Abstract
Objective
To investigate changes in (1) the colonic response to acetylcholine (Ach), (2) the muscarinic (M) receptors in the colon, and (3) the levels of colonic contraction-related proteins after a spinal cord injury (SCI).
Method
We divided 16 Sprague-Dawley rats into 2 groups: the control group and the SCI group. A spinal cord transection was performed surgically at the T10 vertebral level. After 1 week, the entire colon was divided into 2 segments, the proximal and distal colon. Each segment was mounted in a longitudinal or circular muscle direction in a 10-ml organ bath. We determined the intergroup differences as percentage changes in contractility after Ach treatment alone, Ach treatment with M2 receptor antagonist (AQ-RA741) pretreatment, and Ach treatment with M3 receptor antagonist (4-DAMP) pretreatment. Western blot analyses were performed to determine the expression level of RhoA, and heat shock protein 27 (HSP27).
Results
Compared to the control rats, the SCI rats showed an increased response to Ach along both the directions in the proximal colon (p<0.05). Compared to the control group, in the SCI group, the Ach response was significantly different in the proximal segment under AQ-RA741 pretreatment (p<0.05) and in the distal segment under 4-DAMP pretreatment (p<0.05). Findings of the western blot analyses showed a significant decrease in the level of protein gene product 9.5 in the proximal and distal colon and a significant increase in the level of RhoA and HSP27 in the proximal colon of the SCI rats.
Spinal cord injury (SCI) leads to the development of neurogenic bowel, which induces chronic constipation, fecal incontinence, and fecal impaction because of a change in large intestinal motility and the impairment of defecation control.1 Although the mechanism of development of neurogenic bowel after SCI is not clearly understood, Longo et al.2 and Menardo et al.3 have reported that in most SCI patients, colonic motility is decreased. Thus, the colonic transit time is prolonged, particularly in the descending and sigmoid colon. Therefore, these authors suggested that the main me chanism of development of constipation in the neurogenic bowel is the reduced motility of the distal large intestine. However, previous studies have shown that after SCI, the colonic transit time was prolonged mainly in the left colon and rectum.3 Also, the transit time in the right and left colon was comparable but that in the rectum and sigmoid colon was prolonged,4 and the transmit time was not prolonged when the regions above the 9th thoracic vertebra were injured.5
The extrinsic innervation controls the motility of the large intestine via the autonomic nervous system, whereas the intrinsic innervation, including the submucosal plexus and myenteric plexus, control the contraction or collaborative movements of the colonic smooth muscles.2
The neurotransmitter acetylcholine (Ach) acts on the smooth muscle muscarinic (M) receptors and is a major determinant of colonic motility. Moreover, the M type 2 (M2) and type 3 (M3) receptors, in particular, are present on intestinal smooth muscles and they are the major excitatory neurotransmitters that control the contraction of intestinal smooth muscles.6
The M receptors consist of 5 subtypes (M1 to M5), and the M2 and M3 receptors are primarily involved in the motility of the gastrointestinal tract.7 The M2 and M3 mRNA and protein were detected in the gastrointestinal smooth muscles by performing northern blot analysis, reverse transcriptase polymerase chain reaction (RT-PCR), and receptor immunoprecipitation.7 In 1991, Zhang et al.6 reported radioligand binding and northern blot analysis that showed 82% of the M receptors on canine colonic smooth muscles were M2, whereas 18% were M3 receptors. Similarly, compared to the M3 receptors, the M2 receptors were the primary and most abundant receptors in the smooth muscles of the bladder. Although the density of the M2 receptors is usually greater than that of the M3 receptors, smooth muscle contraction is mainly mediated by the M3 receptors.7 Under disease states, the number and sensitivity of the M receptors may be altered. For example, the number of M2 receptors increased in the bladder of diabetic rats.8 In rats with SCI, the increase in the density of all M receptors and the change in receptor subtype from M3 to M2 were thought to cause bladder hyperreflexia.9 The findings of these previous reports suggest that SCI may cause a concomitant change in bowel function and the number or sensitivity of the M receptors on the colonic smooth muscles. However, only a few studies on the change of M receptor subtypes in colonic smooth muscles have been reported to date.
