; 6:e17384. PMID: 21408169.


22. Joshi BP, Liu Z, Elahi SF, Appelman HD, Wang TD. Near-infrared-labeled peptide multimer functions as phage mimic for high affinity, specific targeting of colonic adenomas in vivo (with videos). Gastrointest Endosc. 2012; 76:1197–1206. PMID: 23022051.


23. Joshi BP, Miller SJ, Lee CM, Seibel EJ, Wang TD. Multispectral endoscopic imaging of colorectal dysplasia in vivo. Gastroenterology. 2012; 143:1435–1437. PMID: 23041325.


24. Miller SJ, Lee CM, Joshi BP, Gaustad A, Seibel EJ, Wang TD. Targeted detection of murine colonic dysplasia in vivo with flexible multispectral scanning fiber endoscopy. J Biomed Opt. 2012; 17:021103. PMID: 22463021.


25. Urano Y. Novel live imaging techniques of cellular functions and in vivo tumors based on precise design of small molecule-based 'activatable' fluorescence probes. Curr Opin Chem Biol. 2012; 16:602–608. PMID: 23149093.


26. Mitsunaga M, Kosaka N, Choyke PL, et al. Fluorescence endoscopic detection of murine colitis-associated colon cancer by topically applied enzymatically rapid-activatable probe. Gut. 2013; 62:1179–1186. PMID: 22698650.


27. Tung CH, Mahmood U, Bredow S, Weissleder R. In vivo imaging of proteolytic enzyme activity using a novel molecular reporter. Cancer Res. 2000; 60:4953–4958. PMID: 10987312.
28. Bremer C, Bredow S, Mahmood U, Weissleder R, Tung CH. Optical imaging of matrix metalloproteinase-2 activity in tumors: feasibility study in a mouse model. Radiology. 2001; 221:523–529. PMID: 11687699.


29. Yoon SM, Myung SJ, Ye BD, et al. Near-infrared fluorescence imaging using a protease-specific probe for the detection of colon tumors. Gut Liver. 2010; 4:488–497. PMID: 21253297.


30. Yoon SM, Myung SJ, Kim IW, et al. Application of near-infrared fluorescence imaging using a polymeric nanoparticle-based probe for the diagnosis and therapeutic monitoring of colon cancer. Dig Dis Sci. 2011; 56:3005–3013. PMID: 21465144.


31. Kim BJ, Yang SK, Kim JS, et al. Trends of ulcerative colitis-associated colorectal cancer in Korea: a KASID study. J Gastroenterol Hepatol. 2009; 24:667–671. PMID: 19378391.


32. Murthy SK, Kiesslich R. Evolving endoscopic strategies for detection and treatment of neoplastic lesions in inflammatory bowel disease. Gastrointest Endosc. 2013; 77:351–359. PMID: 23317581.


33. Gounaris E, Martin J, Ishihara Y, et al. Fluorescence endoscopy of cathepsin activity discriminates dysplasia from colitis. Inflamm Bowel Dis. 2013; 19:1339–1345. PMID: 23591598.


34. Awais D, Siegel CA, Higgins PD. Modelling dysplasia detection in ulcerative colitis: clinical implications of surveillance intensity. Gut. 2009; 58:1498–1503. PMID: 19651634.


35. Neumann H, Kiesslich R. Endomicroscopy and endocytoscopy in IBD. Gastrointest Endosc Clin N Am. 2013; 23:695–705. PMID: 23735111.


36. Neumann H, Vieth M, Günther C, et al. Virtual chromoendoscopy for prediction of severity and disease extent in patients with inflammatory bowel disease: a randomized controlled study. Inflamm Bowel Dis. 2013; 19:1935–1942. PMID: 23839228.
37. Kiesslich R, Duckworth CA, Moussata D, et al. Local barrier dysfunction identified by confocal laser endomicroscopy predicts relapse in inflammatory bowel disease. Gut. 2012; 61:1146–1153. PMID: 22115910.


38. Van Cutsem E, Köhne CH, Láng I, et al. Cetuximab plus irinotecan, fluorouracil, and leucovorin as first-line treatment for metastatic colorectal cancer: updated analysis of overall survival according to tumor KRAS and BRAF mutation status. J Clin Oncol. 2011; 29:2011–2019. PMID: 21502544.


