Abstract
Objective
To assess the influence of cognitive therapy, in combination with cognitive software, on manual dexterity in individuals with multiple sclerosis (MS).
Methods
The Nine-Hole Peg Test (NHPT) was used to establish the eligibility of individuals with MS for testing and to assess their upper limb performance. In addition to standard upper limb rehabilitation, 20 participants received RehaCom-based visual-motor therapy, administered three times a week in 20-minute routines.
Results
A significant relationship was found between the use of manual therapy that utilized the cognitive function platform and the improvement of the non-dominant hand (p=0.037). Compared to controls, the experimental group scored higher on the NHPT, when using the dominant hand (p=0.007). All members of the experimental group, aged ≤60 years, needed considerably less time to do the NHPT with the dominant hand (p=0.008).
Multiple sclerosis (MS) is a chronic inflammatory disease of the central nervous system that leads to the impairment of motor, visual, and cognitive functions [1]. The task of improving these functions in individuals with MS represents one of the greatest challenges of modern neurorehabilitation.
Cognitive impairment, which affects 65% of MS individuals, and the progressive deterioration of motor skills have an adverse effect on quality of life [2]. Impairment of cognitive functions such as processing speed, learning, memory, or executive functions potentiates motor deficiency in MS sufferers [3,4]. Limitations in envisioning a movement, typical of individuals with MS, results in reduced upper limb motor efficiency [5]. These deficits can be ameliorated by utilizing motor imagery training, which has been found to stimulate the somatosensory system and consequently enhance mobility [6].
Traditional rehabilitation methods tend to focus on motor skill improvement rather than on cognitive function improvement, which is crucial for individuals with MS. Cognitive software has previously been utilized for the improvement and restoration of cognitive functions in MS sufferers [7].
Studies of MS individuals showed that declines in cognitive function translate into the exacerbation of movement disorders [8,9]. Studies on patients in the early stages of dementia demonstrated that combining cognitive therapy with movement rehabilitation produced the most noteworthy results [8]. The combined application of motor and cognitive therapies produced improvement in dual-task gait performance. Similarly, positive effects were observed when the combined therapies were used to treat patients with a recent history of stroke [9,10]. Further, gait velocity in MS patients improved when standard physical therapy was complemented by cognitive training tasks. While a number of cognitive therapies are in common use, those utilizing computer technology are quickly gaining popularity as they have proven to be highly effective [11].
One such computer-based method, applied for the improvement of cognitive functions, is RehaCom—a program that features three main treatment strategies: psychoeducation and awareness of cognitive functions, enhancement of motivational functions, and training of compensatory and adaptation skills. The program’s exercises are aimed at improving attention, concentration, learning and executive functions. This eventually leads to improving control over adaptive skills aimed at managing or overcoming cognitive impairments. The exercises feature everyday situations and are designed to enable an individual to transition smoothly from engaging in computer-controlled practice to satisfying their real-life cognitive needs. Difficulty levels are automatically tailored to suit each individual’s potential and increase with their progress [12].
In our study, we aimed to assess the effect of cognitive therapy with the RehaCom visual-motor module on the improvement of manual dexterity in individuals with MS. We also attempted to establish whether factors such as age, sex, and extent of disability, as measured by the Expanded Disability Status Scale (EDSS), contribute to the outcomes of this therapy.
The initial group comprised 86 individuals, including 61 females and 25 males (72% and 28%, respectively) diagnosed with MS. The level of disability and progression of the disease were established using the EDSS [13]. The EDSS scores ranged from 1.5–4 (mean score, 2.1). Seventy-three (85%) of the cohort had relapsingremitting MS and 13 (15%) had progressive MS. Assessment of the central nervous system, including manual dexterity, gait, and cognitive functions, was carried out by means of the Multiple Sclerosis Functional Composite [14]. Cerebral lateralization was determined by means of a clinical-experimental method developed by Radtke [15]. The assessment of cognitive functions was conducted using the Mini Mental State Examination scale (MMSE scores varied between 26–30; mean score 27.5). Visual field disorders were excluded in the ophthalmological examination. Before the commencement of the study, we obtained written consent from all participants. Relevant approval from the Ethics Committee was obtained prior to conducting the research on March 7, 2017 (Approval No. KB-0012/102/03/17). The study has been registered with ClinicalTrials.gov (ID: NCT03693118; Influence of Cognitive Function Software Therapy on the Improvement of Manual Skills in Multiple Sclerosis Patients).
The Nine-Hole Peg Test (NHPT) was administered to all MS participants to evaluate their manual dexterity. This standardized scale allowed for high-validity scoring (right, r=0.984; left, r=0.993). Each participant was to insert 9 small pegs into 9 holes on a specially devised board, as quickly as possible. Participants had the opportunity to familiarize themselves with the completion of the test, prior to the actual trial. The test was first conducted using the dominant hand and then with the nondominant hand. If a peg was dropped, the test would be repeated [16].
