Abstract
The clinical indication for capsule endoscopy has expanded from small bowel evaluation to include esophagus or colon evaluation. Nevertheless, the role of capsule endoscopy in evaluation of the stomach is very limited because of the large volume and surface. However, efforts to develop an active locomotion system for capsule manipulation in detailed gastric evaluation are ongoing, because the technique is non-invasive, convenient, and safe, and requires no sedation. Studies have successfully reported gastric evaluation using a magnetic-controlled capsule endoscopy system. Advances in technology suggest that capsule endoscopy will have a major role not only in the evaluation of gastric disorders but also in the pathologic diagnosis, intervention, and treatment of any gastrointestinal tract disorder.
Capsule endoscopy (CE) was introduced in 2001 for small bowel evaluation. Capsules designed for the esophagus and colon have shown promising results and are used in clinical practice [1]. However, the stomach has a large surface area and volume, and the gastric mucosa is difficult to observe entirely by CE. In clinical practice, it is relatively easy to identify and manage gastric lesions with flexible endoscopy, suggesting the limited role of CE for gastric lesions. This review focuses on the advantages and future direction of gastric CE while addressing current limitations.
The swallowed capsule rapidly passes the proximal part of the stomach and usually remains in the antrum before entering the duodenum. Generally, the distal part of the stomach is easily observed with CE.
Vascular lesions such as gastric antral vascular ectasia are more easily detected with capsules than with conventional upper endoscopy [2,3]. One possible reason is related to air insufflation. During esophagogastroduodenoscopy (EGD), air is insufflated to distend the stomach. In contrast, CE is performed under more physiological conditions. Increased intragastric pressure and flattened rugal folds diminish the blood flow to ectatic vessels. In addition, the close and magnified view of gastric mucosa provided by CE may allow better definition of subtle vascular changes [2,4].
Interestingly, CE has been used to detect a lesion in the dark side of the pylorus, which is difficult to evaluate with conventional gastroscopy [5].
Despite several advantages, CE has limited clinical use in the stomach mainly due to passive movement. In a study, the capsule was moved to each part of the stomach under gravity while changing the examinee’s body position to detect intragastric lesions and normal gastric anatomy [6]. However, the result was not satisfactory and had limited value in the evaluation of gastric lesions.
In addition to restricted movement, CE has other limitations. CE cannot remove bubbles, exudate, and liquid material to obtain better images, cannot perform air insufflation to expand the stomach, and cannot perform diagnostic biopsy or therapeutic interventions [7]. The costs are still high compared to conventional endoscopy. Due to these limitations, CE is not performed for gastric evaluation in clinical practice. However, in 10%–20% of occult gastrointestinal bleeding, CE aided the detection of gastric or colonic lesions missed by EGD or colonoscopy [8,9]. Therefore, when reviewing CE images, attention should be given to any gastric lesions that may have been missed with EGD.
Several studies have attempted to overcome the limitations of CE in gastric evaluation. Because gastric evaluation using spontaneous bowel motion or altered body position has very limited use, many studies have been performed to develop capsules with active locomotion systems [10]. Active locomotion systems mainly use two methods: in one, an internal locomotion system uses an embedded on-board miniaturized actuator (e.g., embedded motorized propeller for locomotion in a liquid environment); the other uses an external locomotion system that relies on magnetic force to control capsule movement [11-13].
In the study of active capsule locomotion systems, magnetic-controlled CE has been used most extensively and has provided some clinical data (Table 1) [11]. In the magnetic-controlled system, capsule movement occurs either with a handheld external magnet [14-17] or with a robot-guided magnet through a computer workstation (Figs. 1-3) [18-27]. Robotic control is more precise and reliable than manual control [21,25]; however, both methods have yet to achieve performance in detailed gastric evaluation comparable to that of EGD. In recent studies, robot-guided magnetic-controlled CE showed promising results in detecting gastric lesions with accuracy comparable to that of conventional gastroscopy [26,27]. In most clinical trials for gastric evaluation, CE showed accuracy comparable to that of conventional gastroscopy, but some lesions were detected more sensitively with CE. These were small hyperplastic