39. Goetz M, Hoetker MS, Diken M, Galle PR, Kiesslich R. In vivo molecular imaging with cetuximab, an anti-EGFR antibody, for prediction of response in xenograft models of human colorectal cancer. Endoscopy. 2013; 45:469–477. PMID: 23580409.


40. Thapa N, Kim S, So IS, et al. Discovery of a phosphatidylserine-recognizing peptide and its utility in molecular imaging of tumour apoptosis. J Cell Mol Med. 2008; 12(5A):1649–1660. PMID: 18363834.


41. Mahmood U. Optical molecular imaging approaches in colorectal cancer. Gastroenterology. 2010; 138:419–422. PMID: 20026448.


42. Sumiyama K, Kiesslich R, Ohya TR, Goetz M, Tajiri H. In vivo imaging of enteric neuronal networks in humans using confocal laser endomicroscopy. Gastroenterology. 2012; 143:1152–1153. PMID: 22964351.


Fig. 1
Images under wide-field endoscopy videos after topical administration of fluorescence-labeled peptides. The left and right columns represent frames from white light and fluorescence, respectively. (A) The fluorescent-labeled target peptide FITC-Ahx-QPIHPNNM shows positive binding to multiple adenomas and (B) a single adenoma. (C) The control peptide shows minimal binding. (D) The target peptide also shows minimal binding to the lumen of the control mouse lacking Cre recombinase transgene and (E) the hyperplastic epithelium in a mutant K-ras mouse model. White arrows identify adenomas. Adapted from Miller et al. PLoS One 2011;6:e17384.21