Participation in the cognitive therapy session was conditional upon participants’ NHPT scores and upon their meeting the inclusion criteria: age range between 18 and 65 years, MS clinically diagnosed based on McDonald’s criteria of 2011 [17], EDSS range from 1.5–4 points, and impairment of the upper limb, as indicated by an NHPT score <2 standard deviations (SDs) from the norm for their age and sex [16].
Mental disorders (MMSE score <26), alcoholism, and severe vision disorders including diplopia and coinciding upper limb therapy constituted the exclusion criteria.
Of the 86 MS individuals who completed the NHPT, 40 individuals presented dysfunctions in both hands and fulfilled the eligibility criteria for the study. All 40 individuals had relapsing-remitting MS. These participants were then subdivided randomly into two groups—test and control—consisting of 20 people each. The first 20 participants to complete the training were allocated to the experimental group whereas those who took longer to finish the training comprised the control group. Participants in each group were administered individualized, progressive hand therapy treatments. The experimental group participants, however, were administered hand therapy complemented with the cognitive function program. None of the participants were informed of the employed methodology or the expected outcome. Similarly, the assistant carrying out the final NHPT test did not know which group the examined individual belonged to.
Three times a week, the experimental group received upper limb treatment with the RehaCom cognitive function platform. A visual-motor coordination module consisting of 96 levels was used for training. The key features of the training task included an object (rotor) moving along a pre-defined track (pattern of movement) and a cursor controlled by a joystick. To perform the task, a patient was supposed to move the cursor onto the rotor, which activated the rotor and made it move along a selected track. The patient’s task was to keep the cursor over the moving rotor at all times. Each task was deemed to be completed when the total time the cursor was over the rotor did not exceed the preset time brackets. If the maximum time for performing a particular task was exceeded, the patient had to redo it. By doing this, the patient gradually perfected a certain type of movement pattern [12].
The participants were expected to do all 96 levels of the visual-motor coordination task within 3 months or the treatment would be terminated. Training time ranged from 540–640 minutes (mean, 586 minutes). Prior to the first treatment session, patients were provided with all relevant instructions and information about the test was displayed on the monitor. They could also choose which hand they would start with. They were instructed to change hands following the completion of each task.
After completing each task, the patient would move on to a higher level of difficulty. Failure meant having to redo the failed task. A single training session lasted 20 minutes followed by an optional break. The level of difficulty of each exercise was adjusted to suit the individual abilities of each participant.
Having completed the training, each participant was administered the NHPT again so that we could assess their upper limb efficiency. The time between the first and the second NHPT trial varied, depending on the duration of the training, but was not longer than 3 months. Those who completed the training had 7 days to do the test. The therapy was carried out by the lead researcher. Of the initial group, only 10 participants managed to complete the training. The large number of dropouts was caused by patient’s progressive deterioration of health and factors including a relapse of the disease, failure to turn up for the trial (despite a prior declaration of intention to participate), and family issues.
Like the experimental group, the control group was made up of 20 people with MS who were administered the NHPT twice. Unlike the experimental group, the control group did not receive RehaCom hand therapy.
The statistical analysis was carried out with a licensed version of Statistica 12 (StatSoft Inc., Tulsa, OK, USA). Descriptive statistics (mean, median, upper and lower quartiles) were calculated for each group. The ShapiroWilk test was used for the assessment of normality, while Levene test was used to assess homogeneity of variance. Since a normal distribution of the tested variables was not obtained, the variables in both groups were assessed using the Mann-Whitney U test.
Depending on the sample size, either a chi-squared test or Fisher test were used to analyze quantitative data. The Wilcoxon signed-rank test was used in selected groups for the analysis of both NHPT scores. The assumed level of statistical significance was p≤0.05.
A comparative analysis of the data obtained from the study did not reveal any significant differences between the experimental group and the control group. The former, who received cognitive training, were found to take longer to do the second NHPT than the latter. This difference, however, was not statistically significant (Tables 1, 2).
Fig. 1 illustrates the initial and final NHPT results for the dominant hand and non-dominant hand in both the experimental and control groups. Compared to their initial dominant hand score, the experimental group had a lower final mean NHPT score (p=0.007). Compared to their initial non-dominant hand score, the experimental group had a lower final mean NHPT score (p=0.037). No such significant differences were observed in the control group.
