Abstract
Purpose
Recovery from exercise is a vulnerable phase that has been linked to increased susceptibility to sudden cardiovascular events. Cigarette smoking increases the risk of cardiovascular mortality and morbidity. We tested the hypothesis that postexercise cigarette smoking would attenuate hemodynamics and vascular function during recovery from exercise in young men.
Methods
Thirteen habitual smokers (age, 22±3 years; body mass index, 25.1±3.6 kg/m2) participated in (1) cigarette smoking (0.6 mg nicotine) and (2) sham smoking (SHAM) immediately postexercise (30 minutes on a treadmill; 40% to 60% of heart rate [HR] reserve) in a randomized order. Assessments were hemodynamics (HR, rate-pressure product [RPP], brachial and central artery blood pressures) and vascular function (arterial stiffness via carotid-femoral pulse wave velocity [PWV]; conduit vessel function via brachial artery flow-mediated dilation [FMD]). All variables were assessed at baseline, 10 minutes, and 30 minutes postexercise, except for FMD (baseline and 30 minutes postexercise).
Results
Compared with the SHAM trial, cigarette smoking increased HR, RPP, and brachial and central blood pressures postexercise (interaction, p<0.05). PWV reduced and FMD increased postexercise in the SHAM trial, while cigarette smoking attenuated exercise-induced improvements (interaction, p<0.05).
The favorable cardiovascular adaptations, including reductions in blood pressure and arterial stiffness, and an improvement in conduit vessel function, have been well elucidated following chronic endurance exercise training1,2; however, less is clear regarding the cardiovascular responses to acute aerobic exercise. In particular, the recovery period following acute aerobic exercise involves dynamic alterations in several physiological processes, which either return toward or decrease below a preexercise state, as well as are enhanced postexercise2. For instance, while reductions in brachial and central blood pressure following acute moderate-intensity aerobic exercise have been reported in young adults3,4, unequivocal information exists regarding postexercise changes in arterial stiffness and in conduit vessel5,6. Thus, additional investigation into the recovery period following acute aerobic exercise is clearly warranted, as it may offer not only a window of opportunity to maximize physiological changes in order to optimize long-term benefits, but also provide insight into potential factors that may attenuate the recovering cardiovascular system postexercise.
Importantly, the recovery period from exercise has also been linked to increased susceptibility to cardiovascular instability and sudden cardiovascular events (i.e., fatal tachycardia or sudden myocardial infarction) in vulnerable populations7. While several factors may contribute to cardiovascular instability during recovery from exercise, cigarette smoking may play an important role. Indeed, cigarette smoking is a modifiable cardiovascular risk factor that has been associated with arterial stiffening8-10 and conduit vessel dysfunction11 at rest, as well as impaired vasodilatory capacity during exercise12. Interestingly, even one cigarette smoking may acutely induce the activation of sympathetic tone13 and the reduction of nitric oxide bioavailability following increased inflammation and oxidative stress14. Therefore, it is possible that cigarette smoking may also attenuate the recovering cardiovascular system after acute exercise. Indeed, only a few studies, to date, have evaluated the impact of preexercise cigarette smoking on postexercise arterial stiffness, myocardial stress, and heart rate (HR) recovery in young adults9,15,16, but none have reported any information on conduit vessel function. Since chronic cigarette smokers tend to have a smoke immediately after leisure-time physical activity or exercise, the present study sought to test the hypothesis that postexercise cigarette smoking would attenuate hemodynamics, arterial stiffness, and conduit vessel function during recovery from moderate-intensity aerobic exercise in young men.
All experimental procedures and protocols were approved by the Institutional Review Board of University of Seoul (UOS IRB No. 2019-30). All aspects of the study conformed to the standards set by the Declaration of Helsinki, except for registration in a database. All experimental procedures were explained to participants in writing and verbally, and written informed consent was obtained from all participants prior to study participation.
Thirteen male habitual smokers volunteered to participate in this study. The self-reported smoking history was 6.8±2.8 years and 10±4 cigarettes per day (range, 6–20 cigarettes per day) and none of them smoked e-cigarettes. All participants were free from any known cardiovascular, metabolic, renal, or respiratory disease, and none were using any cardiovascular medications, nonsteroidal anti-inflammatory drugs, antihistamines, or multivitamin/antioxidant supplements. None were physically active, having not engaged in regular aerobic exercise for the past 6 months (i.e., <60 minutes of moderate-to-vigorous physical activity per week based on a self-reported physical activity questionnaire).
Using a cross-over design, all participants were assigned to either (1) cigarette smoking or (2) sham smoking (SHAM) immediately postexercise in a randomized order in two separate study visits, separated at least by 72 hours (Fig. 1). For both visits, participants arrived at the laboratory in the morning (between 8:00 and 10:00 A.M.) following an overnight fast (12 hours) and were instructed to abstain from alcohol, caffeine, and cigarette smoking (8 hours), as well as exercise (24 hours) for minimizing confounding effects. All data collection took place with participants in the supine position in a thermoneutral environment (∼22℃–24℃).