polyps and angiomas [4]. This might be due to the longer examination time or magnified view of CE. However, these finding did not influence the main diagnostic outcomes of conventional endoscopy. The rate of adverse events during magnetic-assisted CE for gastric evaluation was 1%–4% (Table 1). All reported events were without significant sequelae and resolved completely a few hours or days later (e.g., abdominal distension, nausea, vomiting, foreign body sensation, and inability to swallow the capsule) [4,26,27]. Most events seemed to be related to gastric preparation to distend the stomach, which varies slightly among studies but usually involves drinking 400–1,000 mL of water. It is expected that technical advances may facilitate a significant role for magnetic-controlled CE in gastric disease management in the near future.
For internal locomotion, several types of platforms have been developed, including leg-based capsules [28-31], paddling-based technique [32,33], and an earthworm-like mechanism [34,35], but only a propeller-based locomotion system has undergone continuous study, with several published reports of basic mechanical and in vivo animal studies. However, this system is still in the experimental stage, with clinical trials yet to be performed [12,36-38]. The most significant drawback of an internal locomotion system is mechanical complexity requiring large on-board volume and a large power supply. Because of these limitations, magnetic-controlled external locomotion systems have drawn more interest [11].
During CE, the stomach is in a collapsed state and CE cannot expose the entire gastric mucosa. Air insufflation devices have been developed and ex vivo and in vivo animal studies have been conducted. However, no clinical trial has been performed for gastric evaluation [39,40]. In several clinical trials of magnetic-controlled CE for gastric evaluation, orally instilled air-generating powder has been used to achieve adequate gastric distension, especially for the proximal stomach, but resulted in suboptimal visualization [16,21,27,41].
CE for gastric evaluation has limitations similar to that of CE for other gastrointestinal regions, but also has specific considerations associated with gastric anatomy. The stomach is a spacious organ with an irregular shape requiring a sophisticated active locomotion platform, compared to that for other parts of the gastrointestinal tract, explaining why most research on gastric evaluation using CE has focused on active locomotion systems. Magnetic-assisted CE has been studied most extensively. However, the distance between the ventral skin surface and gastric fundus is longer than the distance between ventral skin and antrum, making evaluation of the fundic area difficult [48]. In addition, the collapsed state of the fundus during CE makes evaluation more difficult. Thus, further studies are required to identify the most effective and safe magnet strength needed to manipulate CE in the proximal gastric area as well as to identify a satisfactory method of gastric distension [41].
With rapid technological advances, current limitations will be overcome and costs will decrease. Because of the innate advantages of CE, which is non-invasive, safe, and patient-friendly, current limitations will be overcome to make CE a screening tool superior to that of conventional gastroscopy. These advantages have especially significant clinical implications in countries with national gastric cancer screening programs, as in Korea. If artificial intelligence (AI) is combined with capsule endoscopic image interpretation, automated gastric evaluation without involvement of medical professionals will be possible. Patients can easily perform gastric self-evaluation. Only those with suspected pathology on CE imaging with AI-assisted interpretation, using settings to detect lesions with high sensitivity, will be examined by a physician and undergo conventional gastric endoscopy. For this scenario to be realized, current obstacles that must be overcome are air insufflation, sophisticated active locomotion, correct localization of CE in the body, and sensitive AI-assisted interpretation of CE imaging.
The clinical significance of CE in the evaluation of gastric pathology is very limited. However, due to patient-friendly, non-invasive, and safe features requiring no sedation, trials to develop more sophisticated CE control systems are continuing. In the near future, technical improvements including integration with AI may facilitate the application of CE as an important medical device not only in the small bowel but also for gastric lesion evaluation and management. We dare to predict that the entire gastrointestinal tract can be evaluated with CE (pan-endoscopy) in the future.
REFERENCES
1. ASGE Technology Committee, Wang A, Banerjee S, et al. Wireless capsule endoscopy. Gastrointest Endosc. 2013; 78:805–815.