Analysis of NHPT scores before and after the therapy (Table 3) showed that, following treatment, the experimental group needed significantly less time to perform the test with both the dominant (p=0.007) and non-dominant hand (p=0.037). No such significant difference was observed in the control group. All members of the experimental group, who were aged ≤60 years, took considerably less time to do the NHPT test with the dominant hand (p=0.008). The cutoff point was set at ≤60 in order to ensure relatively equal and representative populations within each group. Individuals aged >60 tend to display a rapid acceleration of decline in general health and physical fitness, which translates to a considerable loss of muscle fibers, reduction of fiber length, and a consequent, significant decrease in upper limb strength [18,19].
Therapies for individuals with MS aim to minimize their neurologic deficits and improve their quality of life. Motor skills enhancement is a complex process, consisting of three essential stages: cognitive (learning a movement), associative (correcting errors), and autonomous (movement automatization) [20]. To achieve progress at each of these stages, therapists can now take advantage of a well devised computer-assisted training program. Such programs may provide an attractive alternative to traditional therapies, appealing particularly to young people with MS [21].
The cognitive therapy program we applied was found to fulfil the stated therapeutic objectives. It is primarily based on the so-called tracking task, which requires the patient to perform a movement according to instructions displayed on the screen. The MS participants could not advance to the next level of difficulty without first being successful on the current level.
Existing studies show that exercises aimed at improving the precision of a particular movement, which allow for the possibility of repeating and correcting the movement, considerably accelerate its mastering and automation [12].
The MS individuals who participated in our study learned to control various movement patterns: simple linear movements, combined linear movements, curved movements, combined simple linear and curved movements, as well as a pseudo stochastic movement. At the highest difficulty level, the participants were expected to learn 32 movement patterns with varying degrees of speed and complexity.
Repeating particular motor tasks has been found to improve visual-motor coordination as well as the ability to anticipate individual patterns of movement. It has also been established that visuospatial functions are needed for the proper functioning of visual-motor skills. Handeye coordination has been found to improve when an individual is unable to assess the distance and location of the point where the object is to be placed [22–24].
The cognitive program we applied consisted of conventional hand therapy, augmented by the stimulation of visuospatial perception, attention, and response time. These extra features constitute an important element of the program as they address the deficits, about which MS patients most frequently complain. One set of tasks contained in the program features “tracking-tasks” and is designed to improve eye-hand coordination. The person performing the task—the operator—is expected to make a strictly defined movement in response to a static or dynamic stimulus. This requires the operator to process visual information and respond with a particular motor action. The more unpredictable or complex the tasks are, the better hand-eye coordination they require. This training aims to improve patients’ visual-motor skills [25].
Combining cognitive training, which stimulates a number of functions, with upper limb therapy resulted in better performance of the limb. This was reflected in the higher NHPT scores achieved by the individuals who underwent the therapy, as compared to the controls, who were treated only with conventional rehabilitation methods. RehaCom-based cognitive therapy in conjunction with traditional motor training may activate a number of cortical areas simultaneously. This facilitates compensatory processes, leads to accelerated improvement of a patient’s motor functions, and consequently shortens the rehabilitation period of MS patients.
Additionally, patients receive immediate feedback on their performance, which translates into increased speed and better training outcomes. Another potential benefit of this combined and extended treatment program is the exploitation of screen and computer technology. As such technology typically appeals to younger generations, the therapy could prove more effective when administered to young and middle-aged patients [26]. Effective therapy typically leads to a faster return to normal life and work. However, evidence for the effectiveness of the therapy, in this regard, requires further research to be validated.
The MS participants in this study, who underwent the RehaCom therapy regimen, have showed noticeable improvement in the manual functions of both their dominant and non-dominant limbs. This improvement was more prominent in the dominant arm, which is in line with existing findings concerning differences between the limbs.
It was found that the dominant upper limb is more efficient than its counterpart due to its comparatively frequent use in everyday activities. The non-dominant hand is mainly supplementary to the dominant one. The development and structure of brain activation networks depends on which hand tends to be used more often for complex movements. MS progression leads to the loss of compensatory capacity in the non-dominant hand [27]. This, however, was not confirmed by the findings of our study, which showed that the combined therapy resulted in improvement in the performance of the non-dominant hand as well.
