INTRODUCTION
Severe acute respiratory syndrome coronavirus 2 (SARS-CoV-2) has caused a pandemic of coronavirus disease 2019 (COVID-19), leading to a global health crisis and significant economic loss (1, 2). Several vaccines had been developed and are being distributed quickly, hoping COVID-19 pandemic ends in the near future. Effective and affordable anti-virals are still necessary for patients who are not vaccinated or where vaccines fail to prevent COVID-19. Currently, various therapeutic candidates against SARS-CoV-2 are being developed and are being treated to people (3, 4, 5, 6). Dexamethasone and Remdesivir are used for the treatment of SARS-CoV-2 (7). Dexamethasone is a corticosteroid drug with anti-inflammatory action. Dexamethasone is used as a steroid therapy to treat SARS-CoV-2 patients (8). Remdesivir is a viral RNA-dependent RNA polymerase inhibitor that has the ability to inhibit SARS-CoV-2 in vitro. Furthermore, patients treated with remdesivir recovered clinically faster than patients treated with placebo (9). Bamlanivimab and etesevimab were neutralizing monoclonal antibodies isolated from the serum of patients recovering from COVID-19 in the United States and China. In a recent study, the combination of bamlanivimab and etesevimab resulted in lower hospitalization and death rate than the placebo group (10). Although there are cases where drug repurposing and neutralizing monoclonal antibody have become therapeutic agents, a therapeutic agent for covid-19 derived from natural products is still being studied (11).
Plant extracts have been used as a medicine for a long time for various pharmacological purposes, including infectious diseases, and natural substances derived from plants can be safe and effective therapeutics for many diseases (12). Moreover, a number of scientific publications have encouraged the use of biological compounds derived from plants in the treatment and prophylaxis of diseases caused by microbial pathogens, including viruses. However, the investigations to develop therapeutics or prophylaxis for viral infection derived from plants are relatively limited in comparison with those for bacterial infection (13, 14). The medicinal or herbal plants, and their phytoconstituents, could be important sources for exploring novel alternative /supplementary treatments or prophylaxis for COVID-19. Recent studies demonstrated that various medicinal plants extracts, herbal extracts, and phytochemicals possess antiviral activities and can be used to prevent or treat COVID-19 (15, 16).
In order to investigate a possible antiviral activity, plant extracts are usually prepared by hot water, ethanol, or methanol extraction process. Here, we studied whether somatid natural gel (SNG), which is manufactured through the multistep process including mixing with water, heat treatment, aeration, centrifugation, and evaporation of garlic, mandarin fruit, and ginseng. Since SNG contains highly concentrated natural substances from the intermediate layer of centrifuged water/plant mixtures, it is possible that SNG has higher levels of bioactive materials compared to hot water prepared materials from the top layer. Joung O et al. demonstrated that a mixture of somatid from minerals and plant extracts possessed a potential to teat cervical cancer caused by human papilloma virus (HPV) (17). In the present study, we investigated whether SNG prepared from mandarin, garlic, or ginseng has antiviral effect on SARS-CoV-2 in Vero cell culture.
MATERIALS AND METHODS
Somatid natural gel (SNG) preparation
SNGs were prepared as described at Korean patent (KP) 10-1628995 and provided by Philsang Life Sciences Co., Ltd (18).
Vero cell culture and SARS-CoV-2 infection
Vero (African green monkey kidney epithelial) cells were maintained in Dulbecco’s Modified Eagle’s Medium 118 (DMEM) (Lonza, Basel, Switzerland) supplemented with 1% penicillin/streptomycin solution (Sigma-Aldrich, Saint Louis, USA) and 10% fetal bovine serum (FBS) (Gibco, Waltham, USA). SARS-CoV-2 was provided by National Culture Collection for Pathogens (NCCP). To infect Vero cells with SARS-CoV-2, cells were incubated with SARS-CoV-2 for 1 h at 37°C, in 5% CO2 incubator. Then, cell culture medium was removed and DMEM containing 2% FBS and 1% penicillin/streptomycin solution was added.