2. Sidhu R, Sanders DS, McAlindon ME. Does capsule endoscopy recognise gastric antral vascular ectasia more frequently than conventional endoscopy? J Gastrointestin Liver Dis. 2006; 15:375–377.
3. Alkhormi AM, Memon MY, Alqarawi A. Gastric antral vascular ectasia: a case report and literature review. J Transl Int Med. 2018; 6:47–51.


4. Rey JF, Ogata H, Hosoe N, et al. Blinded nonrandomized comparative study of gastric examination with a magnetically guided capsule endoscope and standard videoendoscope. Gastrointest Endosc. 2012; 75:373–381.


5. Takahashi Y, Fujimori S, Toyoda M, et al. The blind spot of an EGD: capsule endoscopy pinpointed the source of obscure GI bleeding on the dark side of the pylorus. Gastrointest Endosc. 2011; 73:607–608.


6. Jun BY, Lim CH, Lee WH, et al. Detection of neoplastic gastric lesions using capsule endoscopy: pilot study. Gastroenterol Res Pract. 2013; 2013:730261.


7. Song HJ, Shim KN. Current status and future perspectives of capsule endoscopy. Intest Res. 2016; 14:21–29.


8. Peter S, Heuss LT, Beglinger C, Degen L. Capsule endoscopy of the upper gastrointestinal tract -- the need for a second endoscopy. Digestion. 2005; 72:242–247.


9. Delvaux M, Fassler I, Gay G. Clinical usefulness of the endoscopic video capsule as the initial intestinal investigation in patients with obscure digestive bleeding: validation of a diagnostic strategy based on the patient outcome after 12 months. Endoscopy. 2004; 36:1067–1073.


10. Kwack WG, Lim YJ. Current status and research into overcoming limitations of capsule endoscopy. Clin Endosc. 2016; 49:8–15.


11. Slawinski PR, Obstein KL, Valdastri P. Capsule endoscopy of the future: what’s on the horizon? World J Gastroenterol. 2015; 21:10528–10541.


12. De Falco I, Tortora G, Dario P, Menciassi A. An integrated system for wireless capsule endoscopy in a liquid-distended stomach. IEEE Trans Biomed Eng. 2014; 61:794–804.


13. Ciuti G, Caliò R, Camboni D, et al. Frontiers of robotic endoscopic capsules: a review. J Microbio Robot. 2016; 11:1–18.


14. Rahman I, Pioche M, Shim CS, et al. Magnetic-assisted capsule endoscopy in the upper GI tract by using a novel navigation system (with video). Gastrointest Endosc. 2016; 83:889–895. e1.
15. Lien GS, Liu CW, Jiang JA, Chuang CL, Teng MT. Magnetic control system targeted for capsule endoscopic operations in the stomach--design, fabrication, and in vitro and ex vivo evaluations. IEEE Trans Biomed Eng. 2012; 59:2068–2079.
16. Keller J, Fibbe C, Volke F, et al. Inspection of the human stomach using remote-controlled capsule endoscopy: a feasibility study in healthy volunteers (with videos). Gastrointest Endosc. 2011; 73:22–28.


17. Swain P, Toor A, Volke F, et al. Remote magnetic manipulation of a wireless capsule endoscope in the esophagus and stomach of humans (with videos). Gastrointest Endosc. 2010; 71:1290–1293.
18. Qian Y, Wu S, Wang Q, et al. Combination of five body positions can effectively improve the rate of gastric mucosa’s complete visualization by applying magnetic-guided capsule endoscopy. Gastroenterol Res Pract. 2016; 2016:6471945.


19. Mahoney AW, Abbott JJ. Five-degree-of-freedom manipulation of an untethered magnetic device in fluid using a single permanent magnet with application in stomach capsule endoscopy. Int J Rob Res. 2016; 35:129–147.
20. Yim S, Sitti M. Design and rolling locomotion of a magnetically actuated soft capsule endoscope. IEEE Trans Robot. 2012; 28:183–194.


21. Liao Z, Duan XD, Xin L, et al. Feasibility and safety of magnetic-controlled capsule endoscopy system in examination of human stomach: a pilot study in healthy volunteers. J Interv Gastroenterol. 2012; 2:155–160.


22. Keller H, Juloski A, Kawano H, et al. Method for navigation and control of a magnetically guided capsule endoscope in the human stomach. In : In: 2012 4th IEEE RAS & EMBS International Conference on Biomedical Robotics and Biomechatronics (BioRob); 2012 Jun 24-27; Rome, Italy. Piscataway Township (NJ). IEEE. 2012. p. 859–865.