Increased efficiency of the non-dominant upper limb in our study participants may confirm the hypothesis of the cross-activation model. According to this model, providing physical treatment to the dominant upper limb activates (through the corpus colossus) the non-dominant upper limb. Applying such treatment to the non-dominant limb, however, does not result in similar activation in the opposing hand [28].
The improvement in the efficiency of the upper limb, observed in our patients after the RehaCom therapy, may be attributed to their low EDSS scores of <3. According to some studies, neuroplasticity and the ability to learn new motor skills is possible in early stages of the disease and decreases as the disability worsens. The ability to learn in patients with EDSS <4 is reduced due to the development of more extensive MS lesions, affecting neural pathways [29].
The positive effect that cognitive therapy had on the manual dexterity our MS participants may also be due to the influence of ideokinetic imagery on motor stimulation. The RehaCom program utilizes a technique in which individuals must maintain visual concentration while performing a specified upper limb movement. The imagery constitutes an alternative source of information and supports motor functions. It has been shown that ideokinetic imagery can actually affect the force, precision, speed, and efficiency of a movement as well as motivation [30]. This may be related to the “chaotic motor imagery” theory.
This theory assumes that imagining a movement prior to its actual execution improves the motor efficiency of the limbs. Hitting the target goals, which we set for our study participants, required them to practice skills that depend on hand-eye coordination. This, in turn, leads to improvement in cognitive functions [31-33].
The outcomes of our study demonstrate that the application of ideokinetic imagery in MS therapies contributes to better mobility of the limbs. Unfortunately this mobility goes on to decrease, in line with an increase in severity of cognitive impairment. Combined visual stimulation and hand therapy can provide a valuable supplement to traditional physical therapy. The current study also demonstrated that a clinical course of the disease, progressive impairment, and advanced age adversely affect both manual skills and rehabilitation effectiveness. Using their dominant limbs, patients over 60 years old, who had EDDS scores <3, did worse on the NHPT than their younger counterparts with similar EDSS results. This difference may be attributable to MS-related fatigue and the increasing impairment of cognitive functions [34,35]. Additionally, participants with lower EDSS scores showed greater improvement in performance with the dominant upper limb. This may indicate that using a cognitive function program, applied during upper limb therapy, leads to better results in individuals whose conditions are less severe [36,37].
Another study, involving the observation of a cohort of 1,463 people with MS for a period of 6–12 months, revealed an association between age and progression of the disease [38,39].
Persistence and motivation towards training are essential components of a successful MS therapy. Many people with MS discontinue treatment due to insufficient selfdiscipline, lack of proper motivation, or negative attitude. It was concluded that only well motivated and positively predisposed individuals can take full advantage of virtual therapy [40].
We experienced similar problems with the participants in our study. As many as 50% of participants failed to complete the cognitive software therapy. Of the remaining participants, 6 dropped out after only 2 rehabilitation sessions (2 weeks). This impeded our ability to observe the full effects of the therapy. The low number of the patients who completed the study severely interfered with the collection of reliable data.
Indeed, the small size of the group that completed the study precluded a more complete statistical analysis. Despite this, thestudy yielded promising results that support the administering of joint rehabilitation therapies in order to address both motor and cognitive systems. However, further research, including more MS participants from more population-representative cohorts, is required to confirm the validity and reliability of our findings.
In future, additional incentive programs should be considered to mobilize people with MS to take action. Longterm follow-up studies are necessary to ascertain the stability of cognitive improvements and the degree to which they generalize to everyday functional activities.
In conclusion, the application of cognitive software training for manual therapy in people with MS has positive effects on the use of both dominant and nondominant hands. More evident effects of dominant limb therapy are demonstrated in patients with EDSS scores <3. Improvement in the efficiency of the upper limbs was observed in patients aged <60 years. Less upper limb improvement was observed in patients whose EDSS scores were <3.
Notes
Conceptualization: Pawlukowska W. Methodology: Pawlukowska W, Dobrowolska N, Koziarska D. Formal analysis: Szylinska A. Funding acquisition: Rotter I. Project administration: Pawlukowska W. Visualization: Pawlukowska W. Writing – original draft: Meller A. Writing – review and editing: Nowacki P, Rotter I. Approval of final manuscript: all authors.
ACKNOWLEDGMENTS
The work was financed from statutory money of the Department of Medical Rehabilitation and Clinical Physiotherapy, headed by dr hab. Iwona Rotter.
REFERENCES
1. Comi G, Radaelli M, Soelberg Sorensen P. Evolving concepts in the treatment of relapsing multiple sclerosis. Lancet. 2017; 389:1347–56.


