Abstract
Purpose
Microbiota manipulation through selected probiotics may be a promising tool to prevent cancer development as well as onset, to improve clinical efficacy for cancer treatments. The purpose of this study was to evaluate change in microbiota composition after-probiotics supplementation and assessed the efficacy of probiotics in improving quality of life (QOL) in postoperative cancer patients.
Methods
Stool samples were collected from 30 cancer patients from February to October 2020 before (group I) and after (group II) 8 weeks of probiotics supplementation. We performed 16S ribosomal RNA gene sequencing to evaluate differences in gut microbiota between groups by comparing gut microbiota diversity, overall composition, and taxonomic signature abundance. The health-related QOL was evaluated through the EORTC Quality of life Questionnaire Core 30 questionnaire.
Results
Statistically significant differences were noted in group II; increase of Shannon and Simpson index (P = 0.004 and P = 0.001), decrease of Bacteroidetes and Fusobacteria at the phylum level (P = 0.032 and P = 0.014, retrospectively), increased of beneficial bacteria such as Weissella (0.096% vs. 0.361%, P < 0.004), Lactococcus (0.023% vs. 0.16%, P < 0.001), and Catenibacterium (0.0% vs. 0.005%, P < 0.042) at the genus level. There was a significant improvement in sleep disturbance (P = 0.039) in group II.
Conclusion
Gut microbiota in cancer patients can be manipulated by specific probiotic strains, result in an altered microbiota. Microbiota modulation by probiotics can be considered as part of a supplement that helps to increase gut microbiota diversity and improve QOL in cancer patients after surgery.
The gut microbiota has been considered to be an essential organ, carrying approximately 150 times more genes than that found in the entire human genome [1]. The gut microbiota has emerged as a critical player in the maintenance of human health, influencing not only the gastrointestinal tract but also distal organs such as the brain, liver, and pancreas [2]. With the increased understanding of the relationship between the human microbiota and a variety of diseases, the use of these findings to predict or diagnose diseases had attracted a great deal of attention. As such, dysbiosis, which refers to compositional and functional alterations of the gut microbiota, contributes to the development of various pathologic conditions including cancer [3]. The gut microbiota has been implicated in cancer and shown to modulate the efficacy of anticancer treatments. The microbiota composition and function differ according to location, age, sex, race, and diet of the host. Over the past 10 years, combining next-generation sequencing with computational analysis of the 16S ribosomal RNA (rRNA) amplicons has allowed the characterization of both the diversity and abundance of the gut microbiota.
Probiotics are defined as “microorganisms for which there is scientific evidence of safety and efficacy” and excluding “live cultures associated with fermented foods for which there is no evidence of a health benefit” [4]. Probiotics have been proposed as preventive and therapeutic measures used to restore the healthy composition and function of the gut microbiota. Probiotics have been proven to modulate gut microbiota composition imbalances by increasing bacterial populations, gut epithelium barrier function, and cytokine production. The microbial community composition is more stable during the period of probiotic treatment [5]. However recent studies demonstrated the association of microbiota alterations following treatment with probiotics [6]. The beneficial effect of probiotics depends upon the probiotic strains. Lactobacillus and Bifidobacterium are the most widely used strains for safety and efficacy. The combination of 2 or more probiotics has an effect superior to that of a single probiotic strain [7].
Microbiota manipulation through selected probiotics may be a promising tool to prevent cancer onset, improve the clinical efficacy of anticancer treatments, and mitigate the adverse effects of standard anticancer therapy. Most of these benefits are achieved through the modulation of host immunity and the inflammatory response [8]. Probiotics were effective in alleviating postoperative adverse symptoms by modifying the gut microbiota of cancer surgery patients [9]. Therefore, understanding the role of gut microbiota contributes to improving the care of cancer surgery patients. However, the postoperative clinical benefit of maintaining the gut microbiota balance remains largely unexplored and unknown [10]. The purpose of this study was to evaluate the changes in gut microbiota composition and assess the efficacy of probiotics in improving the quality of life (QOL) of cancer patients.
