Abstract
Background and Objectives
Materials and Methods
Results
Acknowledgments
Notes
Author Contributions
Y.S.J. and J.L. designed the project and supervised the research; S.J., S.G.L., H.J.K., G.L., and S.P. performed the analyses; S.J. and S.G.L. performed the computational analysis; S.P. and I-K.K. contributed to discussions about the research; S.J., S.G.L., Y.S.J., and J.L. wrote the manuscript; all authors reviewed the manuscript.
References

























Fig. 1
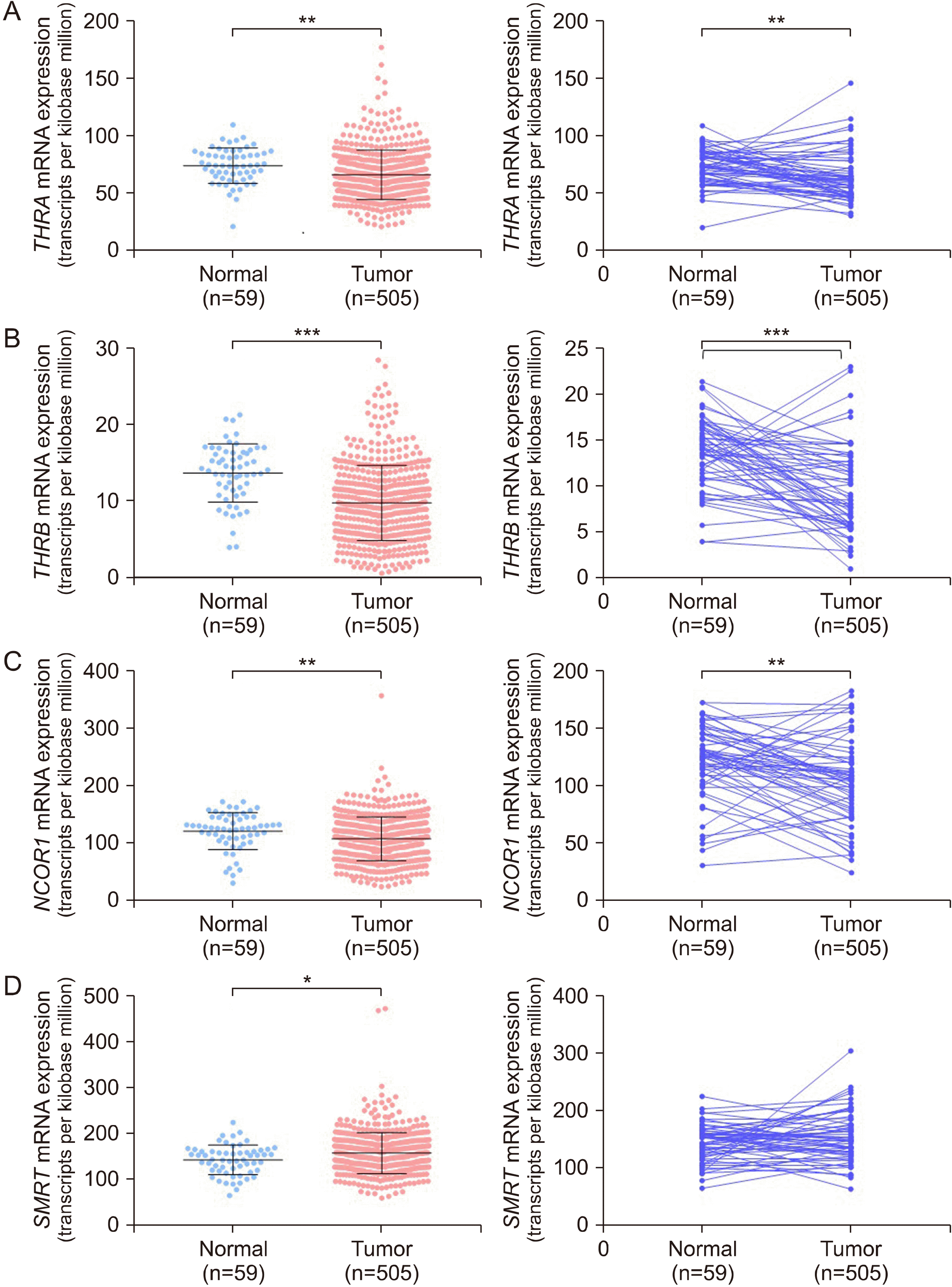
Fig. 2
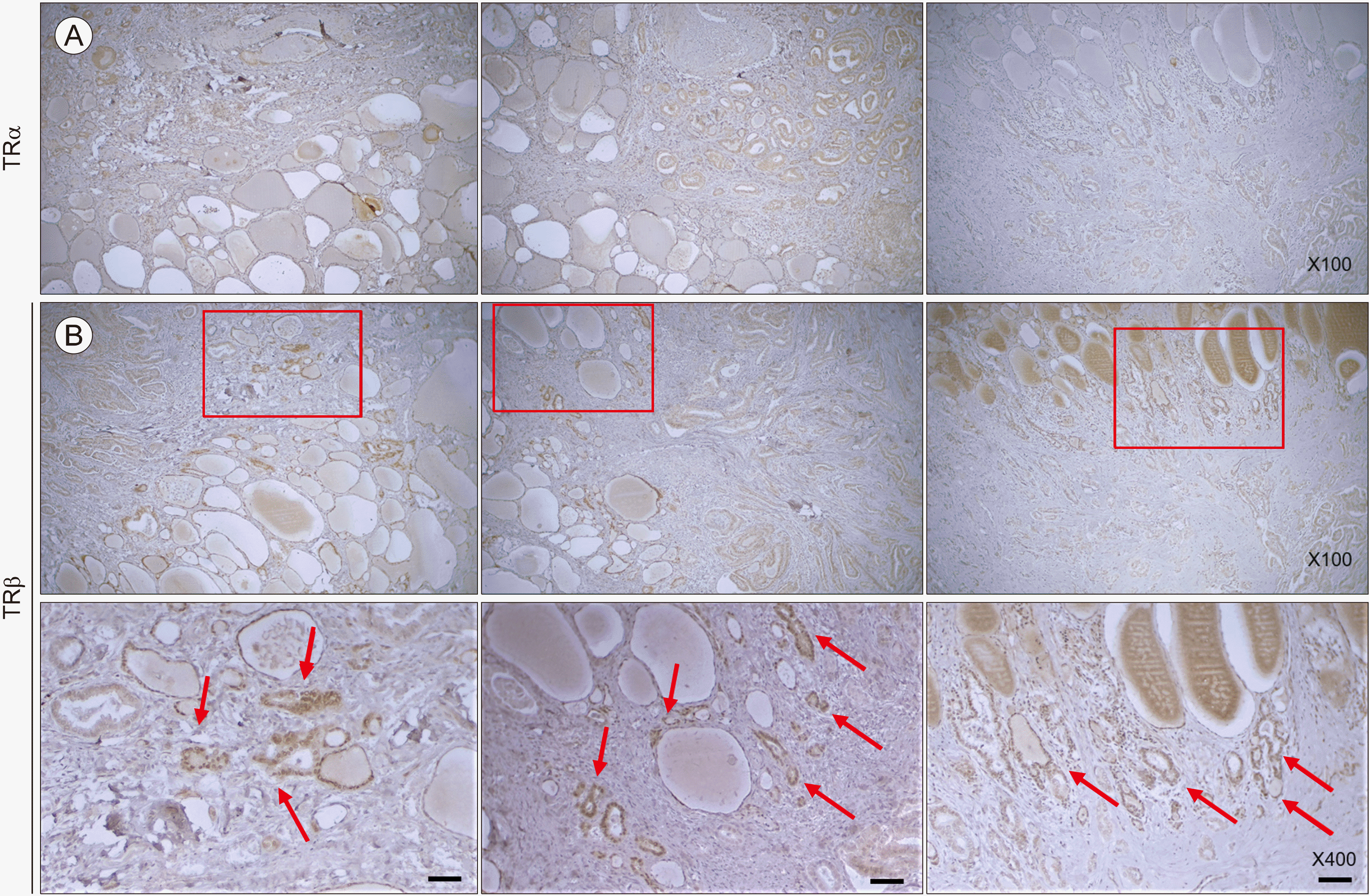
Fig. 3

Fig. 4
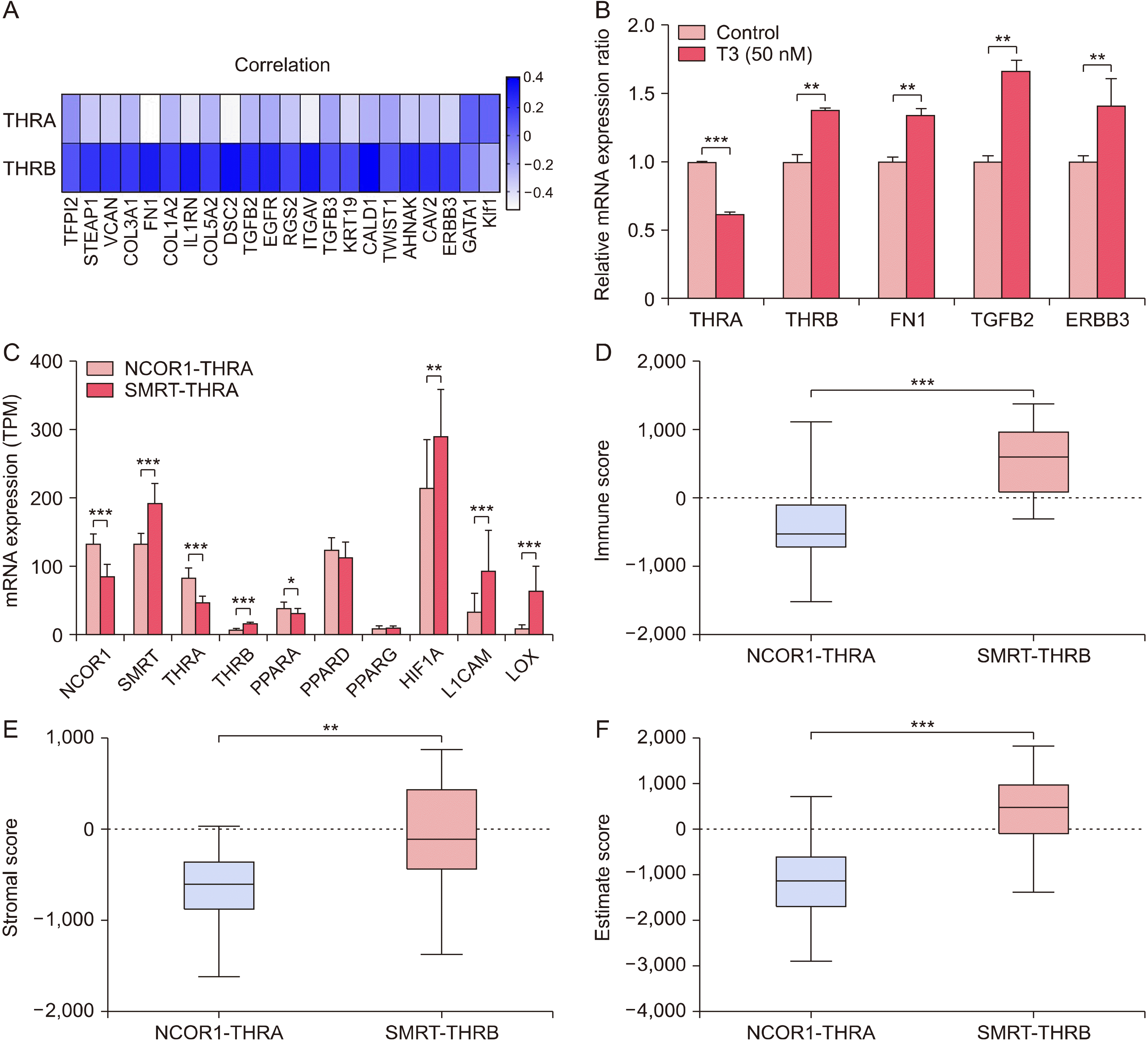
Table 1
Characteristics | THRA | p-value | |
---|---|---|---|
|
|||
Low expression n=126 (%) | High expression n=126 (%) | ||
NCOR1 expression | 112.33±35.59 | 103.92±43.00 | 0.092* |
SMRT expression | 167.14±41.05 | 153.82±40.66 | 0.010* |
Age (years) | 46.64±17.22 | 49.56±15.84 | 0.180* |
Gender (Male:Female) | 34 (27.0):92 (73.0) | 34 (27.0):92 (73.0) | 0.999† |
Tumor size (cm) | 3.02±1.64 | 2.99±1.75 | 0.960* |
MACIS score | 5.59±1.66 | 5.30±1.38 | 0.173* |
Histologic subtype | <0.0001† | ||
Follicular variant | 5 (4.0) | 37 (30.3) | |
Conventional | 100 (80.6) | 80 (65.6) | |