23. Rey JF, Ogata H, Hosoe N, et al. Feasibility of stomach exploration with a guided capsule endoscope. Endoscopy. 2010; 42:541–545.


24. Denzer UW, Rösch T, Hoytat B, et al. Magnetically guided capsule versus conventional gastroscopy for upper abdominal complaints: a prospective blinded study. J Clin Gastroenterol. 2015; 49:101–107.
25. Ciuti G, Donlin R, Valdastri P, et al. Robotic versus manual control in magnetic steering of an endoscopic capsule. Endoscopy. 2010; 42:148–152.


26. Liao Z, Hou X, Lin-Hu EQ, et al. Accuracy of magnetically controlled capsule endoscopy, compared with conventional gastroscopy, in detection of gastric diseases. Clin Gastroenterol Hepatol. 2016; 14:1266–1273. e1.
27. Zou WB, Hou XH, Xin L, et al. Magnetic-controlled capsule endoscopy vs. gastroscopy for gastric diseases: a two-center self-controlled comparative trial. Endoscopy. 2015; 47:525–528.


28. Valdastri P, Webster RJ III, Quaglia C, Quirini M, Menciassi A, Dario P. A new mechanism for mesoscale legged locomotion in compliant tubular environments. IEEE Trans Robot. 2009; 25:1047–1057.


29. Quirini M, Menciassi A, Scapellato S, et al. Feasibility proof of a legged locomotion capsule for the GI tract. Gastrointest Endosc. 2008; 67:1153–1158.


30. Quirini M, Menciassi A, Scapellato S, Stefanini C, Dario P. Design and fabrication of a motor legged capsule for the active exploration of the gastrointestinal tract. IEEE ASME Trans Mechatron. 2008; 13:169–179.


31. Gorini S, Quirini M, Menciassi A, Pernorio G, Stefanini C, Dario P. SMA-based actuator for a legged endoscopic capsule. In : In: The First IEEE/RAS-EMBS International Conference on Biomedical Robotics and Biomechatronics; 2006 Feb 20-22; Pisa, Italy. Piscataway Township (NJ). IEEE. 2006. p. 443–449.
32. Park S, Park H, Park S, Kim B. A paddling based locomotive mechanism for capsule endoscopes. Journal of Mechanical Science and Technology. 2006; 20:1012–1018.


33. Kim HM, Yang S, Kim J, et al. Active locomotion of a paddling-based capsule endoscope in an in vitro and in vivo experiment (with videos). Gastrointest Endosc. 2010; 72:381–387.


34. Kim B, Lee S, Park JH, Park J-O. Design and fabrication of a locomotive mechanism for capsule-type endoscopes using shape memory alloys (SMAs). IEEE ASME Trans Mechatron. 2005; 10:77–86.


35. Kim B, Park S, Jee CY, Yoon S-J. An earthworm-like locomotive mechanism for capsule endoscopes. In : In: 2005 IEEE/RSJ International Conference on Intelligent Robots and Systems; 2005 Aug 2-6; Edmonton, Canada. Piscataway Township (NJ). IEEE. 2005. p. 2997–3002.


36. Morita E, Ohtsuka N, Shindo Y, et al. In vivo trial of a driving system for a self-propelling capsule endoscope using a magnetic field (with video). Gastrointest Endosc. 2010; 72:836–840.


37. Tortora G, Valdastri P, Susilo E, et al. Propeller-based wireless device for active capsular endoscopy in the gastric district. Minim Invasive Ther Allied Technol. 2009; 18:280–290.


38. Carta R, Tortora G, Thoné J, et al. Wireless powering for a self-propelled and steerable endoscopic capsule for stomach inspection. Biosens Bioelectron. 2009; 25:845–851.


39. Gorlewicz JL, Battaglia S, Smith BF, et al. Wireless insufflation of the gastrointestinal tract. IEEE Trans Biomed Eng. 2013; 60:1225–1233.


40. Pasricha T, Smith BF, Mitchell VR, et al. Controlled colonic insufflation by a remotely triggered capsule for improved mucosal visualization. Endoscopy. 2014; 46:614–618.