We hypothesized that the change in bowel movements after SCI may be associated with an altered Ach response due to the change in M2 or M3 receptors. Therefore, we performed an in vitro organ bath study by dividing the proximal and distal colon along a circular and longitudinal muscle direction in order to examine the changes in contractility of each colonic segment after an SCI, with or without M2 or M3 receptor antagonist pretreatment.
Animal experiments were performed in accordance to the Institutional Guidelines provided by the Committee on Animal Research at Wonkwang University, and efforts were made to minimize both animal suffering and the number of animals. We purchased 16 female Sprague-Dawley rats weighing 200-250 g from Samtaco (BioKorea, Osan, Korea), and these were divided into 2 groups: the group without surgical intervention (control group; n=8), and the group with SCI (SCI group; n=8). All surgical procedures including SCI were performed by the same investigator. All rats received intraperitoneal injections of 300 mg/kg chloral hydrate for induction of anesthesia. Complete spinal cord transection was performed at the T10 vetebral level, and Gelfoam® (Baxter Healthcare Corp., Hayward, USA) was inserted into the lesion site to separate the spinal sections. After the completion of all surgical procedures, the surgical area was sterilized. Gentamicin (100 mg/kg) was injected intramuscularly for 3 days to prevent wound infection.
All rats were killed by cervical dislocation at day 7 after the surgery, after which the abdominal cavity was immediately opened and the entire colon was resected. Some parts of the colonic tissues were frozen and stored immediately after resection for western blot analysis. The remaining parts were immersed in an organ bath filled with Kreb's solution saturated with oxygen (95% O2, 5% CO2) at 37℃. The entire colon was divided into the proximal (within 4 cm from the end of cecum) and distal segment (within 4 cm from the anus). Each segment was cut into 1 cm pieces. One end of the tissue sections was fixed to the base of the organ bath, whereas the other end was connected to a force transducer (FT03; Grass, USA) either longitudinally or in a circular fashion by using cotton threads. The preload tension was adjusted to approximately 1 g. The tissues were allowed to equilibrate for at least 60 min to obtain constant contractions. Spontaneous contractions were recorded. Thereafter, various concentrations of Ach (10-9 M to 10-4 M) were added stepwise and the change in contractility with Ach concentrations was recorded. In addition, tissues pretreated with the M2 or M3 receptor antagonists (10-7 M) were treated with stepwise increasing concentrations of Ach (10-9 M to 10-4 M), and the change in the contractility with increasing Ach concentrations was recorded. All data were recorded using the PowerLab data acquisition system (AD Instruments, Sydney, Australia). From the recorded waveform, the area under the curve was calculated by integrating all the data, and the differences between the maximum and minimum values obtained immediately before and after the drug treatment were compared. Each test lasted for at least 5 min.
Ach, the M2 receptor antagonist 11-[[4-[4-(diethylamino) butyl]-1-piperidinyl]acetyl]-5,11-dihydro-6H-pyridol[2,3-b][1,4] benzodiazepin-6-one (AQ-RA741) and the M3 receptor antagonist 1,1-dimethyl-4-diphenylacetoxypiperidinium iodide (4-DAMP) were purchased from Sigma South Croydon, Australia. Different concentrations of Ach (10-9 M to 10-4 M) and 10-7 M of the antagonists were added to the organ baths containing 10 ml Kreb's solution.