Thirty-four cancer surgery patients (21 colorectal cancer [CRC], 10 breast cancer, 2 gastric cancer, and 1 pancreatic cancer patient) who visited Seoul Song Do Colorectal Hospital in Seoul, Korea between February and October 2020 were recruited. Patients who are pregnant or lactating; metabolic syndrome, liver disease, diabetes mellitus, or autoimmune disease were excluded. Also, those who had used antibiotics or consumed lactic acid bacteria-related agents such as probiotics, prebiotics, and synbiotics within the last 2 weeks were excluded from the study. During the 8 weeks of probiotic administration, a total of 4 participants dropped out. Two participants experienced severe side effects from chemotherapy, 1 participant had newly developed severe constipation, and 1 participant failed to attend the follow-up visit. The results of 30 participants were used in the final data analysis. We classified the group before ingestion of probiotics (group I) and the group after ingestion of probiotics (group II).
All study procedures were performed in accordance with the Declaration of Helsinki. This study was approved by the Institutional Review Board of Seoul Song Do Colorectal Hospital (No. 2020-001). Written informed consent was obtained from all patients prior to enrollment.
The probiotic capsule contained approximately 5 × 109 colony-forming unit (CFU) of live freeze-dried bacteria (Ildong Bioscience Co. Ltd., Pyeongtaek, Korea) comprised of a mixture of 9 strains: Bifidobacterium breve IDCC4401, Bifidobacterium bifidum IDCC4201, Bifidobacterium longum IDCC4101, Bifidobacterium lactis IDCC4301, Lactobacillus rhamnosus IDCC3201, Lactobacillus acidophilus IDCC3302, Lactobacillus reuteri IDCC3701, Lactobacillus casei IDCC3451, and Lactococcus lactis IDCC2301. The capsules also contained prebiotics such as inulin, polysaccharides, zinc, selenium, 3 amino acids (glutamine, arginine, and histidine), vitamin B6, and Shiitake mushroom mycelium.
The symbiotic mixture used was double-coated with peptides and polysaccharides. This protective technology can protect the ingested bacteria from unfavorable conditions such as low pH, protease-rich conditions, and bile acid exposure. The participants took the 2 provided probiotics 30 minutes before a meal with water in the early morning for 8 weeks. We considered good compliance when the participants consumed more than 85% of the total products given.
We collected 2 samples from each participant. A fecal sample was collected prior to the administration of probiotics and 8 weeks later. The test kits containing a sterile tube (AccuGene, Incheon, Korea) to collect the stool samples were provided to the participants. The participants were asked to preserve the sample at room temperature until delivery to the laboratory. After delivery, stool specimens were immediately stored at −80℃ until metagenomic DNA extraction.
Approximately 200 mg of each stool sample was subsequently extracted using the commercial QIAamp Power Fecal Pro DNA Kit (Qiagen, Hilden, Germany) according to the manufacturer’s instructions. The concentration and quality of DNA were measured using an ultraviolet spectrophotometer (Eppendorf, Hamburg, Germany). The Qubit dsDNA HS Assay Kit (Invitrogen, Eugene, OR, USA) was used to determine the initial concentration of double-stranded (ds) DNA.
The Ion Torrent 16S Metagenomics Kit (Thermo Fisher Scientific, Pleasanton, CA, USA) was used to amplify 16S rRNA genes in all samples. Polymerase chain reaction (PCR) was performed with 2 primers according to the amplification conditions indicated in the manufacturer’s protocol. Primer 1 contained targets for the V2 (250 bp), V4 (288 bp), and V8 (295 bp) regions, whereas primer 2 contained the V3 (215 bp), V6–7 (260 bp), and V9 (209 bp) regions. After PCR amplification, the conjugated PCR products were purified according to the manufacturer’s instructions. DNA using the Ion Plus Fragment Library Kit (Thermo Fisher Scientific, Carlsbad, CA, USA) and Ion Xpress barcodes. The Ion Xpress Barcode Adapters 1-16 Kit (Thermo Fisher Scientific, Carlsbad, CA, USA) according to the manufacturer’s protocol was performed and the DNA library was purified. Each library was diluted to a concentration of 50 pM and pooled into equal volumes. The Ion 530 kit (Thermo Fisher Scientific) was used for the Ion Chef (Thermo Fisher Scientific) and Ion S5 XL platform (Thermo Fisher Scientific) workflows.
Data analysis was mapped in Ion Reporter software (Thermo Fisher Scientific) to 2 reference 16S rRNA databases (Greengenes ver. 13.5 and MicroSEQ ID ver. 3.0; Thermo Fisher Scientific). To cluster the sequences, operational taxonomic unit (OTU) analysis was performed using Ion Reporter software, which runs Quantitative Insights into Microbial Ecology (QIIME). The genus and species cutoff values were set at 97% and 99%, respectively.
Alpha and beta diversity data generated by the QIIME2 software (ver. 2020.08) were exported from the Ion Reporter software. For alpha diversity analysis, Chao1, the Shannon index, and the Simpson index were generated to analyze species diversity within the samples. All indices were nonparametric indexes that estimated the richness and diversity of the species. According to these 3 parameters, box plots were created based on the species level at 99% similarity. We also used the Wilcoxon rank-sum test for 2 sample groups used to identify the significant differences in alpha diversity. Data visualization was performed using R software ver. 4.0.2 (R Foundation for Statistical Computing, Vienna, Austria; https://www.R-project.org/) and the ggplot2 and ggpubr graphics packages for R.