Upon arrival, all participants rested in the supine position for 10 minutes, after which baseline, preexercise measurements were obtained. Then, all participants exercised on a treadmill for 30 minutes at a moderate intensity (40%–60% of HR reserve [HRR]) in congruence with a previous study which reported increases of vascular function following a bout of aerobic exercise in young adults17. Warm-up and cool-down were performed with light-intensity treadmill walking (25%–30% of HRR) for 5 minutes each. Exercise intensity was monitored via a wireless HR monitor (RS800CX; Polar Electro, Kempele, Finland). Immediately after exercise cessation, all participants smoked either one cigarette (0.6 mg of nicotine) or a sham cigarette (nicotine-free, filtered straw without lighting), with the same frequency and duration of puffs. Then, measurements were obtained in the supine position at 10 minutes and 30 minutes postexercise, except for brachial artery flow-mediated dilation (FMD), which was obtained at baseline and 30 minutes postexercise only.
During the first study visit, all participants underwent assessments of anthropometrics, body composition, and a venous blood draw for descriptive characteristics. Body composition was determined using the bioelectrical impedance analysis (Inbody 3.0; Biospace, Seoul, Korea). A venous blood draw was obtained from the distal phalanx of the left hand with a lancing device (Accu-chek Soft Clix; Roche Diagnostics GmbH, Mannheim, Germany) and analyzed for blood lipid profiles (clinical chemistry analyzer, Samsung LABGEOPT10; Samsung Healthcare, Seoul, Korea).
Resting brachial blood pressure and HR were measured twice at a 2-minute interval using an automated blood pressure monitor (JPN601; OMRON Healthcare Corp., Kyoto, Japan). Central blood pressure, the pressure to which the heart, kidneys, and major arteries are exposed18, was estimated from radial pressure waveforms that were collected for 10 to 12 seconds using applanation tonometry with a high-fidelity strain-gauge transducer (Millar Instruments, Houston, TX). These radial waveforms were further transformed algorithmically using a validated generalized transfer function to estimate central blood pressure (SphygmoCor, AtCor Medical, Sydney, NSW, Australia). If blood pressure values differed by >10 mm Hg, another measurement was obtained, and the average value was used for subsequent analysis. Then, rate-pressure product (RPP), an indicator of myocardial oxygen demand, was calculated as the product of HR and brachial systolic blood pressure (SBP).
Carotid-femoral pulse wave velocity (PWV), a marker of arterial stiffness, was measured in accordance with established guidelines19. A high-fidelity strain-gauge transducer (Millar Instruments, Houston, TX, USA) was used to obtain pressure waveforms for a 10-second epoch from the right common carotid artery and the right femoral artery as previously described20. The peak of an in-phase R wave, as obtained from sequential electrocardiogram monitoring (CM5 configuration) was used as a timing marker. The foot of the pressure wave was identified automatically, removing potential observer bias, using an algorithm that detects the initial upstroke via a line tangent to the initial systolic upstroke point of the pressure tracing and an intersecting horizontal line through the minimum point. The distances from the carotid artery to the carotid artery and from the suprasternal notch to the femoral artery were measured as straight lines using a tape measure. The distance from the carotid artery to the carotid artery was then subtracted from the suprasternal notch-femoral segment to correct for differences in propagation direction along the arterial path length, and PWV was computed automatically using the equation: Δ distance (m)/Δ time (sec).
The brachial artery FMD testing for the assessment of conduit vessel function was performed in accordance with established guidelines21. Briefly, the longitudinal image of the brachial artery and mean blood velocity were imaged midway between the antecubital and axillary regions for 1 minute via ultrasonography (ARIETTA 60; Hitachi Aloka Medical, Tokyo, Japan) using a high-frequency (7.5 MHz) linear array probe (L441; Hitachi Aloka Medical). Then, a blood pressure cuff, which was positioned below the antecubital crease and distal to the ultrasound Doppler probe, was inflated to 250 mm Hg for 5 minutes. After cuff deflation, data were recorded continuously for 3 minutes. Images of vessel diameter and mean blood velocity were recorded and analyzed offline using automated edge-detection software (FMD Studio, Cardiovascular Suite; Quipu, Pisa, Italy). Brachial artery FMD was quantified as the maximal change in brachial artery diameter after cuff deflation, expressed as an absolute increase (Δ diameter [mm]) and a percentage increase from preocclusion values (%FMD). Shear rate was calculated as follows: shear rate (s−1)=mean blood velocity×8/vessel diameter. Shear rate area-under-the-curve (AUC) until peak vasodilation was also determined using the trapezoid rule. The same trained investigator, who was blinded to the study time points and experimental trials, performed and analyzed FMD measurements in all participants.
Statistical analyses were performed using IBM SPSS software (version 25.0; IBM Corp., Armonk, NY, USA). Normality was confirmed with the Shapiro-Wilk test. The Student paired t-tests were used to compare baseline differences between cigarette smoking and sham smoking. All outcome variables were assessed with a two-way repeated measures analysis of variance (time×trial). FMD was also adjusted for shear rate AUC by conducting repeated measure analysis of covariance (ANCOVA). In case of a significant interaction, post hoc tests with Bonferroni correction were performed. Data are presented as mean±standard deviation. Statistical significance was established at a p-value of <0.05.
