Abstract
Porcine epidemic diarrhea virus (PEDV) is a contagious coronavirus infecting pigs that leads to significant economic losses in the swine industry. Given that PEDV infection occurs in gut epithelial cells mainly via the fecal-oral route, induction of PEDV-specific immune responses in the mucosal compartment is required for protective immunity against viral infection. However, an effective mucosal vaccine against the currently prevalent PEDV strain is not available. In this study, we demonstrated that the N-terminal domain (NTD) of the spike (S) protein of PEDV represents a new vaccine candidate molecule to be applied via the mucosal route. We first established an Escherichia coli expression system producing the partial NTD (NTD231–501) of the PEDV S protein. Orally administered NTD231–501 protein specifically interacted with the apical area of M cells in the follicle-associated epithelium of Peyer's patch. Additionally, the NTD protein induced antigen-specific immune responses in both the systemic and mucosal immune compartments when administered orally. Collectively, we propose the NTD of the PEDV S protein to be a candidate mucosal vaccine molecule.
References
2. Pensaert MB, de Bouck P. A new coronavirus-like particle associated with diarrhea in swine. Arch Virol. 1978; 58:243–247.


3. Deng F, Ye G, Liu Q, Navid MT, Zhong X, Li Y, Wan C, Xiao S, He Q, Fu ZF, et al. Identification and comparison of receptor binding characteristics of the spike protein of two porcine epidemic diarrhea virus strains. Viruses. 2016; 8:55.


4. Kocherhans R, Bridgen A, Ackermann M, Tobler K. Completion of the porcine epidemic diarrhoea coronavirus (PEDV) genome sequence. Virus Genes. 2001; 23:137–144.
5. Li BX, Ge JW, Li YJ. Porcine aminopeptidase N is a functional receptor for the PEDV coronavirus. Virology. 2007; 365:166–172.


6. Alonso C, Goede DP, Morrison RB, Davies PR, Rovira A, Marthaler DG, Torremorell M. Evidence of infectivity of airborne porcine epidemic diarrhea virus and detection of airborne viral RNA at long distances from infected herds. Vet Res (Faisalabad). 2014; 45:73.


7. de Arriba ML, Carvajal A, Pozo J, Rubio P. Mucosal and systemic isotype-specific antibody responses and protection in conventional pigs exposed to virulent or attenuated porcine epidemic diarrhoea virus. Vet Immunol Immunopathol. 2002; 85:85–97.


8. Liu C, Tang J, Ma Y, Liang X, Yang Y, Peng G, Qi Q, Jiang S, Li J, Du L, et al. Receptor usage and cell entry of porcine epidemic diarrhea coronavirus. J Virol. 2015; 89:6121–6125.


9. Brandtzaeg P, Kiyono H, Pabst R, Russell MW. Terminology: nomenclature of mucosa-associated lymphoid tissue. Mucosal Immunol. 2008; 1:31–37.


10. Brandtzaeg P, Pabst R. Let's go mucosal: communication on slippery ground. Trends Immunol. 2004; 25:570–577.


11. Kucharzik T, Lügering N, Rautenberg K, Lügering A, Schmidt MA, Stoll R, Domschke W. Role of M cells in intestinal barrier function. Ann N Y Acad Sci. 2000; 915:171–183.


12. Strugnell RA, Wijburg OL. The role of secretory antibodies in infection immunity. Nat Rev Microbiol. 2010; 8:656–667.


13. Frey A, Neutra MR. Targeting of mucosal vaccines to Peyer's patch M cells. Behring Inst Mitt. 1997; 98:376–389.
14. Hase K, Kawano K, Nochi T, Pontes GS, Fukuda S, Ebisawa M, Kadokura K, Tobe T, Fujimura Y, Kawano S, et al. Uptake through glycoprotein 2 of FimH+ bacteria by M cells initiates mucosal immune response. Nature. 2009; 462:226–230.
15. Kim SH, Jung DI, Yang IY, Kim J, Lee KY, Nochi T, Kiyono H, Jang YS. M cells expressing the complement C5a receptor are efficient targets for mucosal vaccine delivery. Eur J Immunol. 2011; 41:3219–3229.


16. Nochi T, Yuki Y, Matsumura A, Mejima M, Terahara K, Kim DY, Fukuyama S, Iwatsuki-Horimoto K, Kawaoka Y, Kohda T, et al. A novel M cell-specific carbohydrate-targeted mucosal vaccine effectively induces antigen-specific immune responses. J Exp Med. 2007; 204:2789–2796.


17. Li X, Chen H. Evaluation of the porcine gastric mucin binding assay for high-pressure-inactivation studies using murine norovirus and tulane virus. Appl Environ Microbiol. 2015; 81:515–521.