41. Ching HL, Hale MF, McAlindon ME. Current and future role of magnetically assisted gastric capsule endoscopy in the upper gastrointestinal tract. Therap Adv Gastroenterol. 2016; 9:313–321.


42. Imagawa H, Oka S, Tanaka S, et al. Improved detectability of small-bowel lesions via capsule endoscopy with computed virtual chromoendoscopy: a pilot study. Scand J Gastroenterol. 2011; 46:1133–1137.


43. Matsumura T, Arai M, Sato T, et al. Efficacy of computed image modification of capsule endoscopy in patients with obscure gastrointestinal bleeding. World J Gastrointest Endosc. 2012; 4:421–428.


44. Park S, Koo K-I, Bang SM, Park JY, Song SY, Cho D. A novel microactuator for microbiopsy in capsular endoscopes. J Micromech Microeng. 2008; 18:025032.


45. Kong K-C, Cha J, Jeon D, Cho D-I. A rotational micro biopsy device for the capsule endoscope. In : In: 2005 IEEE/RSJ International Conference on Intelligent Robots and Systems; 2005 Aug 2-6; Edmonton, Canada. Piscataway Township (NJ). IEEE. 2005. p. 1839–1843.


Fig. 1.
System jointly developed by Olympus and Siemens (robot-assisted manipulation). (A) Capsule endoscope. (B) Guidance magnet. (C) User interface. Adapted from Keller et al. [22] with permission from IEEE.
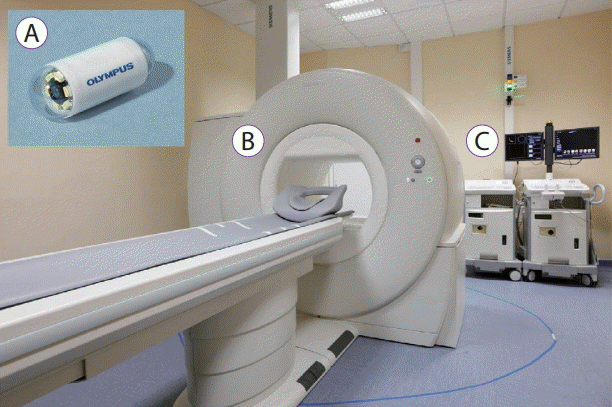
Fig. 2.
NaviCam capsule endoscope and magnetic control system (robot-assisted manipulation). (A) NaviCam capsule endoscope. (B) NaviCam magnetic control system. Adapted from Liao et al. [26] with permission from Elsevier.
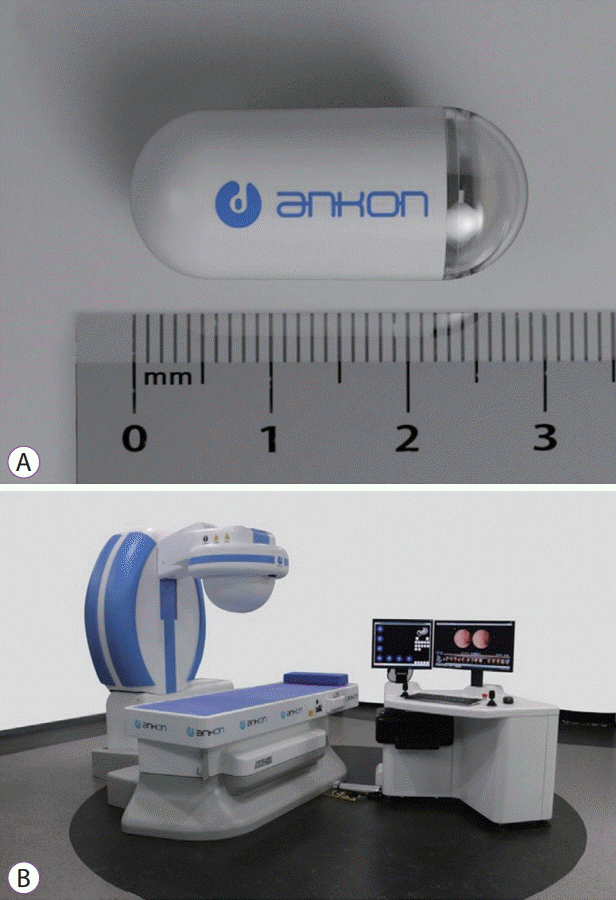
Fig. 3.
MiroCam-Navi system (hand-held manipulation). (A) Real-time viewer. (B) Receiver and sensor. (C) Hand-held magnet. (D) MiroCam-Navi capsule. Adapted from Rahman et al. [14] with permission from Elsevier.
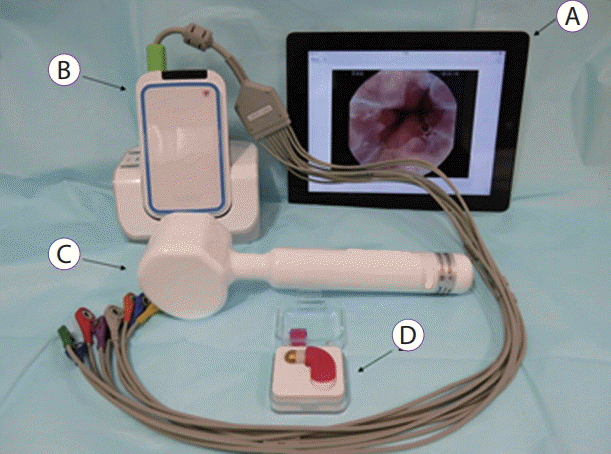
Table 1.
Clinical Trials of Capsule Endoscopy in Gastric Evaluation
Author | Year | Actuation mode | Manipulation | n | Study design | Accuracy for gastric lesions | Safety (adverse events) | Note |
---|---|---|---|---|---|---|---|---|
Swain et al. [17] | 2010 | External magnetic | Hand-held magnet | 1 | Pilot study (case report) | N/A | none | First human trial |