Tall cell variant | 18 (14.5) | 2 (1.6) | |
Othersς | 1 (0.8) | 3 (2.5) | |
Extrathyroidal extension | <0.0001† | ||
No | 65 (57.5) | 89 (80.2) | |
Yes | 48 (42.5) | 22 (19.8) | |
Multifocality | 0.048† | ||
No | 73 (58.9) | 55 (46.2) | |
Yes | 51 (41.1) | 64 (53.8) | |
T stage | 0.013† | ||
T1 | 27 (23.3) | 38 (32.8) | |
T2 | 33 (28.4) | 41 (35.3) | |
T3 | 48 (41.4) | 33 (28.4) | |
T4 | 8 (6.9) | 4 (3.4) | |
N stage | <0.0001† | ||
N0 | 36 (33.0) | 64 (64.6) | |
N1 | 73 (67.0) | 35 (35.4) | |
M stage | 0.480† | ||
M0 | 69 (98.6) | 59 (96.7) | |
M1 | 1 (1.4) | 2 (3.3) | |
TNM stage | 0.127† | ||
I | 63 (53.8) | 62 (54.4) | |
II | 5 (4.3) | 20 (17.5) | |
III | 33 (28.2) | 25 (21.9) | |
IV | 16 (13.7) | 7 (6.1) |
NCOR1: nuclear receptor co-repressor 1, MACIS: distant metastasis, patient age, completeness of resection, local invasion, and tumor size, SMRT: silencing mediator for retinoid or thyroid hormone receptor, SD: standard deviation, TCGA: The Cancer Genome Atlas, THCA: thyroid cancer, THRA: thyroid hormone receptor alpha, TNM: tumor-node- metastasis
Table 2
Characteristics | THRB | p-value | |
---|---|---|---|
|
|||
Low expression n=126 (%) | High expression n=126 (%) | ||
NCOR1 expression | 94.22±37.54 | 120.88±34.07 | <0.0001* |