The expression levels of protein gene product 9.5 (PGP 9.5), heat shock protein 27 (HSP 27), and RhoA were measured using western blot analysis. Colonic tissues were washed with Tris buffered saline (TBS), and approximately 5 g tissue was added to lysis buffer (25 mM Tris-Cl, 1 mM ethylene glycol bis [β-aminoethyl ether]-N,N,N',N'-tetraacetic acid, 1 mM dithiothreitol, 0.1% Triton X-100, protease inhibitor cocktail, and phosphatase inhibitor cocktail; pH 7.4) and homogenized. The samples were then centrifuged at 12,000 rpm for 30 min, and the cytosolic fraction was obtained. The protein con centration was estimated using bovine serum albumin (BSA) as a standard. The protein concentration of all samples was adjusted in order to apply the same amount of protein. The sample buffer was added to the samples, the mixture was boiled at 100℃ for 5 min, and electrophoresis was performed at 100 V for 2 h by using a minigel electrophoresis apparatus (mini-PROTEIN Tetra cell; Bio-Rad Laboratories, Inc., Hercules, CA). After electrophoresis, the gels were stained with Coomassie brilliant blue R-250 for 1 h. Following that, the samples were destained with 10% acetic acid and 10% methanol, and the protein bands were observed. The proteins were transferred to 0.45 µm polyvinylidene difluoride (PVDF) membranes (Roche Diagnostics GmbH, Mannheim, Germany) using protein transfer apparatus (mini Transblot cell; Bio-Rad Laboratories) at 100 V for 90 min. To prevent non-specific binding of the primary antibody, the PVDF membranes were incubated with blocking buffer (5% skim milk in 0.05% tween 20-Tris buffer saline [TBST, pH 7.6]) for 1 h. The membranes were washed 3 times with TBST and incubated overnight at 4℃ with primary antibodies (PGP 9.5, HSP 27, and RhoA) that were diluted to 1:1,000 with TBS containing 3% BSA. The membranes were then washed 4 times with TBST. Next, the membranes were incubated for 1 h with a horseradish peroxidase (HRP)-conjugated goat anti-rabbit IgG secondary antibody (Enzo Life Science International Inc., Plymouth Meeting, PA) (1:5,000). The membranes were washed 4 times with TBST, incubated with Immobilon Western chemiluminescent HRP substrate (Millipore., MA, USA), and exposed to a chemiluminescence film in the dark room. The expression levels of PGP 9.5, HSP27, and RhoA were compared. In addition, as a control experiment, double staining for glyceraldehyde-3-phosphate dehydrogenase (GAPDH) was performed with PGP 9.5, HSP27, and RhoA under the same conditions.
Data analysis was conducted using the Statistical Package for the Social Sciences v 11.0 (SPSS Inc., Chicago, USA). Differences in the contractility of tissues treated with different compounds were determined for the control and SCI groups by performing the independent t-test. We considered p-values less than 0.05 as statistically significant.
Fecal impaction in the large intestines of the SCI rats was more than that in the large intestines of the control rats, and distended large intestines were observed in the SCI rats. Fecal impaction was more noticeable in the distal colon. In addition, fecal pellets of the SCI rats were darker and harder than those of the controls rats (Fig. 1).
In the organ bath experiment, the contractility of both the proximal and distal colon increased with increasing concentration of Ach in both control and SCI groups. However, the contractility in the proximal colon after Ach treatment in the SCI group was significantly higher than that in the control group (p<0.05). The intergroup difference for contractility in the distal colon was not significant (Fig. 2).
Pretreatment with AQ-RA741, which primarily blocks the M2 receptors, reduced the response to Ach in both the proximal and distal colon of rats belonging to both the groups. Similar to the results obtained for Ach treatment without any pretreatment, after AQ-RA741 pretreatment, Ach-induced contraction showed a significant increase in the proximal colon of the SCI rats than in the proximal colon of the control rats (Fig. 3, p<0.05). In contrast, after pretreatment with 4-DAMP, which primarily blocks the M3 receptors, the response to Ach in both proximal and distal colon was significantly reduced. In addition, treatment of the colon specimens, except the longitudinal muscle direction in the proximal colon, with high concentration Ach (>10-5 M) did not produce any differences in Ach-induced contraction in the proximal and the distal colon (Fig. 4, p < 0.05).
The results of the western blot analysis showed that the expression of RhoA and HSP27 in the distal colon was higher than that observed in the proximal colon in both groups. The expression of these proteins in the proximal colon of the SCI rats was significantly higher than that in the proximal colon of the control rats (Fig. 5-A, C, and D, p<0.05). In contrast, the expression of PGP 9.5 in the proximal and distal colon of the SCI rats was significantly lower than that in the proximal and distal colon of the control rats (Fig. 5-A and B, p<0.05).