The beta diversity analysis generated principal coordinates analysis (PCoA) plots for weighted UniFrac, unweighted UniFrac, Bray-Curtis, and Jaccard using the EMPeror graphical tool within QIIME2. Beta diversity reflects the compositional difference between samples in one certain group. Permutational multivariate analysis of variance (PERMANOVA) was used to determine significant differences in the bacterial structures.
Relative abundances were calculated by normalizing the OTU table of the raw counts provided by the Ion Reporter software. The taxa were clustered at the phylum, genus, and species levels. The nonparametric Mann-Whitney test was used to determine statistically significant differences in the relative abundances between the 2 groups in phylum, genus, and species compositions. Only bacteria representing more than 1% of the total taxa were presented on the graph and the rest were grouped as “others.”
The linear discriminant analysis effect size (LEfSe) with default parameters was used to identify species with significant differences between the groups [11]. The statistical method applied using LEfSe software (version 1.0, http://huttenhower.sph.harvard.edu/galaxy/) was the Kruskal-Wallis test (linear discriminant analysis [LDA] score > 2.0).
QOL was assessed by a nurse using the QOL questionnaire instrument designed by European Organization for Research and Treatment of Cancer (EORTC). We received permission from EORTC to use a Korean version of the Quality of life Questionnaire Core 30 (QLQ-C30) related to cancer patients. The patients were required to respond to this questionnaire before and 8 weeks after the administration of probiotics.
The clinical characteristics of the participants, type of surgery (Supplementary Table 1), and current status of patient (Supplementary Table 2) are shown in Table 1. Thirty cancer patients (19 colon cancers, 9 breast cancers, 1 gastric cancer, and 1 pancreatic cancer) participated in this study and all patients underwent cancer surgery within 5 years. The mean age of the participants was 54.9 ± 7.03 years and 27 patients (90.0%) were female. Among the 30 patients, 3 patients (10.0%) were stage I, 10 patients (33.3%) were stage II, 13 patients (43.3%) were stage III, and 4 patients (13.3%) were stage IV. Twelve patients received chemotherapy and 4 patients received radiation therapy.
16S rRNA gene sequencing was performed on 60 stool samples to investigate the alterations in gut microbiota in cancer patients before and after the ingestion of probiotics. The amplicons were sequenced with a total of 18,604,826 reads and after filtering, an average of 57,108 ± 15,634 reads per sample was obtained.
Alpha diversity was estimated by Chao1, and the Shannon and Simpson indices to assess the difference in diversity between the 2 groups. These indices were used to reflect the diversity within the samples, including species richness, abundance, and evenness. No significant differences in the Chao1 index were identified between the 2 groups in the species richness estimate. The Shannon and Simpson indices were significantly increased in the after-probiotics groups (P < 0.01 and P < 0.001) compared to the before-probiotics group (Wilcoxon rank-sum test) (Fig. 1). These results suggest that the intake of a variety of bacteria changed the diversity of the gut microbiota.
To assess beta diversity, PERMANOVA was conducted to determine which variables significantly affected the overall composition of the gut microbiota. PCoA based on 4 metrics (weighted UniFrac, unweighted UniFrac, Bray-Curtis, and Jaccard) was performed to visualize the relationship between the microbiota communities in the 2 groups. PERMANOVA showed no significant difference between the 2 groups across all 4 metrics (P > 0.05) (Fig. 2).
The relative abundance of the dominant taxa at the phylum level in both groups was assessed by microbial taxonomic assignment. In each group of samples, we observed a difference in the gut microbiota (Fig. 3A). Over 98% of the bacteria in the samples belonged to 4 dominant phyla (Bacteroidetes, Firmicutes, Actinobacteria, and Proteobacteria). According to a comparative analysis of the relative abundances at different phylum levels, Bacteroidetes and Fusobacteria showed statistically significant decreases in group II (P = 0.032 and P = 0.014, retrospectively) (Table 2).
Before probiotic administration, the ratio of Firmicutes to Bacteroidetes was similar in the 2 groups. However, after probiotic administration, Bacteroidetes decreased and Firmicutes increased. The Firmicutes/Bacteroidetes (F/B) ratio was significantly decreased in group II.
At the genus level (Fig. 3B), the abundance of 3 taxa were increased (Table 2). At the genus level, Weissella (0.096% vs. 0.361%, P = 0.004), Lactococcus (0.023% vs. 0.16%, P < 0.001), and Catenibacterium (0.0% vs. 0.005%, P = 0.042) were significantly more abundant in group II than in group I.