2. Potagas C, Giogkaraki E, Koutsis G, Mandellos D, Tsirempolou E, Sfagos C, Vassilopoulos D. Cognitive impairment in different MS subtypes and clinically isolated syndromes. J Neurol Sci. 2008; 267:100–6.


3. Tacchino A, Saiote C, Brichetto G, Bommarito G, Roccatagliata L, Cordano C, et al. Motor imagery as a function of disease severity in multiple sclerosis: an fMRI study. Front Hum Neurosci. 2018; 11:628.


4. Filippi M, Riccitelli G, Mattioli F, Capra R, Stampatori C, Pagani E, et al. Multiple sclerosis: effects of cognitive rehabilitation on structural and functional MR imaging measures: an explorative study. Radiology. 2012; 262:932–40.
5. Ytterberg C, Johansson S, Andersson M, Widen Holmqvist L, von Koch L. Variations in functioning and disability in multiple sclerosis: a two-year prospective study. J Neurol. 2008; 255:967–73.
6. Tomassini V, Johansen-Berg H, Jbabdi S, Wise RG, Pozzilli C, Palace J, et al. Relating brain damage to brain plasticity in patients with multiple sclerosis. Neurorehabil Neural Repair. 2012; 26:581–93.


7. Solari A, Motta A, Mendozzi L, Pucci E, Forni M, Mancardi G, et al. Computer-aided retraining of memory and attention in people with multiple sclerosis: a randomized, double-blind controlled trial. J Neurol Sci. 2004; 222:99–104.


8. Tay L, Lim WS, Chan M, Ali N, Chong MS. A combined cognitive stimulation and physical exercise programme (MINDVital) in early dementia: differential effects on single- and dual-task gait performance. Gerontology. 2016; 62:604–10.