Reasons for difficulty with defecation after SCI have not yet been elucidated. To date, several mechanisms such as somatic and visceral sensory impairment due to neural injury, dysfunction of bowel movement control, and loss of voluntary control of the anal sphincter have been suggested to explain this phenomenon. Meshkinpour et al.10 reported a markedly reduced motility index in the distal colon after SCI. Fajardo et al.11 have reported that the baseline colonic activity significantly reduced after SCI, and they suggested that this alteration may be associated with the difficulty with evacuation. Although the colonic transit time after SCI was known to be prolonged primarily in the distal colon, the transit time in each colonic segment is still debatable. Such differences in the previously reported results have been attributed to the differences between animal models and humans,3,10 between complete and incomplete injury,7 and in neurological injury levels.12 Therefore, in order to identify whether colonic motility in the different colonic segments is different, we examined the change in contractility in response to Ach with and without M2 or M3 receptor antagonist pretreatment at 1 week after the SCI. Our results showed that compared with the control rats, the SCI rats exhibited a significant increase of contractility in the proximal colon. Compared with the control rats, the SCI rats showed a decreased contractility in the distal colon, but the decrease was not statistically significant.
The overall response to Ach in the tissues pretreated with AQ-RA741 was lower than the response observed in tissues not pretreated with AQ-RA741. Furthermore, compared to the control rats, the SCI rats showed a significant increase in contractility only in the proximal colon. In contrast, pretreatment with 4-DAMP resulted in a marked reduction in contractility after Ach treatment, and the intergroup difference in contractility of the proximal colon was not significant. Although after treatment with high concentrations of Ach, the contractility along the longitudinal muscle direction of the distal colon was reduced in both the groups, the reduction was significant only for the SCI group. These results indicate that compared to the distal colon, the proximal colon is more responsive to Ach after SCI.
To summarize, the experiments involving the use of receptor antagonists show that the intergroup difference in the response of the proximal colon to Ach was significantly different when tissues were pretreated with an M2 antagonist, whereas the intergroup differences in the response of the proximal colon to Ach was not significantly different, but the contractility in all muscle directions was reduced when the tissues were pretreated with an M3 antagonist. These results suggest that the change in Ach responsive after an SCI is due to the increase either in the response of M3 or in the number of receptors in the proximal colon.
Results of previous studies show that only M2 and M3 receptors are expressed in the colon of animals as well as of humans. In humans, the ratio of M2 and M3 receptors in the colon is 4 : 1 and a similar ratio is seen in the bladder.13,14 The role of each receptor in humans is still debatable. However, M3 receptors are reported to play a major role in the bowel movements in animals, and the colonic transit time decreases if these receptors are blocked.15 Similarly, these receptors play a major role in the bladder and the colon. Recently, the importance of the role of M2 receptor, particularly in the colon, where their contribution is more than that in the bladder, has been emphasized.16 After M3 antagonist pretreatment, the contractility along the longitudinal muscle direction in the distal colon reduced for both the groups, but the reduction was significant only for the SCI group. This result suggests that the major change in the distal colon may be the reduced response of the M2 receptors rather than that of the M3 receptors.
Taken together, our results show that after SCI, the increased contractility along both longitudinal and circular muscle directions in the proximal colon may be attributable to the increased response of the M3 receptors, whereas the reduced contractility in the distal colon may be attributable to the reduced response of the M2 receptors. In other words, SCI may lead to alterations in the number or activity of the M receptors in colonic smooth muscles, and this may change colonic motility. Therefore, to understand the mechanism of the alteration of colonic motility after an SCI, studies should investigate the changes in coordinated muscle movements and in inhibitory systems involving nitric oxide in addition to changes in M receptors.