Baseline descriptive characteristics are provided in Table 1. Preexercise and postexercise hemodynamics are presented in Table 2. At baseline, resting HR, RPP, and brachial and central SBP and diastolic blood pressure (DBP) were not different between the two trials (p>0.05). HR increased from baseline at 10 minutes postexercise and returned toward baseline at 30 minutes postexercise in the SHAM trial, whereas HR remained elevated above baseline in the cigarette smoking trial and was higher in the cigarette smoking trial than in the SHAM trial throughout postexercise recovery (interaction, p<0.05). RPP increased from baseline in the cigarette trial only and was higher in the cigarette smoking trial than in the SHAM trial throughout postexercise recovery (interaction, p<0.05). Brachial SBP decreased at 30 minutes postexercise in the SHAM trial and was lower in the SHAM trial than in the cigarette smoking trial throughout postexercise recovery (interaction, p<0.05). Brachial DBP as well as central systolic and DBP decreased at 30 minutes postexercise in the SHAM trial, but increased at 10 minutes postexercise in the cigarette smoking trial and was higher in the cigarette smoking trial than in the SHAM trial through postexercise recovery (interaction, p<0.05).
Preexercise and postexercise arterial stiffness and conduit vessel function are presented in Fig. 2 and Table 3. At baseline, carotid-femoral PWV and brachial artery FMD variables were not different between the two trials (p>0.05), except for shear rate AUC. Carotid-femoral PWV decreased from baseline at 30 minutes postexercise in the SHAM trial only and was lower in the SHAM trial than in the cigarette smoking trial throughout postexercise recovery (interaction, p<0.05) (Fig. 2A). Resting brachial artery diameter, resting shear rate, and time to peak vasodilation did not change from baseline at 30 minutes postexercise in any trials (p>0.05 for all) (Table 3). Peak brachial artery diameter increased from baseline at 30 minutes postexercise similarly in both trials (p<0.05) (Table 3). Brachial artery FMD, assessed by both absolute change in brachial artery diameter (Table 3) and the peak percentage change (Fig. 2B), increased at 30 minutes postexercise in the SHAM trial, but not in the cigarette smoking trial (interaction, p<0.05). Shear rate AUC was different between trials only at baseline and increased from baseline at 30 minutes postexercise in the SHAM trial only (interaction, p<0.05) (Table 3). Percent FMD divided by shear rate AUC was not different between trials at any time points and did not change postexercise (p>0.05) (Table 3). Adjusting for shear rate AUC using ANCOVA, percent FMD was also no longer statistically significant (interaction, p=0.053) (Table 3).
The main findings of this study were two-fold. First, acute cigarette smoking immediately after aerobic exercise attenuated hemodynamic recovery, as evidenced by sustained elevations in HR, RRP, and brachial and central blood pressures. Second, the delayed hemodynamic recovery was accompanied by attenuation in an exercise-induced reduction in arterial stiffness and improvement in conduit vessel function (via brachial artery FMD). Taken together, these findings highlight the negative ramification of postexercise cigarette smoking on hemodynamics, arterial stiffness, and conduit vessel function during recovery from moderate-intensity aerobic exercise in young men.
The dynamic alterations in hemodynamics following an acute bout of moderate-intensity aerobic exercise have been well studied, including HR recovery and postexercise hypotension2. In the present study, we observed delayed HR recovery, coupled with elevated RPP throughout exercise recovery in the cigarette smoking trial compared with the sham trial (Table 2), demonstrating the negative impact of postexercise cigarette smoking on autonomic function and myocardial oxygen demand. Our findings are in agreement with previous work that has identified elevations in HR and RPP during and following 3 minutes isometric handgrip exercise when the participants smoked one cigarette 10 minutes prior to exercise initiation16. The delayed autonomic recovery following exercise in the cigarette smoking trial may be attributed, in part, to an increase in sympathetic dominance and delayed parasympathetic reactivation13. Plasma catecholamine concentrations have also been reported to increase following acute cigarette smoking and remained elevated for 30 minutes afterwards22, and this sympathetic activation is thought to be mediated by nicotine23. Given that delayed autonomic recovery, which contributes to an increase in myocardial oxygen demand, has been associated with an increased risk of future hypertension and mortality24,25, our findings of delayed autonomic recovery and increased myocardial oxygen in the cigarette smoking trial suggest that cigarette smoking should be avoided to minimize susceptibility to cardiovascular instability and the risk of sudden cardiovascular events following exercise.