At the species level (Fig. 3C), Blauta producta (0.55% vs. 0.053%, P = 0.045), Clostridium butyricum (0.006% vs. 0%, P = 0.022), and Veillonella dispar (0.09% vs. 0.078%, P = 0.033) significantly decreased in group II. However, Weissella cibaria (0.139% vs. 0.245%, P = 0.002), B. breve (0.000% vs. 0.013%, P = 0.001), and Lactobacillus garvieae (0.005% vs. 0.024%, P = 0.018) were significantly more abundant in group II than in group I.
To identify the distinguishing taxa of the gut microbiota within the 2 groups, the LEfSe method was implemented. LEfSe analysis revealed significant differences (LDA score > 2.0, P < 0.05) between the 2 groups in 10 OTUs (Fig. 4). At the phylum level, Bacteroidetes was significantly enriched in group I (Fig. 4B). At the genus level, 2 taxa exhibited significantly higher abundances in group II compared to group I, including Weissella and Lactococcus.
The QOL parts of the questionnaires in the EORTC QLQ-C30 were merged into several scales, as shown in Table 3. Data were available for only 30 of the 34 patients because of dropped the study. The QOL after 8 weeks did not differ between the 2 groups with the exception of scales related to sleep disturbances (P = 0.037).
In the present study, we demonstrated that a proportion of the strains administered as probiotics significantly increased in the fecal microbiota. Our study showed changes in the diversity and composition of the fecal microbiota after cancer patients took probiotics. First of all, probiotics are an area of rare safe and undesirable side effects for these particular patients. These findings were supported by a meta-analysis study on probiotic safety involving 2,242 cancer patients [12]. Moreover, no deaths related to probiotics have been reported, indicating that probiotics are safe for cancer patients.
We performed several comparative analyses between the before and after probiotic ingestion groups. It is generally believed that probiotics affect the diversity and composition of gut microbiota. In alpha diversity analysis, Chao1 showed no significant difference in species abundance between the 2 groups (P > 0.05). In contrast, the Shannon index and Simpson index of group II were significantly increased, indicating that the consumption of probiotics had an effect on the abundance and evenness of species. The gut microbiota in postsurgery CRC patients had a lower alpha diversity and a looser ecological interaction network result in the condition for postsurgery CRC patients such as anterior resection syndrome or bowel dysfunction [1314]. A diverse microbial community is a key feature of healthy intestinal microbiota and probiotics might have increased the diversity in our study. Recent evidence suggests that it may also influence the efficacy and/or minimize the adverse effects of chemotherapy, radiotherapy, and antibiotics [1516].
Generally, the gut microbiota is dominated by Firmicutes or Bacteroidetes, whereas Proteobacteria, Actinobacteria, Verrucomicrobia, and Fusobacteria are less abundant at the phyla level. Our data showed that the level of Bacteroidetes and Fusobacteria was markedly decreased in group II. The F/B ratio has been associated with maintaining homeostasis, and changes in this ratio can lead to various pathologies. The F/B ratio was also found to be lower in elderly subjects (65 years and older, n = 161) compared to young adults (28–46 years, n = 9) in the study by Claesson et al. [17]. It has been speculated that such changes in microbiota composition may reflect the gradual decline in organ function and ability to maintain barrier integrity in elderly individuals [18]. In the study by Biagi et al. [19], the F/B ratio was estimated at 3.6, 5.1, and 3.9 in young adults (25–40 years, n = 20), elderly people (63–76 years, n = 22), and centenarians (99–104 years, n = 21), respectively. In the present study, we found that the F/B ratio in group II (3.6) was higher than that in group I (2.5), suggesting that taking probiotics could reduce potential disease risks.
The ingestion of probiotics can contribute to the restoration of dysbiotic gut microbiota and the prevention of cancer. Fusobacterium, in particular, is one of the most important pathogens reported to be closely associated with CRC. Increasing evidence has shown its roles in the carcinogenesis, diagnosis, progression, and prognosis of CRC [20]. Specifically, the overabundance of invasive Fusobacterium has been suggested to negatively impact patient outcomes. Fusobacterium species can promote host proinflammatory responses and possess virulence characteristics that promote their adhesiveness to host epithelial cells and their ability to invade epithelial cells [21]. Our study suggested that Fusobacterium tended to decrease due to the probiotic effect in cancer patients.