HSP27, along with caldesmon, is associated with bowel contractility after an SCI and is involved in the opening and closing of the active sites where the myosin heads are attached during smooth muscle contraction. Previous studies have shown that p38 mitogen-activated protein (MAP) kinases induce phosphorylation of HSP27,17,18 and the increase in phosphorylated HSP27 is associated with the increase in contractility of the corresponding smooth muscles.19 In contrast, smooth muscle contraction is initiated by the phosphorylation of myosin light chain (MLC) and controlled by MLC kinase (MLCK), which is activated by the calcium-calmodulin complex. MLC phosphatase (MLCP) antagonizes MLCK, and MLCP levels are reduced by RhoA, protein kinase C, and smooth muscle phosphoprotein CPI-17.20 RhoA not only suppresses MLCP activity, but also colocalizes with the actin binding protein HSP27, and thus, is involved in contractility. In addition, RhoA plays a decisive role in Ca2+ sensitization. Through this mechanism, it maintains phosphorylated myosin and induces repeated and continuous smooth muscle contraction.20 Therefore, during an Ach response, both HSP27 and RhoA are associated with the increase and decrease in contractility of smooth muscles, and an increase in the level of these proteins plays an important role in the induction and maintenance of smooth muscle contraction.20 Our results showed a significant increase in the levels of HSP27 and RhoA in the proximal colon of the SCI rats than in the proximal colon of the control rats. This finding is in agreement with results of the organ bath study that showed a significant intergroup difference in the Ach response of the proximal segment of the colon.
PGP 9.5 is a well-known general neuronal marker and specifically reacts with neurons and the neuroendocrine system.21 Recent studies have shown that PGP 9.5-postive immunoreactive cells are widely distributed throughout the lower gastrointestinal tract, and their density in the rectum, and the distal, transverse, and proximal colon is not different.22 A previous study showed that PGP 9.5 level was elevated in an overactive bladder that developed after SCI.23 However, studies on modulation of PGP 9.5 level in the colon have been rarely reported. Although we observed an increase in the levels of proteins associated with contractility in the proximal colon, the PGP 9.5 level, which reflects the increase or decrease of innervation, was significantly decreased in both the proximal and distal colon. This finding is consistent with that of previous studies showing reduced PGP 9.5 levels in the peripheral tissues after nerve injuries,24 and this may be because of the blockage of neurotransmission from the regions anterior to the SCI.
After Ach treatment, the contractility along both the longitudinal and circular muscle directions in the proximal colon of the SCI rats was significantly higher than that in the proximal colon of the control rats. When Ach treatment was conducted after AQ-RA741 pretreatment, the contractility along the longitudinal and circular muscle direction in the proximal colon of the SCI rats showed a significant increase. When Ach treatment was conducted after 4-DAMP pretreatment, only the contractility along the longitudinal muscle direction in the distal colon of the control rats significantly increased. Furthermore, the levels of the contraction-associated proteins in the proximal colon increased, and the alterations in colonic motility were associated with changes in the M receptor subtypes. Such alterations were associated with the increase of contractility in the proximal colon rather than in the distal colon. Moreover, we believe that the decrease in the Ach response of the distal colon partially contributed to the reduced contractility. To understand the mechanism of reduced contractility in the distal colon, additional studies should be conducted on the modulation of proteins associated with contraction or alteration of excitatory or inhibitory neurotransmitters or receptors that are associated with bowel contractility.
ACKNOWLEDGEMENTS
This work was supported by the Korea Research Foundation Grant funded by the Korean Government (KRF-2008-521-E00100).
References
1. Glickman S, Kamm MA. Bowel dysfunction in spinalcord-injury patients. Lancet. 1996; 347:1651–1653. PMID: 8642958.


2. Longo WE, Ballantyne GH, Modlin IM. The colon, anorectum and spinal cord patient. A review of the functional alterations of the denervated hindgut. Dis Colon Rectum. 1989; 32:261–267. PMID: 2646085.
3. Menardo G, Bausano G, Corazziari E, Fazio A, Marangi A, Genta V, Marenco G. Large-bowel transit in paraplegic patients. Dis Colon Rectum. 1987; 30:924–928. PMID: 3691262.


4. Im SH, Rah UW, Lee IY, Cho KH. Colon transit time and management of upper motor neuron type neurogenic bowel in spinal cord injury. J Korean Acad Rehabil Med. 2000; 24:446–452.