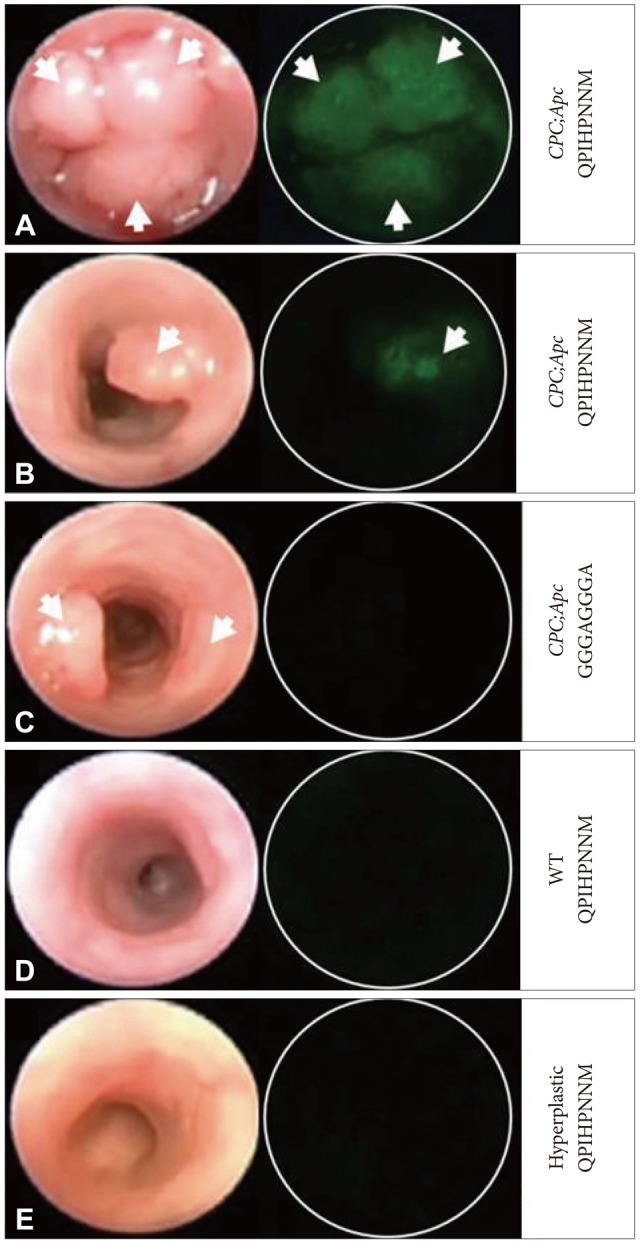
Fig. 2
γ-Glutamyl hydroxymethyl rhodamine green (gGlu-HMRG) fluorescence-guided tissue biopsy confirmed the presence of neoplastic lesions histologically. (A) gGlu-HMRG fluorescence-guided tissue biopsy under fluorescence colonoscopy. (B) Histology obtained by tissue biopsy. (C) Chronic microscopic colitis did not show detectable gGlu-HMRG fluorescence. Adapted from Mitsunaga et al. Gut 2013;62:1179-1186, with permission from BMJ Publishing Group Ltd.26 WL, white light image; BL, blue excitation light image; FL, fluorescence image.
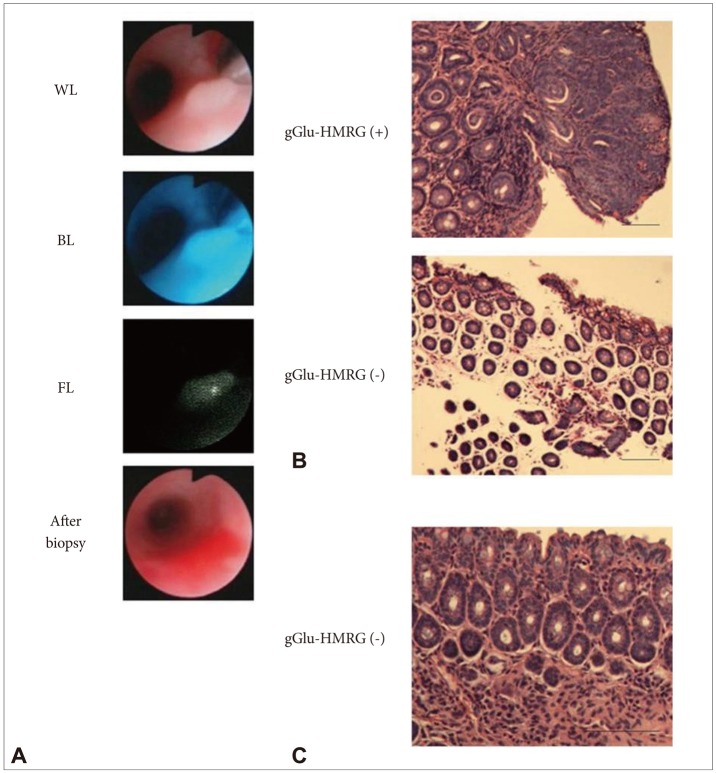
Fig. 3
Histology and near-infrared fluorescence (NIRF) imaging using an matrix metalloproteinase (MMP)-activatable probe in the AOM/DSS model. (A, B) Representative images of colons from BALB/c mice treated with AOM/DSS after injecting an MMP-activatable probe (A) or normal saline (B). (C) Histology and NIRF findings in BALB/c mice treated with AOM/DSS. Adapted from Yoon et al. Gut Liver 2010;4:488-497.29 IHC, immunohistochemistry; NIR, near infrared.
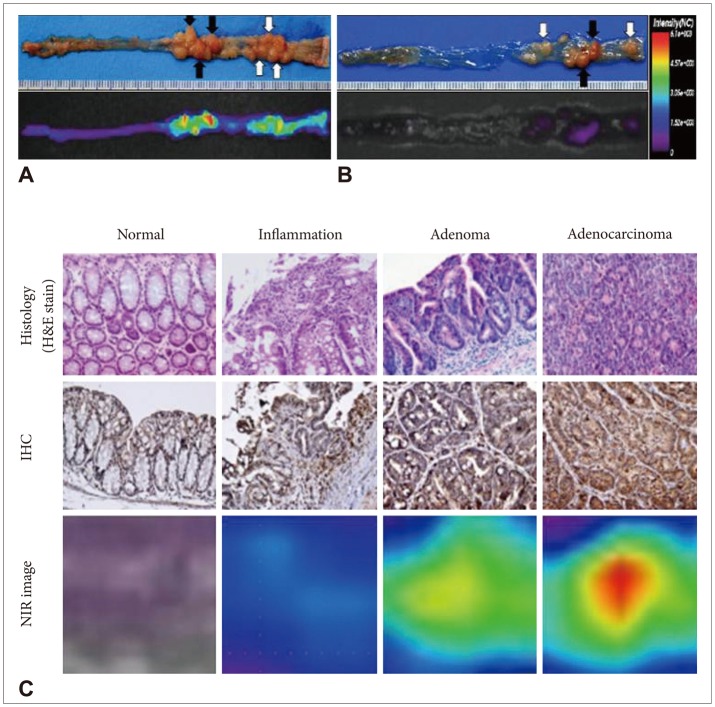