In a previous study, cigarette smoking has been reported to acutely increase brachial and central blood pressures for approximately 15 minutes22. Our findings extend those of the previous study22 by demonstrating that cigarette smoking immediately after exercise attenuated the reductions in brachial and central blood pressures following exercise (Table 2). The reduction in brachial blood pressure following acute aerobic exercise is typically accompanied by a reduction in total peripheral resistance that is not completely offset by an increase in cardiac output2,26. It is likely that cigarette smoking, which increases sympathetic activation and release of catecholamines27, may have attenuated the reductions in brachial blood pressure following exercise, due to an increase in total peripheral resistance and an elevation in HR28. In an animal study, acute cigarette smoking induces an increase in vasoconstriction that is mediated, in part, via release of a vasoconstrictor thromboxane A229, demonstrating another potential mechanism by which postexercise cigarette smoking may have attenuated the reduction in brachial blood pressure in the present study. In contrast to brachial blood pressure, central blood pressure is the pressure to which the heart, kidneys, and major arteries are exposed18 and is a better predictor of future hypertension and cardiovascular mortality30. In the present study, we found elevations in central blood pressure following exercise in the cigarette smoking trial (Table 2). Since the heart is exposed to central blood pressure, our findings of elevations in central blood pressure induced by cigarette smoking are in support of the rise in myocardial oxygen demand after exercise. The mechanisms by which cigarette smoking increases central blood pressure after exercise are unclear, but may be mediated, in part, by an increase in arterial wave reflection and a reduction in pulse pressure amplification8,31. Taken together, extending previous studies, our findings of attenuated postexercise reductions in brachial and central blood pressure provide new evidence for the ability of cigarette smoking to mitigate the hypotensive benefits associated with acute aerobic exercise.
Increased arterial stiffness and conduit vessel dysfunction are independent predictors of future cardiovascular events, independently of traditional cardiovascular risk factors32,33. While chronic aerobic exercise has been demonstrated to provoke favorable changes in vascular health, including reduced arterial stiffness and improved conduit vessel function34, the acute impacts of moderate-intensity aerobic exercise on arterial stiffness and conduit vessel function are less clear. In the present study, we found a reduction in PWV and an increase in brachial FMD in the sham trial (Fig. 2), suggesting exercise-induced improvements in arterial stiffness and conduit vessel function. In contrast to our findings, a previous study observed no change in PWV following acute moderate-intensity exercise in healthy men, demonstrating no evidence of exercise-induced improvement in arterial stiffness5. However, conduit vessel function, assessed via brachial artery FMD, has been reported to transiently improve following acute moderate-intensity exercise5,6, which is in agreement with findings from the present study. The mechanisms by which acute moderate-intensity exercise improved arterial stiffness and conduit vessel function are multifactorial but may be mediated, in part, via shear stress-induced production of nitric oxide from the endothelial cells and vascular smooth muscle cell relaxation5,35.
Importantly, although the unfavorable effects of both chronic and acute cigarette smoking on arterial stiffness14,21 and conduit vessel function11 have been documented, no studies, to date, have evaluated impact of postexercise cigarette smoking on arterial stiffness and conduit vessel function. Extending these previous studies, our findings of no changes in arterial stiffness and conduit vessel function following acute exercise in the cigarette smoking trial (Fig. 2) suggest that cigarette smoking may abolish an improvement in arterial stiffness and conduit vessel function provoked by exercise. The potential mechanism underlying it could be mediated by cigarette smoking-induced increases in inflammation and the associated production of reactive oxygen species, and the resultant reduction in nitric oxide bioavailability36,37. In addition, given the importance of shear stimulus in mediating the FMD response38, we controlled for the difference in shear rate AUC for the FMD response (Table 3). Although we lost statistical significance with the percent FMD and shear rate AUC ratio (p=0.959), ANCOVA-corrected percent FMD approached significance (p=0.053). It is important to note that shear rate appears to explain up to a third of between-person variability in the FMD response39, and, thus, the loss of statistical power when controlling for the shear stimulus may be, due, in part to high variability in the shear rate AUC data in the present study. Nevertheless, our findings of attenuated improvement in conduit vessel function in the cigarette smoking trial (Fig. 2B) provide new evidence for the potential of cigarette smoking to dampen the positive effects of acute exercise on arterial stiffness and conduit vessel function.
We acknowledge experimental considerations that may be perceived as limitations to the present study. First, our participants were habitual male smokers, which limits our ability to infer about potential sex differences or postexercise responses in non-smokers. Second, we did not directly assess cardiorespiratory fitness in our participants. Given that regular participation in physical activity has been reported to attenuate any adverse effects of cigarette smoking on vascular function10, future studies should explore if individuals with high fitness levels are protected against cigarette smoking-induced transient impairment in hemodynamics and vascular function following exercise.
Cigarette smoking immediately after exercise attenuated hemodynamic recovery and an improvement in arterial stiffness and conduit vessel function in young habitual smokers. Together, these findings provide evidence for the adverse effects of cigarette smoking on hemodynamic and vascular function during recovery from exercise.
REFERENCES
1. Hellsten Y, Nyberg M. 2015; Cardiovascular adaptations to exercise training. Compr Physiol. 6:1–32. DOI: 10.1002/cphy.c140080. PMID: 26756625.