Plaque assay
Vero cells in 12-well plates were treated with cell culture supernatant diluted step-wise by 10-fold for 1 h at 37°C, in 5% CO2 incubator. After washing with PBS, cells were overlaid with DMEM with 0.5% agarose (genDEPOT, Texas, USA). Cells were fixed in 10% formaldehyde (Daejung, Siheung, Korea) for 10 min at 3 days post infection (dpi). Overlaid media was removed, and cells were stained with 0.4% crystal violet (Junsei, Tokyo, Japan) for 10 min. Then, visible plaques were counted.
Cytotoxicity assay
Mandarin, garlic, and ginseng SNGs were diluted to 10 mg/ml with DMEM and were filtered by 0.2 μm syringe filter (CORNING, New York, USA). Vero cells in 96-well plates were treated with SNGs at various concentrations for 72 h at 37°C, in 5% CO2 incubator. Cell cytotoxicity was evaluated by using EZ-cytox kit (Dogenbio, Seoul, Korea).
Cytopathic effect (CPE) inhibition assay
The assessment of the anti-SARS-CoV-2 activity of the SNG was based on the evaluation of the inhibition of virus induced cytopathic effect (CPE) in Vero cells. Vero cells were seeded into 96-well plates and infected with SARS-CoV-2 at multiplicity of infection (m.o.i) 0.01. After 1 h, cells were treated with SNGs at various concentrations for 72 h at 37°C, in 5% CO2 incubator. Cells were fixed in 10% formaldehyde for 10 min and stained with 0.4% crystal violet for 10 min. Crystal violet solution was removed and cells were washed with PBS. Crystal violet from remaining cells were eluted by methanol (Daejung, Siheung, Korea) and acetic acid (Daejung, Siheung, Korea) mixture (1:1) for 3 h at room temperature in a gentle rocker. Optical density was measured by spectrophotometer (BioTek, Winooski, USA) at 550 nm. The CPE inhibition percentages were measured using the following equation: T-VC/CC-VC, where T is the optical density (OD) of compound treated cells, VC is the OD of virus control, and CC is the OD of cell control (19).
Immunofluorescence assay (IFA)
Vero cells were cultured in an 8-well chamber slide and infected with SARS-CoV-2 at m.o.i 0.01 for 1 h. After the virus was removed, cells were treated with the mandarin SNG for 16 h at 37°C, in 5% CO2 incubator. Then, cells were fixed with a mixture of acetone and methanol for 10 min. Fixated cells were washed with PBS twice and treated with 5% Normal goat serum (Abcam, Cambridge, UK) for blocking non-specific binding sites for 1 h at RT (Blocking). After washing with PBS twice, cells were incubated with anti-SARS CoV-2 nucleocapsid protein antibody (primary antibody) (SinoBiological, Beijing, China) overnight at 4°C. Cells were stained with goat-anti-mouse 488 antibody (Jackson Laboratory, Bar Harbor, USA) for 1 h at RT. Cells were washed three times with buffer and mounted with Fluorescent Mounting Media (Dako, Glostrup, Denmark) and observed under fluorescence microscope (Olympus, Tokyo, Japan).
qRT-PCR
Viral RNA was extracted using the AccuPrep® Viral RNA Extraction Kit (Bioneer, Daejeon, Korea). Complementary DNA (cDNA) was synthesized by using Rever Tra ACE® qPCR RT Kit (TOYOBO, Osaka, Japan). qRT-PCR was performed using AccuPower® GreenStarTM RT-qPCR Master Mix (Bioneer, Daejeon, Korea). Viral RNA copy numbers were calculated using the SARS-CoV-2 RNA standard sample. Synthesized cDNA from viral RNA with a cDNA synthesis kit (TOYOBO, Osaka, Japan) were used to make standard samples. PCR amplification kit (Invitrogen, Waltham, USA) and PCR clean up kit (Promega, Madison, USA) were used for amplification and clean up. TOPspeed™ DNA Ligation kit (Promega, Madison, USA) was used for ligation, and transformation was performed using DH5-alpha competent cell (Enzynomics, Daejeon, Korea). The following primers were used: Receptor-Binding Domain (RBD) Forward: 5’-CAA TGG TTT AAC AGG CAC AGG-3’, RBD reverse: 5’-CTC AAG TGT CTG TGG ATC ACG-3’, RBD Forward for standard : 5’-GCT CCA TGG CCT AAT ATT ACA AAC TTG TGC C-3’, and RBD reverse for standard: 5’-TGC TCT AGA CTC AAG TGT CTG TGG ATC AC-3’ (20).