9. Faria AL, Cameirao MS, Couras JF, Aguiar JR, Costa GM, Bermudez I Badia S. Combined cognitive-motor rehabilitation in virtual reality improves motor outcomes in chronic stroke: a pilot study. Front Psychol. 2018; 9:854.


10. Kim GY, Han MR, Lee HG. Effect of dual-task rehabilitative training on cognitive and motor function of stroke patients. J Phys Ther Sci. 2014; 26:1–6.
11. Sosnoff JJ, Socie MJ, Sandroff BM, Balantrapu S, Suh Y, Pula JH, et al. Mobility and cognitive correlates of dual task cost of walking in persons with multiple sclerosis. Disabil Rehabil. 2014; 36:205–9.


12. HASOMED. RehaCom – cognitive therapy [Internet]. Magdeburg, Germany: HASOMED GmbH;c2020. [cited 2020 Mar 10]. Available from: http://www.hasomed.de/en/products/rehacom-cognitive-therapy.html.
13. Kurtzke JF. Rating neurologic impairment in multiple sclerosis: an expanded disability status scale (EDSS). Neurology. 1983; 33:1444–52.


14. Fischer JS, Rudick RA, Cutter GR, Reingold SC. The Multiple Sclerosis Functional Composite Measure (MSFC): an integrated approach to MS clinical outcome assessment. Mult Scler. 1999; 5:244–50.


15. Radtke MB. A set of methods for diagnosing lateralization. [place unknown]: Laboratory of Psychological and Pedagogical Tests at SEBG;2011. Available from: https://www.pracowniatestow.pl/pl/p/Zestawmetod-diagnozy-lateralizacji/91.
16. Oxford Grice K, Vogel KA, Le V, Mitchell A, Muniz S, Vollmer MA. Adult norms for a commercially available Nine Hole Peg Test for finger dexterity. Am J Occup Ther. 2003; 57:570–3.


17. Polman CH, Reingold SC, Banwell B, Clanet M, Cohen JA, Filippi M, e al. Diagnostic criteria for multiple sclerosis: 2010 revisions to the McDonald criteria. Ann Neurol. 2011; 69:292–302.


18. Boatright JR, Kiebzak GM, O’Neil DM, Peindl RD. Measurement of thumb abduction strength: normative data and a comparison with grip and pinch strength. J Hand Surg Am. 1997; 22:843–8.


20. Taylor JA, Ivry RB. The role of strategies in motor learning. Ann N Y Acad Sci. 2012; 1251:1–12.


21. Plohmann AM, Kappos L, Ammann W, Thordai A, Wittwer A, Huber S, et al. Computer assisted retraining of attentional impairments in patients with multiple sclerosis. J Neurol Neurosurg Psychiatry. 1998; 64:455–62.


22. Guedon O, Gauthier G, Cole J, Vercher JL, Blouin J. Adaptation in visuomanual tracking depends on intact proprioception. J Mot Behav. 1998; 30:234–48.


23. Gauthier GM, Vercher JL, Mussa Ivaldi F, Marchetti E. Oculo-manual tracking of visual targets: control learning, coordination control and coordination model. Exp Brain Res. 1988; 73:127–37.


24. Ono S. The neuronal basis of on-line visual control in smooth pursuit eye movements. Vision Res. 2015; 110(Pt B):257–64.


25. Matotek K, Saling MM, Gates P, Sedal L. Subjective complaints, verbal fluency, and working memory in mild multiple sclerosis. Appl Neuropsychol. 2001; 8:204–10.


26. Gilmore N, Ross K, Kiran S. The intensive cognitivecommunication rehabilitation program for young adults with acquired brain injury. Am J Speech Lang Pathol. 2019; 28(1S):341–58.


27. Jacobs J. The influence of hand dominance on the expression of arm-hand dysfunction and its relationship with the underlying neurophysiological disturbances in multiple sclerosis [master’s thesis]. Hasselt, Belgium: Hasselt University;2014.
28. Parlow SE, Kinsbourne M. Asymmetrical transfer of training between hands: implications for interhemispheric communication in normal brain. Brain Cogn. 1989; 11:98–113.