SMRT expression | 141.19±38.16 | 176.54±49.18 | <0.0001* |
Age (years) | 43.62±13.84 | 49.29±15.88 | 0.004* |
Gender (Male:Female) | 28 (22.2):98 (77.8) | 25 (19.8):101 (80.2) | 0.643† |
Tumor size (cm) | 3.00±1.64 | 2.97±1.61 | 0.893* |
MACIS score | 5.01±1.42 | 5.64±1.65 | 0.003* |
Histologic subtype | 0.047† | ||
Follicular variant | 29 (23.0) | 20 (16.0) | |
Conventional | 92 (73.0) | 90 (72.0) | |
Tall cell variant | 3 (2.4) | 15 (12.0) | |
Othersς | 2 (1.6) | 0 (0.0) | |
Extrathyroidal extension | 0.005† | ||
No | 92 (78.6) | 69 (61.6) | |
Yes | 25 (21.4) | 43 (38.4) | |
Multifocality | 0.276† | ||
No | 66 (54.1) | 75 (61.0) | |
Yes | 56 (45.9) | 48 (39.0) | |
T stage | 0.129† | ||
T1 | 35 (29.4) | 32 (27.4) | |
T2 | 49 (41.2) | 37 (31.6) | |
T3 | 32 (26.9) | 41 (35.0) | |
T4 | 3 (2.5) | 7 (6.0) | |
N stage | 0.065† | ||
N0 | 63 (57.8) | 46 (45.1) | |
N1 | 46 (42.2) | 56 (54.9) | |
M stage | 0.941† | ||
M0 | 63 (95.5) | 67 (95.7) | |
M1 | 3 (4.5) | 3 (4.3) | |
TNM stage | 0.040† | ||
I | 74 (62.2) | 56 (47.9) | |
II | 11 (9.2) | 13 (11.1) | |
III | 23 (19.3) | 34 (29.1) | |
IV | 11 (9.2) | 14 (12.0) |