Flavonoids contents analysis
Flavonoids contents of mandarin SNG were analyzed at Jeju Special Self-Governing Province Agricultural Research and Extension Service as described previously (21).
RESULT
Cytotoxicity evaluation of mandarin, garlic, and ginseng SNGs
Cell viability was measured by performing MTT assay to evaluate the toxicity of mandarin, garlic, and ginseng SNGs. Three types of SNGs were diluted serially 10-fold from 10 mg/ml to 0.078 mg/ml and added into Vero cells. As shown in Fig. 1, mandarin and garlic SNGs did not show cytotoxicity at all concentrations. However, ginseng SNG showed over 20% cytotoxicity at 1.25, 2.5, 5, and 10 mg/ml (Fig. 1B).
Fig. 1
Cell viability of mandarin, garlic, and ginseng SNGs. Vero cells were cultivated in 96-well plate (flat). Three types of SNGs were diluted stepwise by 2-fold from 0.078 to 10 mg/ml and were treated in cells for 3 h. Values are expressed as Mean±SEM. The different superscripts denote differences at *p< 0.05, **p< 0.01 and ***p< 0.001.
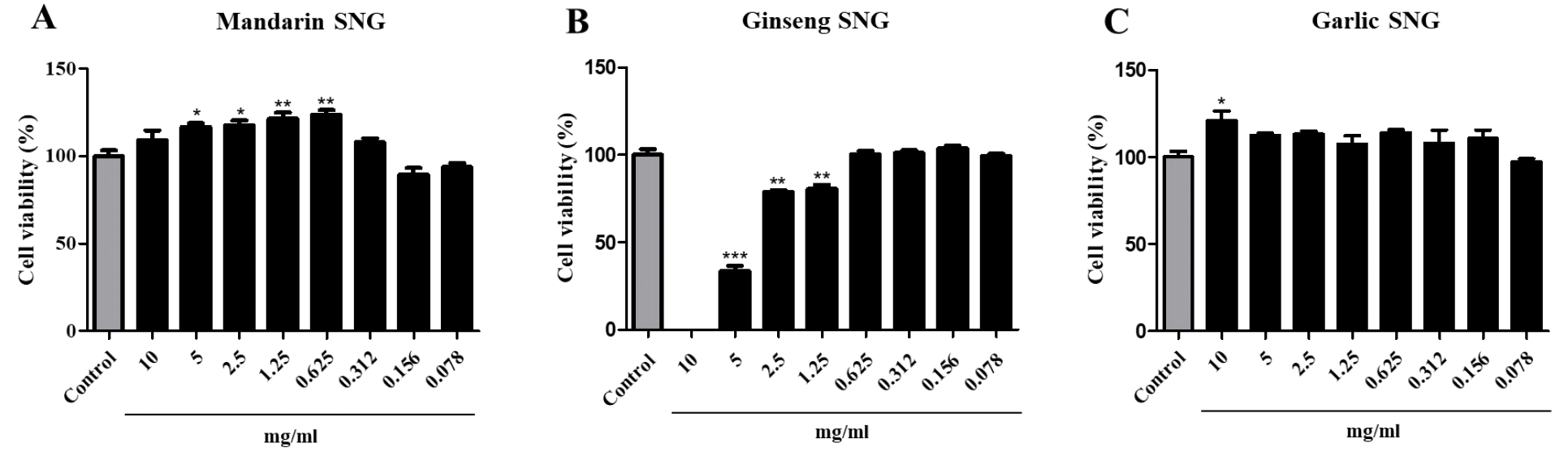
Mandarin SNG inhibits the CPE induced by SARS-CoV-2