2. Romero SA, Minson CT, Halliwill JR. 2017; The cardiovascular system after exercise. J Appl Physiol (1985). 122:925–32. DOI: 10.1152/japplphysiol.00802.2016. PMID: 28153943. PMCID: PMC5407206.


3. Brito LC, Fecchio RY, Peçanha T, Andrade-Lima A, Halliwill JR, Forjaz CL. 2018; Postexercise hypotension as a clinical tool: a "single brick" in the wall. J Am Soc Hypertens. 12:e59–64. DOI: 10.1016/j.jash.2018.10.006. PMID: 30425018.


4. Bunsawat K, Lefferts EC, Grigoriadis G, et al. 2021; Central and peripheral postexercise blood pressure and vascular responses in young adults with obesity. Med Sci Sports Exerc. 53:994–1002. DOI: 10.1249/MSS.0000000000002540. PMID: 33060547.


5. Siasos G, Athanasiou D, Terzis G, et al. 2016; Acute effects of different types of aerobic exercise on endothelial function and arterial stiffness. Eur J Prev Cardiol. 23:1565–72. DOI: 10.1177/2047487316647185. PMID: 27121699.


6. Hwang IC, Kim KH, Choi WS, et al. 2012; Impact of acute exercise on brachial artery flow-mediated dilatation in young healthy people. Cardiovasc Ultrasound. 10:39. DOI: 10.1186/1476-7120-10-39. PMID: 23031621. PMCID: PMC3519716.


7. Goodman JM, Burr JF, Banks L, Thomas SG. 2016; The acute risks of exercise in apparently healthy adults and relevance for prevention of cardiovascular events. Can J Cardiol. 32:523–32. DOI: 10.1016/j.cjca.2016.01.019. PMID: 27017149.


8. Mahmud A, Feely J. 2003; Effect of smoking on arterial stiffness and pulse pressure amplification. Hypertension. 41:183–7. DOI: 10.1161/01.HYP.0000047464.66901.60. PMID: 12511550.


9. Doonan RJ, Scheffler P, Yu A, et al. 2011; Altered arterial stiffness and subendocardial viability ratio in young healthy light smokers after acute exercise. PLoS One. 6:e26151. DOI: 10.1371/journal.pone.0026151. PMID: 22028821. PMCID: PMC3189960.


10. Park W, Miyachi M, Tanaka H. 2014; Does aerobic exercise mitigate the effects of cigarette smoking on arterial stiffness? J Clin Hypertens (Greenwich). 16:640–4. DOI: 10.1111/jch.12385. PMID: 25135246.


11. Carnevale R, Sciarretta S, Violi F, et al. 2016; Acute impact of tobacco vs electronic cigarette smoking on oxidative stress and vascular function. Chest. 150:606–12. DOI: 10.1016/j.chest.2016.04.012. PMID: 27108682.


12. Gaenzer H, Neumayr G, Marschang P, Sturm W, Kirchmair R, Patsch JR. 2001; Flow-mediated vasodilation of the femoral and brachial artery induced by exercise in healthy nonsmoking and smoking men. J Am Coll Cardiol. 38:1313–9. DOI: 10.1016/S0735-1097(01)01575-3. PMID: 11691501.


13. Mendonca GV, Pereira FD, Fernhall B. 2011; Effects of cigarette smoking on cardiac autonomic function during dynamic exercise. J Sports Sci. 29:879–86. DOI: 10.1080/02640414.2011.572991. PMID: 21547834.


14. Vlachopoulos C, Ioakeimidis N, Abdelrasoul M, et al. 2016; Electronic cigarette smoking increases aortic stiffness and blood pressure in young smokers. J Am Coll Cardiol. 67:2802–3. DOI: 10.1016/j.jacc.2016.03.569. PMID: 27282901.