29. Casadio M, Sanguineti V, Morasso P, Solaro C. Abnormal sensorimotor control, but intact force field adaptation, in multiple sclerosis subjects with no clinical disability. Mult Scler. 2008; 14:330–42.


30. Sharma N, Baron JC, Rowe JB. Motor imagery after stroke: relating outcome to motor network connectivity. Ann Neurol. 2009; 66:604–16.


31. Heremans E, D’hooge AM, De Bondt S, Helsen W, Feys P. The relation between cognitive and motor dysfunction and motor imagery ability in patients with multiple sclerosis. Mult Scler. 2012; 18:1303–9.


32. Lacourse MG, Orr EL, Cramer SC, Cohen MJ. Brain activation during execution and motor imagery of novel and skilled sequential hand movements. Neuroimage. 2005; 27:505–19.


33. Adamovich SV, Fluet GG, Tunik E, Merians AS. Sensorimotor training in virtual reality: a review. NeuroRehabilitation. 2009; 25:29–44.


34. Lange BS, Requejo P, Flynn SM, Rizzo AA, Valero-Cuevas FJ, Baker L, et al. The potential of virtual reality and gaming to assist successful aging with disability. Phys Med Rehabil Clin N Am. 2010; 21:339–56.


35. Confavreux C, Vukusic S. Age at disability milestones in multiple sclerosis. Brain. 2006; 129(Pt 3):595–605.


36. Briken S, Gold SM, Patra S, Vettorazzi E, Harbs D, Tallner A, et al. Effects of exercise on fitness and cognition in progressive MS: a randomized, controlled pilot trial. Mult Scler. 2014; 20:382–90.


37. Forn C, Rocca MA, Valsasina P, Bosca I, Casanova B, Sanjuan A, et al. Functional magnetic resonance imaging correlates of cognitive performance in patients with a clinically isolated syndrome suggestive of multiple sclerosis at presentation: an activation and connectivity study. Mult Scler. 2012; 18:153–63.


38. Tremlett H, Paty D, Devonshire V. Disability progression in multiple sclerosis is slower than previously reported. Neurology. 2006; 66:172–7.


39. Scalfari A, Neuhaus A, Daumer M, Ebers GC, Muraro PA. Age and disability accumulation in multiple sclerosis. Neurology. 2011; 77:1246–52.