CPE inhibition assay was performed to evaluate whether the three types of SNG inhibit SARS-CoV-2 replication in Vero cell cultures. Cells in 96 well plates were treated with mandarin or garlic SNG at concentrations ranging from 0.078 to 10 mg/ml and ginseng SNG at concentrations ranging from 0.078 to 2.5 mg/ml (Fig. 2). The mandarin SNG significantly inhibited CPE caused by SARS-CoV-2 replication at 0.312 mg/ml or higher (p< 0.05). Cells treated with 2.5 mg/ml or higher mandarin SNG showed an O.D. value similar to that of the control (Fig. 2-A2). In addition, ginseng SNG treatment suppressed SARS-CoV-2 replication at over 0.156 mg/ml (p< 0.05, Fig. 2-B2). However, it did not block 100%; because of cytotoxicity at 1.25 to 2.5 mg/ml, cell viability appears to be lower than that of 0.625 mg/ml treated group (Fig. 1B, Fig. 2-B2). Garlic SNG did not inhibit CPE by SARS-CoV-2 replication at all (Fig. 2-C2).
Fig. 2
Inhibitory effect of mandarin, ginseng, and garlic SNGs on CPE by SARS-CoV-2 replication. Infected Vero cells were incubated for 1 h with three types of SNGs of serially diluted concentration. Virus was treated with