18. Chung HC, Lee JH, Nguyen VG, Huynh TM, Lee GE, Moon HJ, Park SJ, Kim HK, Park BK. New emergence pattern with variant porcine epidemic diarrhea viruses, South Korea, 2012–2015. Virus Res. 2016; 226:14–19.


19. Chung HC, Nguyen VG, Moon HJ, Lee JH, Park SJ, Lee GE, Kim HK, Noh YS, Lee CH, Goede D, et al. Isolation of porcine epidemic diarrhea virus during outbreaks in South Korea, 2013–2014. Emerg Infect Dis. 2015; 21:2238–2240.


20. Kim SH, Lee KY, Jang YS. Mucosal immune system and M cell-targeting strategies for oral mucosal vaccination. Immune Netw. 2012; 12:165–175.


21. Gracie JA, Bradley JA. Interleukin-12 induces interferon-gamma-dependent switching of IgG alloantibody subclass. Eur J Immunol. 1996; 26:1217–1221.
22. Gerdts V, Zakhartchouk A. Vaccines for porcine epidemic diarrhea virus and other swine coronaviruses. Vet Microbiol. 2017; 206:45–51.


23. Harper S, Speicher DW. Expression and purification of GST fusion proteins. Curr Protoc Protein Sci. 2008. ;Chapter 6: Unit 6.6.


24. Li W, Luo R, He Q, van Kuppeveld FJ, Rottier PJ, Bosch BJ. Aminopeptidase N is not required for porcine epidemic diarrhea virus cell entry. Virus Res. 2017; 235:6–13.


25. Lycke N. Recent progress in mucosal vaccine development: potential and limitations. Nat Rev Immunol. 2012; 12:592–605.


26. Nochi T, Yuki Y, Katakai Y, Shibata H, Tokuhara D, Mejima M, Kurokawa S, Takahashi Y, Nakanishi U, Ono F, et al. A rice-based oral cholera vaccine induces macaque-specific systemic neutralizing antibodies but does not influence pre-existing intestinal immunity. J Immunol. 2009; 183:6538–6544.