5. Beuret-Blanquart F, Weber J, Gouvemeur JP, Demangeon S, Denis P. Colon transit time and anorectal manometric anomalies in 19 patients with complete transection of the spinal cord. J Auton Nerv Syst. 1990; 30:199–207. PMID: 2229888.
6. Zhang LB, Horowitz B, Buxton IL. Muscarinic receptors in canine colonic circular smooth muscle. I. Coexistence of M2 and M3 subtypes. Mol Pharmacol. 1991; 40:943–951. PMID: 1758444.
7. Uchiyama T, Chess-Williams R. Muscarinic receptor subtypes of the bladder and gastrointestinal tract. J Smooth Muscle Res. 2004; 40:237–247. PMID: 15725706.


8. Tong YC, Chin WT, Cheng JT. Alterations in urinary bladder M2-muscarinic receptor protein and mRNA in 2-week streptozotocin-induced diabetic rats. Neurosci Lett. 1999; 277:173–176. PMID: 10626841.


9. Braverman AS, Ruggieri MR Sr. Hypertrophy changes the muscarinic receptor subtype mediating bladder contraction from M3 toward M2. Am J Physiol Regul Integr Comp Physiol. 2003; 285:R701–R708. PMID: 12763741.
10. Meshkinpour H, Harmon D, Thompson R, Yu J. Effects of thoracic spinal cord transection on colonic motor activity in rats. Paraplegia. 1985; 23:272–276. PMID: 4069737.


11. Fajardo NR, Pasiliao RV, Modeste-Duncan R, Creasey G, Bauman WA, Korsten MA. Decreased colonic motility in persons with chronic spinal cord injury. Am J Gastroenterol. 2003; 98:128–134. PMID: 12526948.


12. Lim SS, Choi KH, Myung SJ, Sung IY. Evaluation of the neurogenic bowel by colon transit time and anorectal manometry in the spinal cord injured patients. J Korean Acad Rehabil Med. 2001; 25:249–255.
13. Gómez A, Martos F, Bellido I, Marquez E, Garcia AJ, Pavia J, Cuesta FS. Muscarinic receptor subtypes in human and rat colon smooth muscle. Biochem Pharmacol. 1992; 43:2413–2419. PMID: 1610405.


14. Kerr PM, Hillier K, Wallis RM, Garland CJ. Charactrization of muscarinic receptors mediating contractions of circular and longitudinal muscle of human isolated colon. Br J Pharmacol. 1995; 115:1518–1524. PMID: 8564213.
15. Matsui M, Motomura D, Fujikawa T, Jiang J, Takahashi S, Manabe T, Taketo MM. Mice lacking M2 and M3 muscarinic acetylcholine receptors are devoid of cholinergic smooth muscle contractions but still viable. J Neurosci. 2002; 22:10627–10632. PMID: 12486155.
16. Matsui M, Motomura D, Karasawa H, Fujikawa T, Jiang J, Komiya Y, Takahashi S, Taketo MM. Multiple functional defects in peripheral autonomic organs in mice lacking muscarinic acetylcholine receptor gene for the M3 subtype. Proc Natl Acad Sci USA. 2000; 97:9579–9584. PMID: 10944224.


17. Yamboliev IA, Hedges JC, Mutnick JL, Adam LP, Gerthoffer WT. Evidence for modulation of smooth muscle force by the p38 MAP kinase/HSP27 pathway. Am J Physiol Heart Circ Physiol. 2000; 278:H1899–H1907. PMID: 10843887.
18. Somara S, Bitar KN. Phosphorylated HSP27 modulates the association of phosphorylated caldesmon with tropomyosin in colonic smooth muscle. Am J Physiol Gastrointest Liver Physiol. 2006; 291:G630–G639. PMID: 16627824.


19. Patil SB, Tsunoda Y, Pawar MD, Bitar KN. Translocation and association of ROCK-II with RhoA and HSP27 during contraction of rabbit colon smooth muscle cells. Biochem Biophys Res Commun. 2004; 319:95–102. PMID: 15158447.