virus of m.o.i 0.01. After incubation for 72 h, cells were fixed in 10% formaldehyde and decolorized by Methanol/Acetic acid. Optical density was measured using a spectrophotometer. Values are expressed as Mean±SEM. The different superscripts denote differences at **p< 0.01, ***p< 0.001.
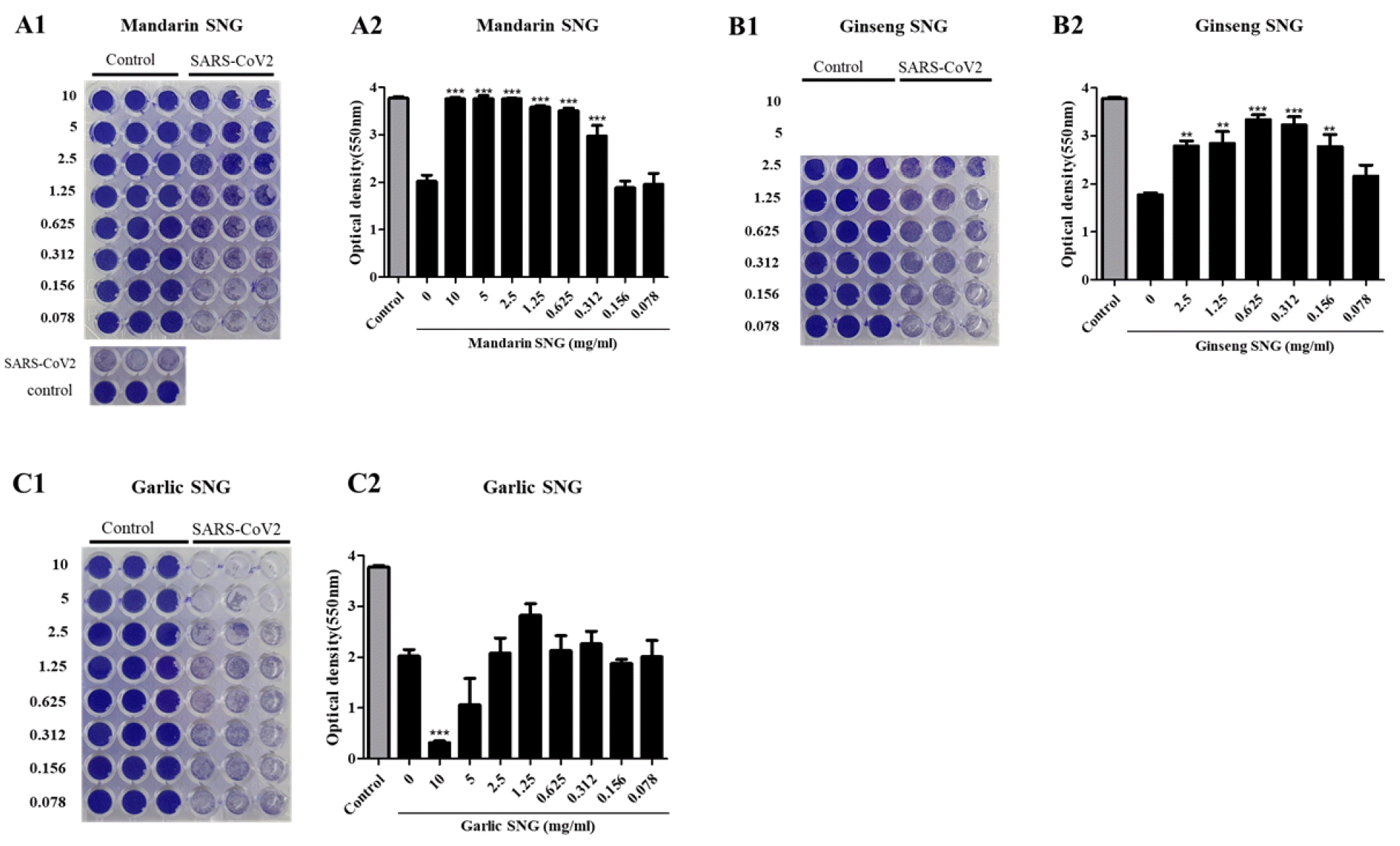
Mandarin SNG inhibits expression of nucleocapsid protein
IFA was performed on mandarin SNG that showed an effect of inhibiting CPE by SARS-CoV-2 replication. The protein expression of SARS-CoV-2 was significantly reduced in the group treated with mandarin SNG at 2.5 mg/ml compared to the group without mandarin SNG (Fig. 3). In the other concentrations, the protein expression decreased in a concentration- dependent manner (Fig. 3).
Fig. 3
Inhibitory effect of mandarin SNG on the expression of SASR-CoV-2 N-protein. Vero cells were treated with mandarin SNG of serially 2-fold diluted concentration from 0.625 to 2.5 mg/ml. Cells were fixed with cold acetone/methanol solution and stained with anti-SARS-CoV-2 nucleocapsid protein antibody with secondary antibody conjugated with FITC.