Figure 1.
The NTD231–501 of the PEDV S1 protein contains a sugar-binding domain. Schematic diagram shows the CTD and the NTD of the S protein encompassing amino acids 231–501.
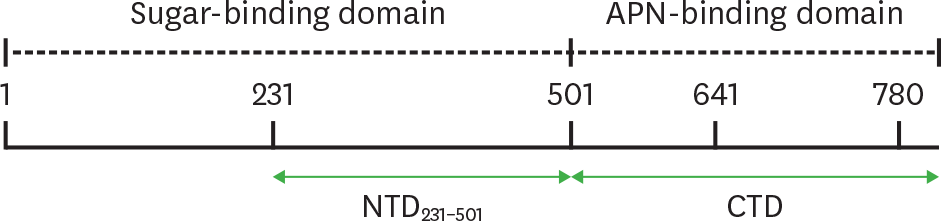
Figure 2.
Expression of the NTD231–501 of the PEDV S1 protein in E. coli. (A) The gene region encoding NTD231–501 was cloned into the pGEX 4T–1 vector containing a GST tag. (B) Recombinant NTD231–501 of the PEDV S1 protein was expressed by isopropyl β-D-1-thiogalactopyranoside induction and confirmed by Western blot analysis using an anti-GST Ab. (C) Recombinant NTD231–501 of the PEDV S1 protein was purified by glutathione Sepharose chromatography.
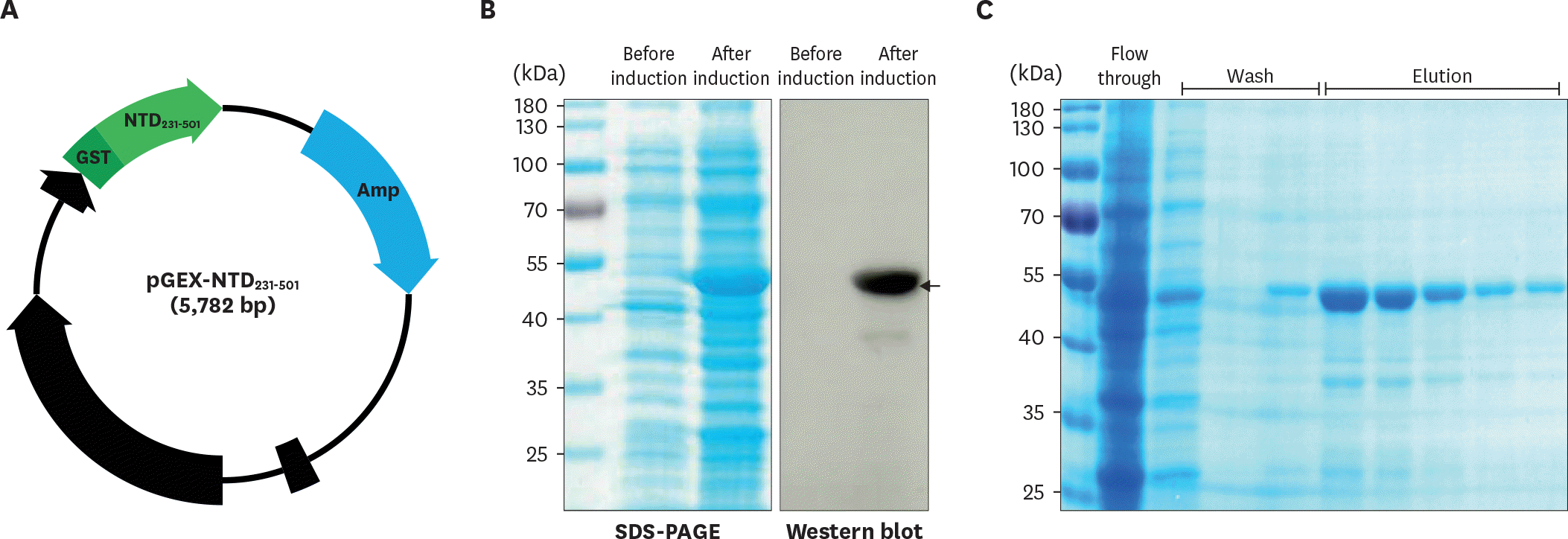
Figure 3.
Recombinant NTD231–501 of the PEDV S1 protein exhibited mucin-binding activity in a mucin-binding assay. (A) Recombinant NTD231–501 of the PEDV S1 protein purified by GST affinity chromatography was incubated on an ELISA plate coated with mucin. Binding activity was determined using an HRP-conjugated anti-GST Ab followed by the addition of tetramethyl benzidine substrate. Numbers on the X-axis indicate the amounts of NTD or control proteins. (B) Whole PP incubated with recombinant NTD231–501 of the PEDV S1 protein purified by GST