15. Papathanasiou G, Georgakopoulos D, Papageorgiou E, et al. 2013; Effects of smoking on heart rate at rest and during exercise, and on heart rate recovery, in young adults. Hellenic J Cardiol. 54:168–77. PMID: 23685653.
16. Anyfanti P, Triantafyllidou E, Papadopoulos S, et al. 2017; Smoking before isometric exercise amplifies myocardial stress and dysregulates baroreceptor sensitivity and cerebral oxygenation. J Am Soc Hypertens. 11:376–84. DOI: 10.1016/j.jash.2017.04.004. PMID: 28456497.


17. Johnson BD, Padilla J, Wallace JP. 2012; The exercise dose affects oxidative stress and brachial artery flow-mediated dilation in trained men. Eur J Appl Physiol. 112:33–42. DOI: 10.1007/s00421-011-1946-8. PMID: 21472439.


18. Kollias A, Lagou S, Zeniodi ME, Boubouchairopoulou N, Stergiou GS. 2016; Association of central versus brachial blood pressure with target-organ damage: systematic review and meta-analysis. Hypertension. 67:183–90. DOI: 10.1161/HYPERTENSIONAHA.115.06066. PMID: 26597821.
19. Van Bortel LM, Duprez D, Starmans-Kool MJ, Safar ME, Giannattasio C, Cockcroft J, et al. 2002; Clinical applications of arterial stiffness, Task Force III: recommendations for user procedures. Am J Hypertens. 15:445–52. DOI: 10.1016/S0895-7061(01)02326-3. PMID: 12022247.


20. Bunsawat K, Ranadive SM, Lane-Cordova AD, Yan H, Kappus RM, Fernhall B, et al. 2017; The effect of acute maximal exercise on postexercise hemodynamics and central arterial stiffness in obese and normal-weight individuals. Physiol Rep. 5:e13226. DOI: 10.14814/phy2.13226. PMID: 28364031. PMCID: PMC5392516.


21. Thijssen DH, Bruno RM, van Mil AC, et al. 2019; Expert consensus and evidence-based recommendations for the assessment of flow-mediated dilation in humans. Eur Heart J. 40:2534–47. DOI: 10.1093/eurheartj/ehz350. PMID: 31211361.


22. Franzen KF, Willig J, Cayo Talavera S, Meusel M, Sayk F, Reppel M, et al. 2018; E-cigarettes and cigarettes worsen peripheral and central hemodynamics as well as arterial stiffness: a randomized, double-blinded pilot study. Vasc Med. 23:419–25. DOI: 10.1177/1358863X18779694. PMID: 29985113.


23. Narkiewicz K, van de Borne PJ, Hausberg M, et al. 1998; Cigarette smoking increases sympathetic outflow in humans. Circulation. 98:528–34. DOI: 10.1161/01.CIR.98.6.528. PMID: 9714109.


24. Cole CR, Blackstone EH, Pashkow FJ, Snader CE, Lauer MS. 1999; Heartrate recovery immediately after exercise as a predictor of mortality. N Engl J Med. 341:1351–7. DOI: 10.1056/NEJM199910283411804. PMID: 10536127.


25. Jae SY, Bunsawat K, Fadel PJ, Fernhall B, Choi YH, Park JB, et al. 2016; Attenuated heart rate recovery after exercise testing and risk of incident hypertension in men. Am J Hypertens. 29:1103–8. DOI: 10.1093/ajh/hpw028. PMID: 27037713.


26. Halliwill JR. 2001; Mechanisms and clinical implications of postexercise hypotension in humans. Exerc Sport Sci Rev. 29:65–70. DOI: 10.1249/00003677-200104000-00005. PMID: 11337825.


27. Walker JF, Collins LC, Rowell PP, Goldsmith LJ, Moffatt RJ, Stamford BA. 1999; The effect of smoking on energy expenditure and plasma catecholamine and nicotine levels during light physical activity. Nicotine Tob Res. 1:365–70. DOI: 10.1080/14622299050011501. PMID: 11072434.


28. Pickering TG. 1990; Inheritance of hypertension and blood pressure reactivity. Hypertension. 16:498–500. DOI: 10.1161/01.HYP.16.5.498. PMID: 2228149.


29. Iida M, Iida H, Dohi S, Takenaka M, Fujiwara H. 1998; Mechanisms underlying cerebrovascular effects of cigarette smoking in rats in vivo. Stroke. 29:1656–65. DOI: 10.1161/01.STR.29.8.1656. PMID: 9707209.


30. McEniery CM, Cockcroft JR, Roman MJ, Franklin SS, Wilkinson IB. 2014; Central blood pressure: current evidence and clinical importance. Eur Heart J. 35:1719–25. DOI: 10.1093/eurheartj/eht565. PMID: 24459197. PMCID: PMC4155427.