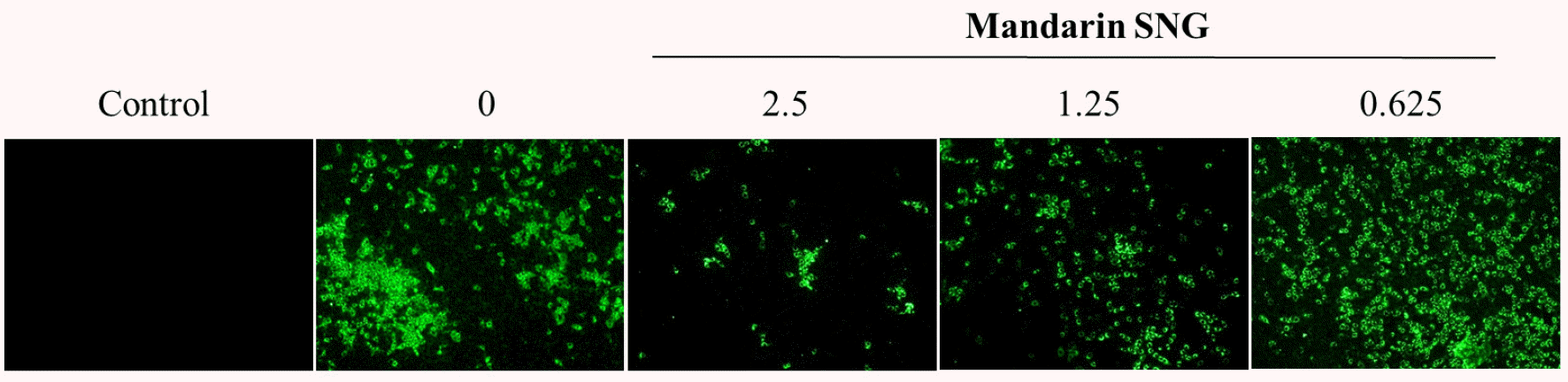
Inhibitory activity of mandarin SNG on SARS-CoV-2 replication
qRT-PCR was conducted to determine the effect of mandarin SNG treatment on the expression of SARS-CoV-2 gene (Fig. 4A), and plaque assay was conducted to confirm the inhibition of SARS-CoV-2 replication by measuring the amount of infectious virus released to the outside of the cell (Fig. 4B). When the mandarin SNG was treated in Vero cells at 2.5 mg/ml or higher, it was confirmed that the gene expression of SARS-CoV-2 was significantly reduced (Fig. 4B). In addition, when the mandarin SNG was treated in cells at 2.5 mg/ml or higher, it was confirmed that the amount of virus was reduced by 90% or higher (Fig. 4B).
Fig. 4
Inhibitory activity of mandarin SNG on SARS-CoV-2 replication. Vero cells were treated with mandarin SNG of diluted concentration from 0.625 to 2.5 mg/ml. Figure A shows the amount of SARS-CoV-2 RNA from Vero cells treated with mandarin SNG by qRT-PCR, and figure B shows the amount of SARS-CoV-2 in the cell supernatant treated with mandarin SNG by plaque assay. Values are expressed as Mean±SEM (Fig4-A). The different superscripts denote differences at **p< 0.01 (Fig. 4-A).
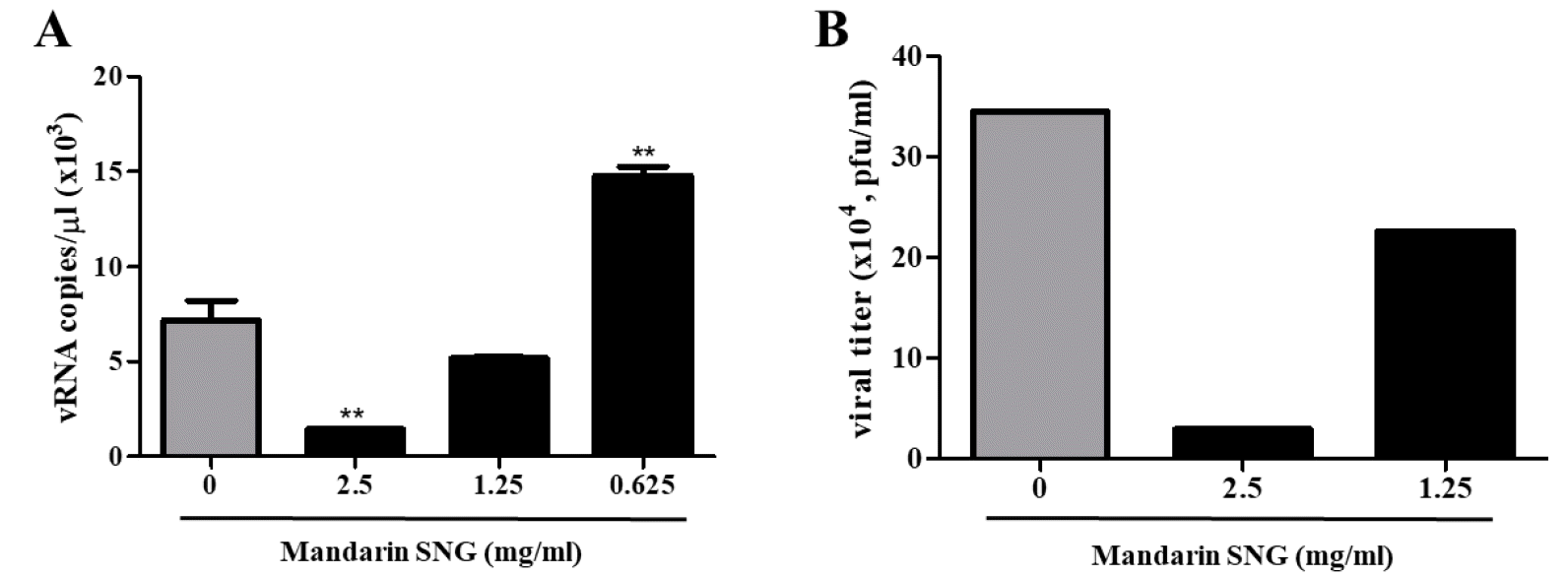
Flavonoids composition of mandarin SNG
Flavonoids are the main components of plant polyphenols which are well-known bioactive agents (22). In order to see which flavonoid could be the major component of mandarin SNG, we analyzed flavonoids content in mandarin SNG and found that narirutin and hesperidin were the two most common (Table 1).
DISCUSSION
SARS-CoV-2 has been prevalent globally since its outbreak in Wuhan, China (23). In order to suppress the SARS-CoV-2 pandemic, it is essential to develop not only a vaccine but also a therapeutic agent (24). Plant-derived natural substances have been studied as therapeutic candidates (12), and recent studies have shown that several natural extracts are effective in the inhibition of SARS-CoV-2. Platycodin D, a natural component of Platycodon grandiflorum, is known to inhibit the