affinity chromatography was stained with NKM 16-2–4 anti-M cell Ab (Alexa 488, green), anti-GST Ab (Alexa 594, red), or WGA (Alexa 405, blue). Samples were examined by confocal laser scanning microscopy. Dotted lines depict the M cells, and scale bars represent 20 μm. * p<0.05 indicates a significant difference between the NTD231–501 protein and control recombinant protein.
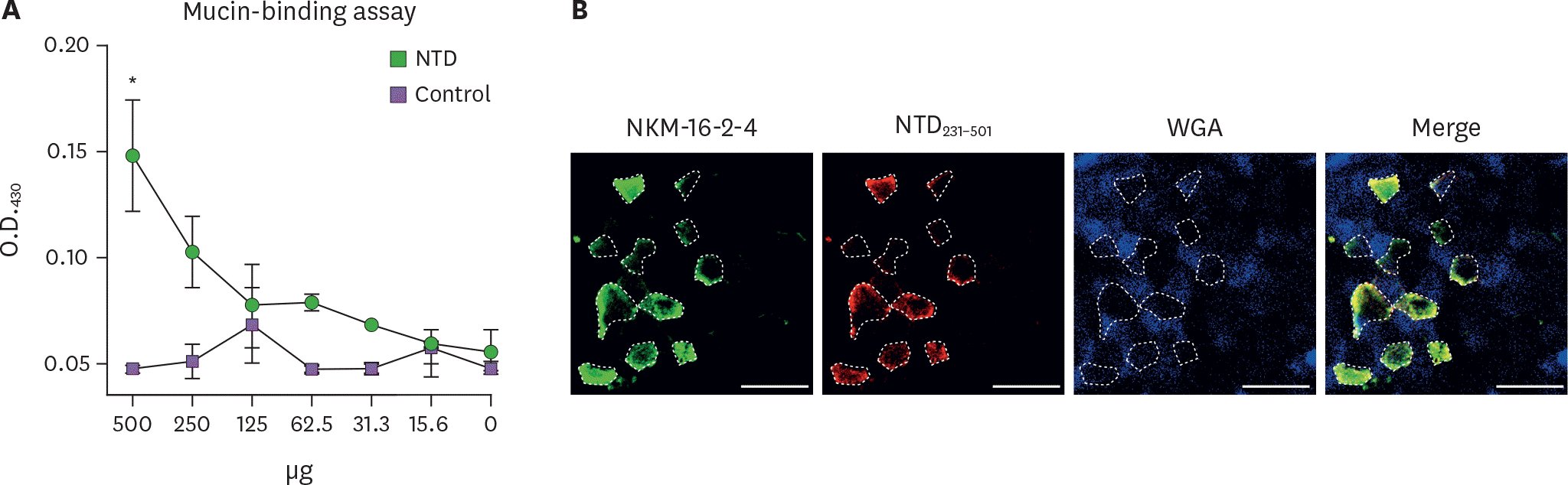
Figure 4.
Oral mucosal immunization of recombinant NTD231–501 of the PEDV S1 protein into mice induces Ag-specific immune responses in the systemic and mucosal compartments. Each group of mice was orally administered PBS, NTD231–501, or NTD231–501 with CT once a week for 3 wk. (A) Sera, (B) feces, and (C) LP cells were prepared at (A and B) 3 days and (C) 4 weeks after the last immunization. (A) The NTD231–501-specific serum IgG isotype and NTD231–501-specific fecal IgA were determined by ELISA. (C) The number of NTD231–501-specific IgA Ab-secreting cells in the LP was determined by ELISPOT assay. Data are presented as means±standard error (n=3), and a representative result from three independent experiments is shown. * p<0.05 indicates a significant difference among the groups compared.
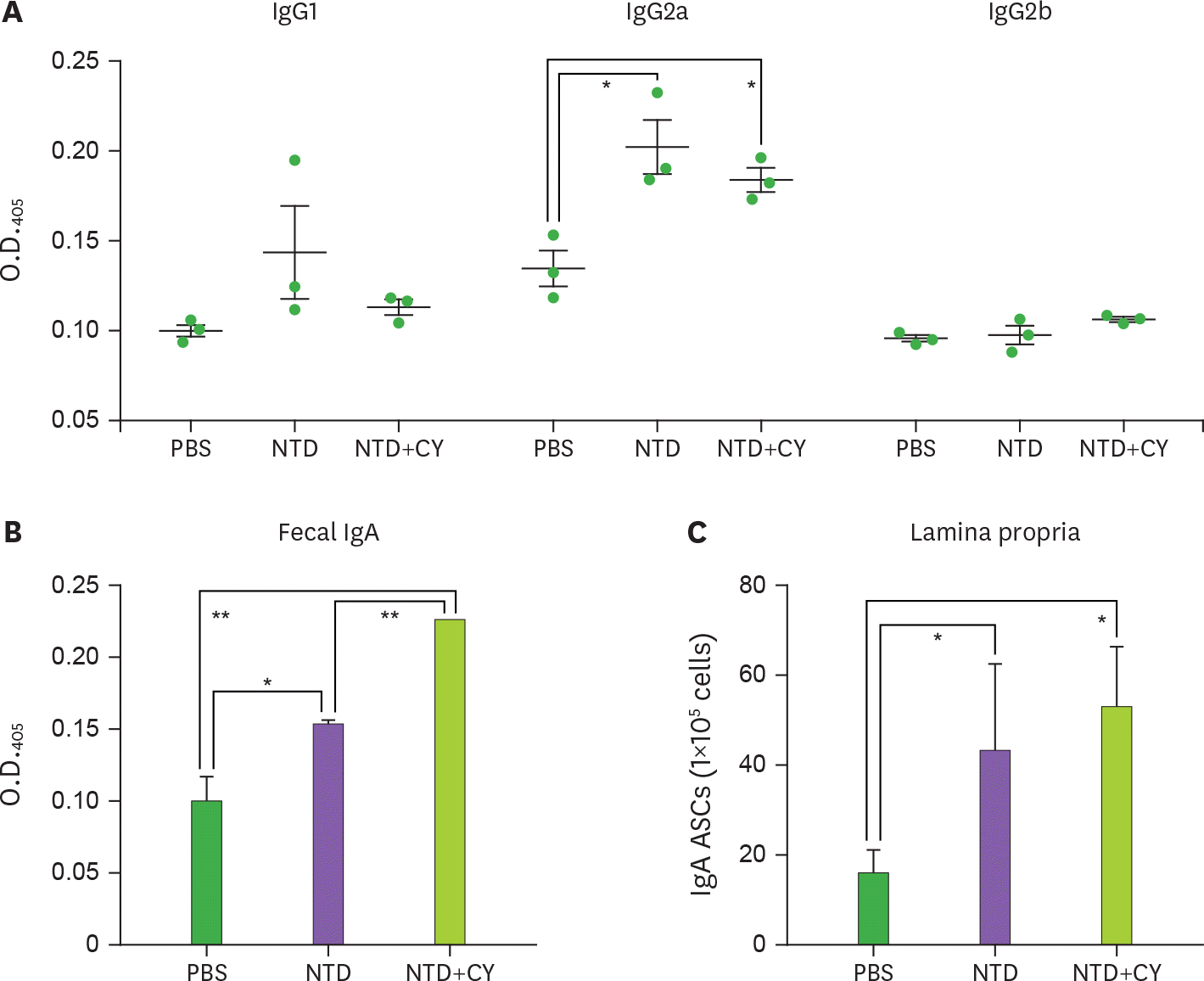