NCOR1: nuclear receptor co-repressor 1, MACIS: distant metastasis, patient age, completeness of resection, local invasion, and tumor size, SMRT: silencing mediator for retinoid or thyroid hormone receptor, SD: standard deviation, TCGA: The Cancer Genome Atlas, THCA: thyroid cancer, THRB: thyroid hormone receptor beta, TNM: tumor-node- metastasis
Table 3
Characteristics | NCOR1/THRA n=24 (%) | SMRT/THRB n=24 (%) | p-value |
---|---|---|---|
Age (years) | 48.36±14.56 | 52.78±16.98 | 0.356* |
Gender (Male:Female) | 3 (12.5):21 (87.5) | 10 (41.7):14 (58.3) | 0.023† |
Tumor size (cm) | 2.68±1.50 | 3.02±1.38 | 0.475* |
MACIS score | 5.16±1.24 | 6.05±2.01 | 0.097* |
Histologic subtype | 0.172† | ||
Follicular variant | 4 (18.2) | 1 (4.3) | |
Conventional | 17 (77.3) | 18 (78.3) | |
Tall cell variant | 0 (0.0) | 4 (17.4) | |
Othersς | 1 (4.5) | 0 (0.0) | |
Extrathyroidal extension | 0.028† | ||
No | 19 (82.6) | 12 (52.2) | |
Yes | 4 (17.4) | 11 (47.8) | |
Multifocality | 0.555† | ||
No | 11 (47.8) | 13 (56.5) | |
Yes | 12 (52.2) | 10 (43.5) | |
T stage | 0.038† | ||
T1 | 9 (39.1) | 3 (13.0) | |
T2 | 7 (30.4) | 7 (30.4) | |
T3 | 6 (26.1) | 11 (47.8) | |
T4 | 1 (4.3) | 2 (8.7) | |
N stage | 0.001† | ||
N0 | 17 (81.0) | 7 (31.8) | |
N1 | 4 (19.0) | 15 (68.2) | |
M stage | 0.309† | ||
M0 | 14 (100.0) | 13 (92.9) | |