invasion of SARS-CoV-2 into cells by interfering with membrane fusion (25). Glycyrrhizin, an extract of licorice, inhibits SARS-CoV-2 replication by inhibiting the viral main protease Mpro (26). Epigallocatechin Gallate, abundant in green tea, is studied to inhibit Nsp15 of SARS-CoV-2 (27). In the present study, we demonstrated that mandarin SNG effectively inhibits SARS-CoV-2 replication in vitro.
A potential beneficial effect of citrus fruit extracts were widely studied, and a recent review highlighted the potential benefits of citrus fruit components for therapeutics or prophylaxis for viral infections such as COVID-19 (28). It is well known that citrus fruits are rich of vitamins and flavonoids. Among the flavonoids, hesperidin has been reported that it inhibits influenza virus (29). Haggag et al. suggested the hesperidin could be useful for prophylactic purpose and therapeutic of SARS-CoV-2 (30) and a molecular docking study by Joshi et al. demonstrated that hesperidin may strongly bind to SARS-CoV-2 protease and angiotensin-converting enzyme 2 (ACE-2) (31), suggesting that hesperidin may have anti-viral activity on SARS-CoV-2. A recent study by Kandeil et al. showed hesperidin possesses the virucidal effect as well as viral replication inhibitory effect (32). Antiviral effect of hesperidin was also demonstrated in mice infected with encephalomyocarditis virus (33). In the present study, we clearly demonstrated that SNG prepared from mandarin, one of major citrus fruits consumed in Korea, suppressed SARS-CoV-2 replication and this antiviral effect could be mediated by hesperidin which is a major flavonoids component of mandarin SNG.
In conclusion, we demonstrated the anti-viral activity of mandarin SNG on SARS-CoV-2 in vitro, and this result provides a potential use of these materials to prevent or treat COVID-19. However, further in-depth studies are necessary to define molecular mechanisms by which mandarin SNG suppresses SARS-CoV-2. In addition, in vivo studies using COVID-19 animal models are essential to prove its antiviral effects on SARS-CoV-2.