20. Patil SB, Pawar MD, Bitar KN. Phosphorylated HSP27 essential for acetylcholine-induced association of RhoA with PKCalpha. Am J Physiol Gastrointest Liver Physiol. 2004; 286:G635–G644. PMID: 14592945.
21. Kent C, Clarke PJ. The immunolocalisation of the neuro endocrine specific protein PGP9.5 during neurogenesis in the rat. Dev Brain Res. 1991; 58:147–150. PMID: 1826643.
22. Matsumoto K, Hosoya T, Tashima K, Namiki T, Murayama T, Horie S. Distribution of transient receptor potential vanilloid 1 channel-expressing nerve fibers in mouse rectal and colonic enteric nervous system: relationship to peptidergic and nitrergic neurons. Neuroscience. 2011; 172:518–534. PMID: 20951772.


23. Brady CM, Apostolidis AN, Harper M, Yiangou Y, Becjett A, Jacques TS, Freeman A, Scaravilli F, Fowler CJ, Anand P. Paraller changes in bladder suburothelial vanilloid receptor TRPV1 and pan-neural marker PGP9.5 immunoreactivity in patients with neurogenic detrusor overactivity after intravesical resiniferatoxin treatment. BJU Int. 2004; 93:770–776. PMID: 15049988.
24. Oaklander AL, Brown JM. Unilateral nerve injury produces bilateral loss of distal innervation. Ann Neurol. 2004; 55:639–644. PMID: 15122703.


Fig. 1
Photographs of the colon of rats belonging to both groups. The upper and lower panels show the colons of the control (CON) spinal cord injured (SCI) rats, respectively. Note the fecal impaction in the distal colon of the SCI rats.
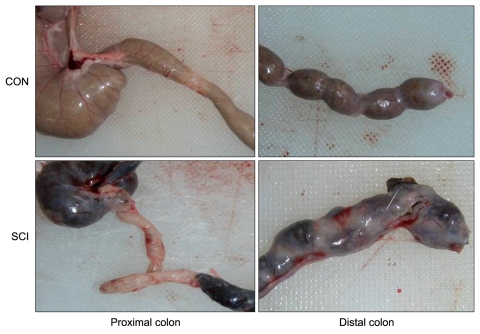
Fig. 2
Percentage changes in contractility after treatment with acetylcholine (Ach) for the control (CON) and spinal cord injury (SCI) groups. The intergroup difference in the percentage changes in contractility in the proximal colon along the longitudinal and circular muscle direction was significant. The values are expressed as mean±SD. *Denotes significant difference between the CON and SCI groups (*p<0.05).
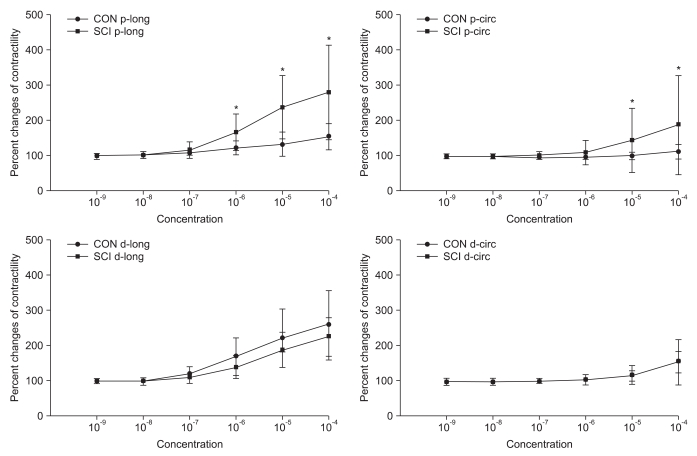
Fig. 3
Percentage changes in contractility of the proximal and distal colon of the control (CON) and spinal cord injury (SCI) groups after acetylcholine (Ach) treatment of samples pretreated with AQ-RA741. The intergroup difference in the percentage changes in contractility in the proximal colon along the longitudinal and circular muscle direction was significant. The values are expressed as mean±SD. *Denotes significant difference between the CON and SCI groups (*p<0.05).