In the current study, changes in the microbiota composition at the genus level were more apparent than those at the phylum level. Our results provide evidence that probiotic strains affected the composition of gut microbiota at the genus and species levels. Recently, specific Weissella strains have received attention as potential probiotics, and the strain development, particularly of W. cibaria strains, is receiving attention because of their high probiotic potential for controlling periodontal disease [22]. Lactococcus is a gram-positive bacterium used extensively in the production of buttermilk and cheese. However, it has also become famous as the first genetically modified organism to be used live for the treatment of human disease. Reports showed that lactic acid production had immunomodulatory, anticancer, and anti-inflammatory activities and beneficial effects on the skin [23]. Another genus enriched in the probiotic group, Catenibacterium species, produces short-chain fatty acids, such as butyric acids, from glucose fermentation [24]. Butyric acid may serve beneficial roles in colonic anti-inflammation and metabolic health. Our findings showed probiotic interventions that favored the expansion of beneficial microorganisms acting either on endogenous bacteria or the administered probiotics at the genus level.
Probiotics contain the right strain, the right amount, the right formulation, and right conditions for the intended use. Like the lactic acid bacteria used in our study, synbiotics (combination of probiotics and prebiotics) and the combination of 2 or more probiotics had an effect superior to that of a single probiotic strain [25]. Most probiotic products today are developed with Bifidobacterium, Lactobacillus, and other lactic acid bacteria, such as Lactococcus and Streptococcus. Other promising strains include bacteria genera, such as Bacillus, Escherichia, and Propionibacterium, and some other yeast genera, mainly Saccharomyces. The probiotics used in our study were composed of 4 strains of Bifidobacterium, 4 strains of Lactobacillus, and 1 strain of Lactococcus. Each capsule contained approximately 5 × 109 CFU of live freeze-dried bacteria. The capsules also contained inulin, polysaccharides, zinc, selenium, 3 amino acids (glutamine, arginine, and histidine), vitamin B6, and Shiitake mushroom mycelium. In fact, the principal aim of administrating probiotics to cancer patients is to repopulate Lactobacillus in the gut microbiota of compromised patients, thus reestablishing the levels and functionality of the commensal bacteria depleted after treatment [26]. The randomized administration of B. lactis and L. acidophilus to CRC patients changed the epigenetic patterns of tumor tissue from baseline, with potential therapeutic benefits in CRC by manipulation of the gut microbiota [25]. The preparation or composition of probiotic products might also be factors that contribute to the different effects. However, guidelines regarding the strain, duration, and dosage of probiotics for cancer patients are not clear.
Our study focused on the QOL in cancer patients after the ingestion of probiotics using the EORTC QOL-C30 as the QOL score. Although the QOL in cancer patients might be an important endpoint, few clinical research studies have evaluated this endpoint because depression, anxiety, chronic fatigue, and sleep disturbance are common symptoms in cancer patients that negatively affect the QOL. Gut microbes may communicate through the gut-brain axis via the production of neuroactive and neuroendocrine molecules such as serotonin, gamma-aminobutyric acid (GABA), histamine, noradrenaline, and adrenaline [27]. A recent triple-blind, randomized placebo-controlled study showed reduced cognitive reactivity to sad moods after a 4-week intervention with multispecies probiotics [28]. Takada et al. [29] reported an improvement in sleep quality in academic students during a period of increasing stress after 11 weeks of probiotic consumption. Overall, multispecies probiotic intake was noted to exert a positive effect on depressive mood state and sleep quality [30]. Our findings extend previous observations, suggesting that the probiotic mixture we used was effective in improving sleep disturbances in cancer patients. The precise role of probiotics in cancer-related mental problems should be investigated in the future.
The results of this study supported the hypothesis that the gut microbiota of cancer patients can be manipulated by specific probiotic strains, resulting in altered gut microbiota enriched with beneficial bacteria. Our study provides evidence that the regulation of gut microbiota by probiotics can be considered as part of a supplement that helps increase gut microbiota diversity and improve the QOL after surgery in cancer patients. However, our study was conducted at a single center and was limited by the number of patients enrolled. Therefore, multicenter randomized controlled trials including multimodal probiotics may provide more clinical evidence supporting the positive effects of probiotics in cancer patients.
References
1. Ursell LK, Haiser HJ, Van Treuren W, Garg N, Reddivari L, Vanamala J, et al. The intestinal metabolome: an intersection between microbiota and host. Gastroenterology. 2014; 146:1470–1476. PMID: 24631493.