Modified colon capsule (Given Imaging Ltd., Yoqneam, Israel) with external magnet paddle | ||||||||
Rey et al. [23] | 2010 | External magnetic | Robotic control | 53 | Pilot study (case series) | N/A | 0% | Magnetic driving system jointly developed by Olympus Medical Systems Corporation (Tokyo, Japan) and Siemens Healthcare (Erlangen, Germany) |
Keller et al. [16] | 2011 | External magnetic | Hand-held magnet | 10 | Pilot study (case series) | N/A | 0% | Modified colon capsule (Given Imaging Ltd.) with external magnet paddle |
Liao et al. [21] | 2012 | External magnetic | Robotic control | 34 | Pilot study (case series) | N/A | 0% | NaviCam (Ankon Technologies Co. Ltd., Wuhan, China) |
Rey et al. [4] | 2012 | External magnetic | Robotic control | 61 | Pilot study (single-center, prospective, self-controlled, blinded trial) | N/A | 3/61 (4.9%) | Magnetic driving system jointly developed by Olympus Medical Systems Corporation and Siemens Healthcare |
Jun et al. [6] | 2013 | Passive (no actuation) | Passive (no actuation) | 8 | Pilot study (case series) | 50%a) | 0% | PillCam ESO (Given Imaging Ltd.) with various position changes |
Denzer et al. [24] | 2015 | External magnetic | Robotic control | 189 | Two-center, prospective, self-controlled, blinded trial | 88.1%–90.5% | 0% | PillCam ESO (Given Imaging Ltd.) with innominate magnetic guidance system |
Zou et al. [27] | 2015 | External magnetic | Robotic control | 68 | Two-center, prospective, self-controlled, blinded trial | 91.2% | 3/68 (4.4%) | NaviCam (Ankon Technologies Co. Ltd.) |
Qian et al. [18] | 2016 | External magnetic | Robotic control | 60 | Case series | N/A | N/A | NaviCam (Ankon Technologies Co. Ltd.) |
Liao et al. [26] | 2016 | External magnetic | Robotic control | 350 | Multicenter, prospective, self-controlled, blinded trial | 93.4% | 5/350 (1.4%) | NaviCam (Ankon Technologies Co. Ltd.) |
Rahman et al. [14] | 2016 | External magnetic | Hand-held magnet | 26 | Pilot study (self-controlled comparative study) | 96%a) | N/A | MiroCam-Navi (Intromedic Ltd., Seoul, Korea) |