31. Saladini F, Benetti E, Fania C, Mos L, Casiglia E, Palatini P. 2016; Effects of smoking on central blood pressure and pressure amplification in hypertension of the young. Vasc Med. 21:422–8. DOI: 10.1177/1358863X16647509. PMID: 27197683.


32. Vlachopoulos C, Aznaouridis K, Stefanadis C. 2010; Prediction of cardiovascular events and all-cause mortality with arterial stiffness: a systematic review and meta-analysis. J Am Coll Cardiol. 55:1318–27. DOI: 10.1016/j.jacc.2009.10.061. PMID: 20338492.
33. Darabont R, Tautu OF, Pop D, et al. 2015; Visit-to-visit blood pressure variability and arterial stiffness independently predict cardiovascular risk category in a general population: results from the SEPHAR II Study. Hellenic J Cardiol. 56:208–16. PMID: 26021242.
34. Tanaka H. 2019; Antiaging effects of aerobic exercise on systemic arteries. Hypertension. 74:237–43. DOI: 10.1161/HYPERTENSIONAHA.119.13179. PMID: 31256721.


35. Ashor AW, Lara J, Siervo M, Celis-Morales C, Mathers JC. 2014; Effects of exercise modalities on arterial stiffness and wave reflection: a systematic review and meta-analysis of randomized controlled trials. PLoS One. 9:e110034. DOI: 10.1371/journal.pone.0110034. PMID: 25333969. PMCID: PMC4198209.


36. Csiszar A, Podlutsky A, Wolin MS, Losonczy G, Pacher P, Ungvari Z. 2009; Oxidative stress and accelerated vascular aging: implications for cigarette smoking. Front Biosci (Landmark Ed). 14:3128–44. DOI: 10.2741/3440. PMID: 19273262. PMCID: PMC2756477.


37. Gül I, Karapinar H, Yarlioglues M, et al. 2011; Acute effects of passive smoking on endothelial function. Angiology. 62:245–7. DOI: 10.1177/0003319710377077. PMID: 20682610.


38. Tinken TM, Thijssen DH, Hopkins N, et al. 2009; Impact of shear rate modulation on vascular function in humans. Hypertension. 54:278–85. DOI: 10.1161/HYPERTENSIONAHA.109.134361. PMID: 19546374. PMCID: PMC3012006.


39. Gibbs BB, Dobrosielski DA, Lima M, Bonekamp S, Stewart KJ, Clark JM. 2011; The association of arterial shear and flow-mediated dilation in diabetes. Vasc Med. 16:267–74. DOI: 10.1177/1358863X11411361. PMID: 21708874. PMCID: PMC4397901.