2. Cheng WY, Wu CY, Yu J. The role of gut microbiota in cancer treatment: friend or foe? Gut. 2020; 69:1867–1876. PMID: 32759302.


3. Schwabe RF, Jobin C. The microbiome and cancer. Nat Rev Cancer. 2013; 13:800–812. PMID: 24132111.


4. Food and Agriculture Organization of the United Nations (FAO). World Health Organization (WHO). Health and nutritional properties of probiotics in food including powder milk with live lactic acid bacteria. Report of a Joint FAO/WHO Expert Consultation on evaluation of health and nutritional properties of probiotics in food including powder milk with live lactic acid bacteria: 2001 Oct 1-4; Córdoba, Argentina. FAO & WHO;2001.
5. Cha BK, Jung SM, Choi CH, Song ID, Lee HW, Kim HJ, et al. The effect of a multispecies probiotic mixture on the symptoms and fecal microbiota in diarrhea-dominant irritable bowel syndrome: a randomized, double-blind, placebo-controlled trial. J Clin Gastroenterol. 2012; 46:220–227. PMID: 22157240.
6. Hemarajata P, Versalovic J. Effects of probiotics on gut microbiota: mechanisms of intest inal immunomodulation and neuromodulation. Therap Adv Gastroenterol. 2013; 6:39–51.
7. Chapman CM, Gibson GR, Rowland I. Health benefits of probiotics: are mixtures more effective than single strains? Eur J Nutr. 2011; 50:1–17. PMID: 21229254.


8. van Heinsbergen M, Janssen-Heijnen ML, Leijtens JW, Slooter GD, Konsten JL. Bowel dysfunction after sigmoid resection underestimated: multicentre study on quality of life after surgery for carcinoma of the rectum and sigmoid. Eur J Surg Oncol. 2018; 44:1261–1267. PMID: 29778617.


9. Li W, Dowd SE, Scurlock B, Acosta-Martinez V, Lyte M. Memory and learning behavior in mice is temporally associated with diet-induced alterations in gut bacteria. Physiol Behav. 2009; 96:557–567. PMID: 19135464.


10. Peitsidou K, Karantanos T, Theodoropoulos GE. Probiotics, prebiotics, synbiotics: is there enough evidence to support their use in colorectal cancer surgery? Dig Surg. 2012; 29:426–438. PMID: 23258276.


11. Segata N, Izard J, Waldron L, Gevers D, Miropolsky L, Garrett WS, et al. Metagenomic biomarker discovery and explanation. Genome Biol. 2011; 12:R60. PMID: 21702898.


12. Hassan H, Rompola M, Glaser AW, Kinsey SE, Phillips RS. Systematic review and meta-analysis investigating the efficacy and safety of probiotics in people with cancer. Support Care Cancer. 2018; 26:2503–2509. PMID: 29704110.


13. Cong J, Zhu H, Liu D, Li T, Zhang C, Zhu J, et al. A pilot study: changes of gut microbiota in post-surgery colorectal cancer patients. Front Microbiol. 2018; 9:2777. PMID: 30515141.


14. Park SS, Kim B, Kim MJ, Roh SJ, Park SC, Kim BC, et al. The effect of curative resection on fecal microbiota in patients with colorectal cancer: a prospective pilot study. Ann Surg Treat Res. 2020; 99:44–51. PMID: 32676481.


15. Taur Y, Jenq RR, Perales MA, Littmann ER, Morjaria S, Ling L, et al. The effects of intestinal tract bacterial diversity on mortalit y fol lowing al logeneic hematopoietic stem cell transplantation. Blood. 2014; 124:1174–1182. PMID: 24939656.
16. Delia P, Sansotta G, Donato V, Frosina P, Messina G, De Renzis C, et al. Use of probiotics for prevention of radiation-induced diarrhea. World J Gastroenterol. 2007; 13:912–915. PMID: 17352022.


17. Claesson MJ, Cusack S, O'Sullivan O, Greene-Diniz R, de Weerd H, Flannery E, et al. Composition, variability, and temporal stability of the intestinal microbiota of the elderly. Proc Natl Acad Sci U S A. 2011; 108(Suppl 1):4586–4591. PMID: 20571116.


18. Kundu P, Blacher E, Elinav E, Pettersson S. Our gut microbiome: the evolving inner self. Cell. 2017; 171:1481–1493. PMID: 29245010.


19. Biagi E, Nylund L, Candela M, Ostan R, Bucci L, Pini E, et al. Through ageing, and beyond: gut microbiota and inflammatory status in seniors and centenarians. PLoS One. 2010; 5:e10667. PMID: 20498852.