40. Massetti T, Trevizan IL, Arab C, Favero FM, RibeiroPapa DC, de Mello Monteiro CB. Virtual reality in multiple sclerosis: a systematic review. Mult Scler Relat Disord. 2016; 8:107–12.
Fig. 1.
The analysis of the initial and final Nine-Hole Peg Test (NHPT) scores regarding the dominant hand and non-dominant hand.
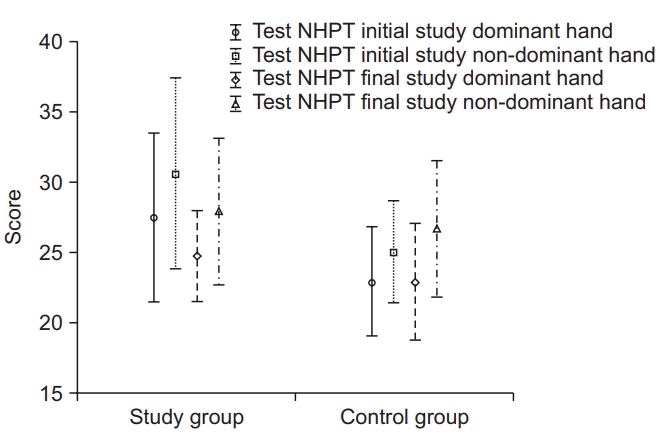
Table 1.
A comparative analysis of the age, EDSS scores, age of onset and duration of the disease in the study and control groups
Variable |
Control group (n=20) |
Study group (n=10) |
p-value | ||||||
---|---|---|---|---|---|---|---|---|---|
Mean | Q25 | Median | Q75 | Mean | Q25 | Median | Q75 | ||
Age (yr) | 49.6 | 39 | 45 | 63 | 53.9 | 46 | 57.5 | 61 | 0.733a) |
EDSS | 2.75 | 1.5 | 2.5 | 3.5 | 4.1 | 2.5 | 4.25 | 6 | 0.093a) |
Time from symptom onset to diagnosis (yr) | 4.4 | 1 | 2 | 4 | 1.5 | 0 | 1 | 3 | 0.278a) |
Duration of disease (yr) | 11.2 | 7 | 9.5 | 12 | 14.9 | 9 | 11 | 15 | 0.470a) |
Initial NHPT scores | |||||||||
Dominant hand | 22.93 | 19.02 | 20.54 | 28.44 | 27.54 | 23.47 | 26.36 | 28.62 | 0.212a) |
Non-dominant hand | 25.04 | 21.87 | 22.48 | 29.29 | 30.60 | 23.48 | 28.51 | 35.56 | 0.186a) |
Final NHPT scores | |||||||||
Dominant hand | 22.94 | 18.93 | 20.46 | 28.09 | 24.73 | 21.99 | 24.34 | 27.42 | 0.345a) |
Non-dominant hand | 26.68 | 21.89 | 23.94 | 29.89 | 27.91 | 22.35 | 26.08 | 32.23 | 0.623a) |
Table 2.
Characteristics of participants
Control group (n=20) | Study group (n=10) | p-value | |
---|---|---|---|
Sex | 0.626a) | ||
Female | 6 (30.0) | 8 (80.0) | |
Male | 14 (70.0) | 2 (20.0) | |
Age (yr) | 0.675a) | ||
<60 | 6 (30.0) | 6 (60.0) | |
≥60 | 14 (70.0) | 4 (40.0) | |
Education | 0.670a) | ||
Vocational | 2 (10.0) | 3 (30.0) | |
Secondary | 8 (40.0) | 4 (40.0) | |
Tertiary | 10 (50.0) | 3 (30.0) | |
EDSS | 0.185a) | ||
<3 | 6 (30.0) | 3 (30.0) | |
≥3 | 14 (70.0) | 7 (70.0) |
Table 3.
Analysis of the differences between the initial and follow-up NHPT scores for dominant and non-dominant hands in both study and control groups
Dominant hand |
p-valuea) |
Non-dominant hand |
p-valuea) | |||||||
---|---|---|---|---|---|---|---|---|---|---|
Mean | Q25 | Median | Q75 | Mean | Q25 | Median | Q75 | |||
Group | ||||||||||
Control | 0.02 | -0.56 | -0.05 | 1.17 | 0.959 | 1.64 | -0.35 | 0.53 | 3.35 | 0.093 |
Study | -2.82 | -3.12 | -1.31 | -0.44 | 0.007 | -2.69 | -4.36 | -2.42 | -1.13 | 0.037 |
Age (yr) | ||||||||||
<60 | -2.43 | -2.90 | -1.22 | -0.40 | 0.008 | -0.60 | -2.42 | -0.54 | 1.48 | 0.583 |
≥60 | 0.14 | -0.51 | 0.24 | 1.18 | 0.674 | -0.42 | -2.25 | 0.10 | 1.86 | 0.889 |
Sex | ||||||||||
Female | -0.75 | -1.69 | -0.50 | 0.26 | 0.103 | -1.29 | -2.43 | -0.93 | 0.17 | 0.056 |
Male | -2.93 | -3.12 | -0.93 | 0.23 | 0.249 | 1.26 | 0.60 | 2.92 | 3.55 | 0.345 |
EDSS | ||||||||||
<3 | -0.89 | -1.51 | -0.73 | -0.35 | 0.033 | 0.33 | -1.13 | -0.35 | 0.46 | 0.859 |
≥3 | -1.81 | -3.12 | -0.29 | 1.17 | 0.286 | -1.23 | -4.36 | -0.41 | 2.49 | 0.534 |