Fig. 2
Effects of postexercise cigarette smoking on (A) carotid-femoral pulse wave velocity (PWV) and (B) brachial artery flow-mediated dilation (FMD). Values are presented as mean±standard deviation. *p<0.05, different from baseline; †p< 0.05, different from the sham trial.
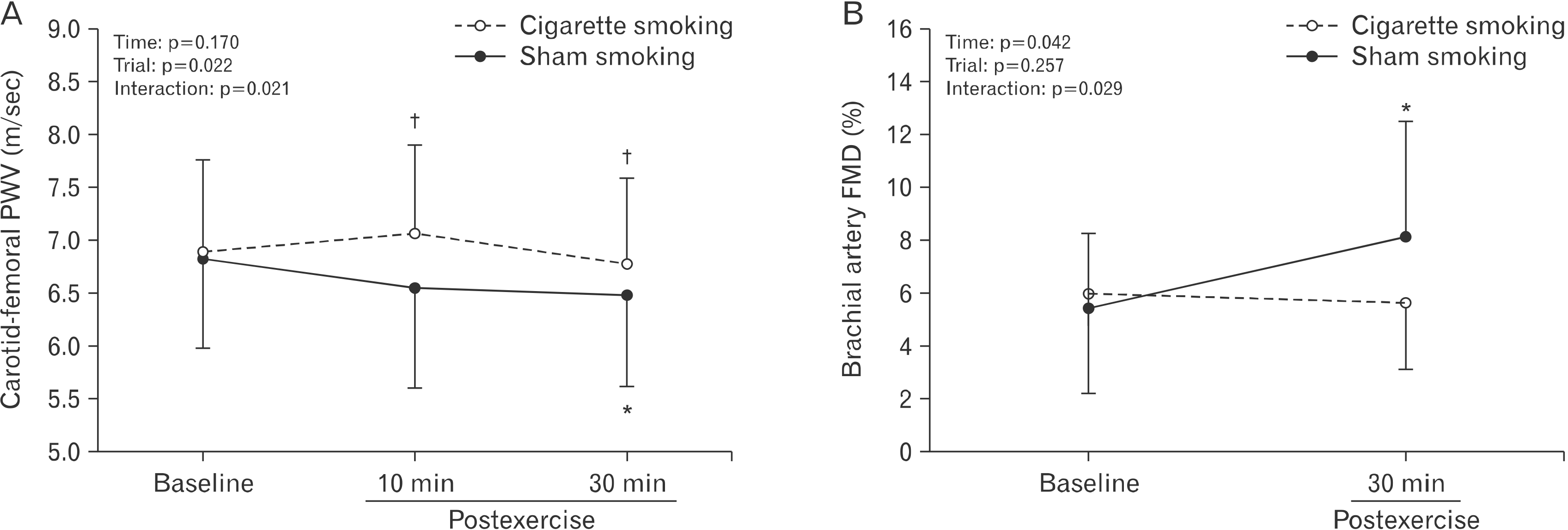
Table 1
Descriptive characteristics
Table 2
Effects of postexercise cigarette smoking on hemodynamics during recovery from exercise
Variable | Trial | Baseline | Post 10 min | Post 30 min | p-value | ||
---|---|---|---|---|---|---|---|
|
|||||||
Time | Trial | Interaction | |||||
Brachial SBP (mm Hg) | Cigarette | 120±9 | 124±1‡ | 118±11†,‡ | 0.001 | 0.003 | 0.027 |
Sham | 118±8 | 115±8 | 111±7*,† | ||||
Brachial DBP (mm Hg) | Cigarette | 73±8 | 79±11*,‡ | 75±11†,‡ | 0.002 | 0.009 | 0.001 |
Sham | 72±9 | 72±9 | 69±9 | ||||
Central SBP (mm Hg) | Cigarette | 103±8 | 107±10‡ | 102±9†,‡ | 0.001 | 0.004 | 0.006 |
Sham | 101±7 | 99±8 | 96±7*,† | ||||
Central DBP (mm Hg) | Cigarette | 74±8 | 82±12*,‡ | 76±11†,‡ | 0.001 | 0.004 | <0.001 |
Sham | 73±9 | 73±9 | 70±10† | ||||
Heart rate (beats/min) | Cigarette | 61±7 | 79±14*,‡ | 71±13*,†,‡ | <0.001 | 0.008 | <0.001 |
Sham | 61±8 | 68±9* | 65±8 | ||||
RPP | Cigarette | 7,284±1,238 | 9,787±2,036*,‡ | 8,908±1,842*,‡ | <0.001 | <0.001 | <0.001 |
Sham | 7,288±1,294 | 7,838±1,503 | 7,218±1,297 |
Table 3
Effects of postexercise cigarette smoking on conduit vessel function during recovery from exercise
Variable | Trial | Baseline | Post 30 min | p-value | ||
---|---|---|---|---|---|---|
|
||||||
Time | Trial | Interaction | ||||
Resting diameter (mm) | Cigarette | 3.98±0.46 | 4.02±0.49 | 0.717 | 0.695 | 0.256 |
Sham | 4.00±0.43 | 3.98±0.44 | ||||
Resting shear rate (sec−1) | Cigarette | 378±142 | 331±84 | 0.242 | 0.122 | 0.740 |
Sham | 319±126 | 286±115 | ||||
Peak diameter (mm) | Cigarette | 4.22±0.49 | 4.24±0.54 | 0.030 | 0.583 | 0.185 |
Sham | 4.21±0.46 | 4.30±0.49* | ||||
Absolute FMD (mm) | Cigarette | 0.24±0.15 | 0.23±0.11 | 0.021 | 0.297 | 0.031 |
Sham | 0.21±0.09 | 0.33±0.17* | ||||
Percent FMD (%) | Cigarette | 6.00±3.77 | 5.67±2.54 | 0.042 | 0.257 | 0.029 |
Sham | 5.47±2.80 | 8.16±4.37* | ||||
Shear rate AUC | Cigarette | 16,073±8,315† | 20,715±10,698 | 0.840 | 0.518 | 0.005 |
Sham | 22,516±10,374 | 17,009±7,393* | ||||
Time to peak vasodilation (sec) | Cigarette | 64±17 | 66±17 | 0.862 | 0.230 | 0.833 |
Sham | 60±22 | 59±15 | ||||
Percent FMD (%)/shear rate AUC ratio (10−3 AU) | Cigarette | 0.46±0.33 | 0.36±0.29 | 0.668 | 0.362 | 0.959 |
Sham | 0.72±1.76 | 0.59±0.44 | ||||
ANCOVA-corrected percent FMD (%) | Cigarette | 6.00±3.77 | 5.67±2.54† | 0.734 | 0.643 | 0.053 |
Sham | 5.47±2.80 | 8.16±4.37* |