20. Rubinstein MR, Wang X, Liu W, Hao Y, Cai G, Han YW. Fusobacterium nucleatum promotes colorectal carcinogenesis by modulating E-cadherin/β-catenin signaling via its FadA adhesin. Cell Host Microbe. 2013; 14:195–206. PMID: 23954158.


21. Castellarin M, Warren RL, Freeman JD, Dreolini L, Krzywinski M, Strauss J, et al. Fusobacterium nucleatum infection is prevalent in human colorectal carcinoma. Genome Res. 2012; 22:299–306. PMID: 22009989.


22. Kwak SH, Cho YM, Noh GM, Om AS. Cancer preventive potential of Kimchi lactic acid bacteria (Weissella cibaria, Lactobacillus plantarum). J Cancer Prev. 2014; 19:253–258. PMID: 25574459.


23. Kanayama M, Kato Y, Tsuji T, Konoeda Y, Hashimoto A, Kanauchi O, et al. Enhancement of immunomodulative effect of lactic acid bacteria on plasmacytoid dendritic cells with sucrose palmitate. Sci Rep. 2018; 8:3147. PMID: 29453437.


24. Kageyama A, Benno Y. Catenibacterium mitsuokai gen. nov., sp. nov., a gram-positive anaerobic bacterium isolated from human faeces. Int J Syst Evol Microbiol. 2000; 50 Pt 4:1595–1599. PMID: 10939666.


25. Hibberd AA, Lyra A, Ouwehand AC, Rolny P, Lindegren H, Cedgård L, et al. Intestinal microbiota is altered in patients with colon cancer and modified by probiotic intervention. BMJ Open Gastroenterol. 2017; 4:e000145.


26. Zitvogel L, Ma Y, Raoult D, Kroemer G, Gajewski TF. The microbiome in cancer immunotherapy: diagnostic tools and therapeutic strategies. Science. 2018; 359:1366–1370. PMID: 29567708.


27. Rutsch A, Kantsjö JB, Ronchi F. The gut-brain axis: how microbiota and host inflammasome influence brain physiology and pathology. Front Immunol. 2020; 11:604179. PMID: 33362788.


28. Steenbergen L, Sellaro R, van Hemert S, Bosch JA, Colzato LS. A randomized controlled trial to test the effect of multispecies probiotics on cognitive reactivity to sad mood. Brain Behav Immun. 2015; 48:258–264. PMID: 25862297.


29. Takada M, Nishida K, Gondo Y, Kikuchi-Hayakawa H, Ishikawa H, Suda K, et al. Beneficial effects of Lactobacillus casei strain Shirota on academic stress-induced sleep disturbance in healthy adults: a double-blind, randomised, placebo-controlled trial. Benef Microbes. 2017; 8:153–162. PMID: 28443383.


30. Marotta A, Sarno E, Del Casale A, Pane M, Mogna L, Amoruso A, et al. Effects of probiotics on cognitive reactivity, mood, and sleep quality. Front Psychiatry. 2019; 10:164. PMID: 30971965.