M1 | 0 (0.0) | 1 (7.1) | |
TNM stage | 0.038† | ||
I | 14 (63.6) | 9 (39.1) | |
II | 3 (13.6) | 2 (8.7) | |
III | 4 (18.2) | 7 (30.4) | |
IV | 1 (4.5) | 5 (21.7) |
NCOR1: nuclear receptor co-repressor 1, MACIS: distant metastasis, patient age, completeness of resection, local invasion, and tumor size, SMRT: silencing mediator for retinoid or thyroid hormone receptor, SD: standard deviation, TCGA: The Cancer Genome Atlas, THCA: thyroid cancer, THRA: thyroid hormone receptor alpha, THRB: thyroid hormone receptor beta, TNM: tumor-node-metastasis
Table 4
Characteristics | NCOR1/THRA n=24 (%) | SMRT/THRB n=24 (%) | p-value |
---|---|---|---|
Ras driver mutation | 0.018† | ||
Absent | 18 (78.3) | 23 (100.0) | |
Present | 5 (21.7) | 0 (0.0) | |
BRAF driver mutation | 0.376† | ||
Absent | 13 (56.5) | 10 (43.5) | |
Present | 10 (43.5) | 13 (56.5) | |
TERT promoter mutation | 0.086† | ||
Absent | 16 (94.1) | 13 (72.2) | |
Present | 1 (5.9) | 5 (27.8) | |
RAS/RAF score | −0.024±0.71 | −0.78±0.23 | <0.0001* |
ERK score | −2.08±18.29 | 24.25±14.04 | <0.0001* |
Differentiation score | 0.37±1.08 | −0.87±1.01 | <0.0001* |
Tumor status | 0.130† | ||
Free | 23 (100.0) | 19 (90.5) | |
With tumor | 0 (0.0) | 2 (9.5) |