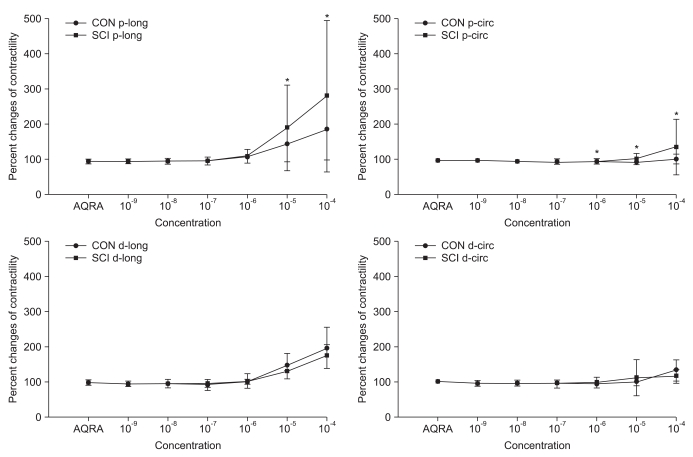
Fig. 4
Percentage changes in contractility of the proximal and distal colon of the CON and SCI groups after Ach treatment of samples pretreated with 4-DAMP. The intergroup difference in the percentage changes in contractility in the distal colon along the longitudinal muscle direction was significant. The values are expressed as mean±SD. *Denotes significant difference between the CON and SCI groups (*p<0.05).
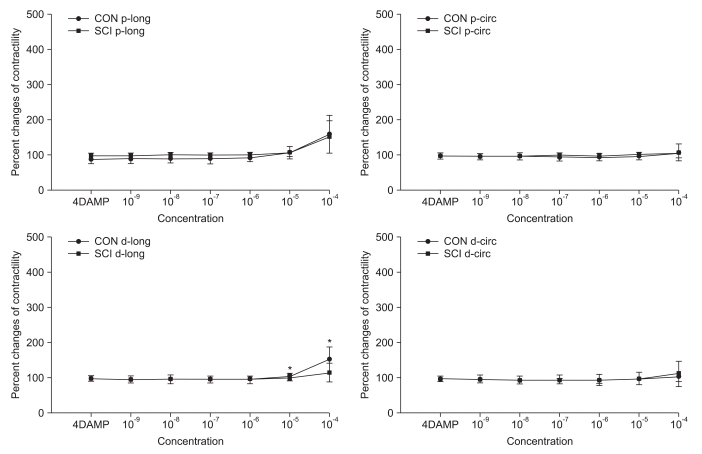
Fig. 5
Results of western blot analysis for PGP 9.5 (B), RhoA (C), and HSP 27 (D) in the proximal and distal colon. (A) shows representative cases of each protein. The intergroup differences in the levels of PGP 9.5 in the proximal and distal colon and of RhoA and HSP 27 in the proximal colon were significant. The values are as expressed mean±SD. *Denotes significant difference between the CON and SCI groups (*p<0.05).
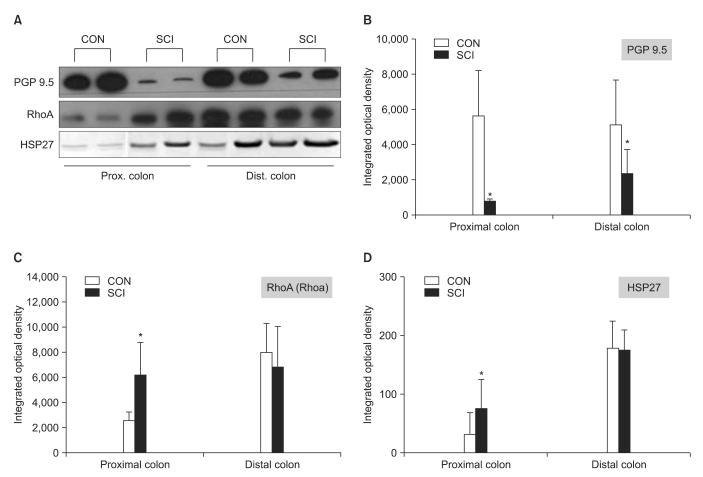