SUPPLEMENTARY MATERIALS
Supplementary Tables 1 and 2 can be found via https://doi.org/10.4174/astr.2021.101.5.281.
Fig. 1
Alpha diversity. Boxplots of alpha diversity metrics; (A) Chao1, (B) Shannon index, and (C) Simpson index. Red bars and points indicate before group values; blue bars and points indicate after group values. Significance was assessed by means of paired Wilcoxon test. Group I, the group before ingestion of probiotics; group II, the group after ingestion of probiotics.
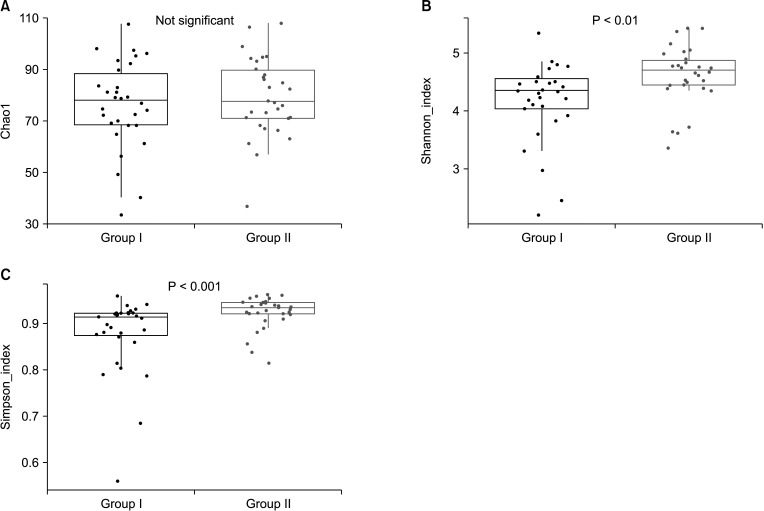
Fig. 2
Microbial comparison for beta diversity. Beta diversity based on (A) the weighted UniFrac distance, (B) the unweighted UniFrac distance, (C) Bray-Curtis distance, and (D) Jaccard distance were compared with principal coordinates analysis for the 2 groups. The groups were compared using the P-value of the permutational multivariate analysis of variance method.
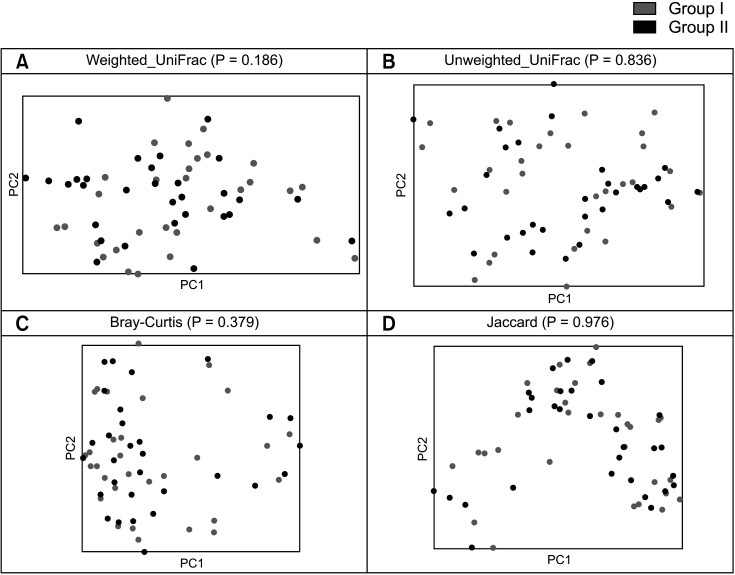
Fig. 3
Gut microbiota composition at the (A) phylum, (B) genus, and (C) species level for the 2 groups. *P < 0.05.
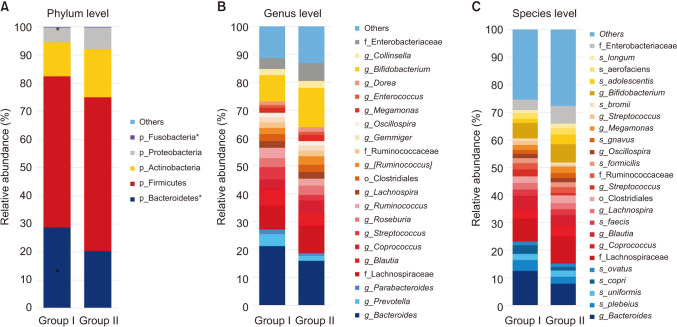
Fig. 4
The linear discriminant analysis effect size (LEfSe) analysis of gut microbiota between the 2 groups. (A) Cladogram generated by LEfSe indicating differences at phylum, class, family and genus levels between the 2 groups. Each successive circle represents a phylogenetic level. Regions in red indicate taxa enriched in before group affected while regions in green indicate taxa enriched in after group. Differing taxa are listed on the right side of the cladogram. (B) Bar graphs showing linear discriminant analysis (LDA) scores. Horizontal bars represent the effect size for each taxon. The length of the bar represents the log10 transformed LDA score, indicated by vertical dotted lines. Significance determined using default parameters (P < 0.05 by Kruskal-Wallis test and LDA score > 2). The name of the taxon level is abbreviated as p, phylum; c, class; g, genus; and s, species.
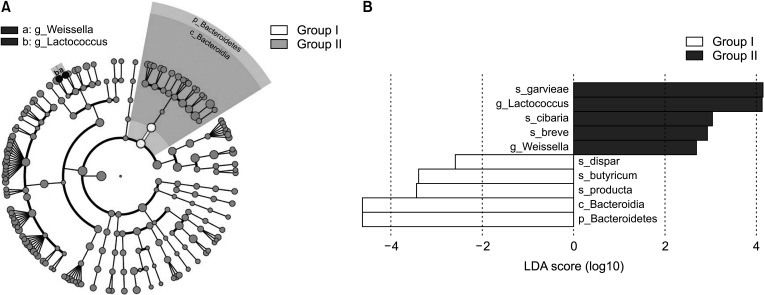