Abstract
Background
The effect of intrauterine hyperglycemia on fat mass and regional fat proportion of the offspring of mothers with gestational diabetes mellitus (OGDM) remains to be determined.
Methods
The body composition of OGDM (n=25) and offspring of normoglycemic mothers (n=49) was compared using dualenergy X-ray absorptiometry at age 5 years. The relationship between maternal glucose concentration during a 100 g oral glucose tolerance test (OGTT) and regional fat mass or proportion was analyzed after adjusting for maternal prepregnancy body mass index (BMI).
Results
BMI was comparable between OGDM and control (median, 16.0 kg/m2 vs. 16.1 kg/m2). Total, truncal, and leg fat mass were higher in OGDM compared with control (3,769 g vs. 2,245 g, P=0.004; 1,289 g vs. 870 g, P=0.017; 1,638 g vs. 961 g, P=0.002, respectively), whereas total lean mass was lower in OGDM (15,688 g vs. 16,941 g, P=0.001). Among OGDM, total and truncal fat mass were correlated with fasting and 3-hour glucose concentrations of maternal 100 g OGTT during pregnancy (total fat mass, r=0.49, P=0.018 [fasting], r=0.473, P=0.023 [3-hour]; truncal fat mass, r=0.571, P=0.004 [fasting], r=0.558, P=0.006 [3-hour]), but there was no correlation between OGDM leg fat mass and maternal OGTT during pregnancy. Regional fat indices were not correlated with concurrent maternal 75 g OGTT values.
Maternal hyperglycemia during pregnancy increases the risk of adverse pregnancy outcomes including macrosomia, premature delivery, and preeclampsia [1,2]. Prenatal exposure to the diabetic milieu leads to hyperinsulinemia in the fetus that may lead to increased adiposity in offspring [3]. Recently, the Hyperglycemia and Adverse Pregnancy Outcomes (HAPO) follow-up study group reported that gestational diabetes mellitus (GDM) identified by International Association of Diabetes and Pregnancy Study Group (IADPSG) criteria is associated with increased total fat percent, sum of skinfolds, and the incidence of obesity in 10- to 14-year-old offspring, after adjusting for maternal body mass index (BMI) during pregnancy [4,5]. In that study, maternal glucose concentrations during pregnancy showed a linear association with offspring adiposity indices including BMI z-score and body fat percent [4,5]. However, higher BMI or obesity in offspring of mothers with gestational diabetes mellitus (OGDM) of prepubertal age were found in some [6-8], but not all studies [9,10].
One reason for the inconsistent findings between maternal hyperglycemia during pregnancy and offspring obesity is that early studies used BMI or sum of skinfolds as an indicator for adiposity, both of which have limitations in assessing total or regional fat proportion separately from lean mass [6,9-11]. Another reason is that many studies did not adjust for the effect of maternal obesity [6,9,10] and notably, adjustment for maternal BMI attenuated most of these associations [5,7,11,12]. Considering the variability of body composition with age in children [13,14], the age range of offspring should be narrow or stratified in order to observe consistent findings.
In this study, we compared the body composition of OGDM with that of offspring of normoglycemic mothers at 5 years of age using dual-energy X-ray absorptiometry (DXA). In addition, we evaluated the correlation between intrauterine hyperglycemia and whole body or regional fat proportion in OGDM.
Informed consent was obtained from the parents and children involved in this study. The Institutional Review Board (IRB) of Seoul National University Bundang Hospital approved the study protocol (IRB No. B-1007-105-007). This study was conducted according to the World Medical Association’s Declaration of Helsinki [15].
Twenty-five OGDM and 49 age-matched control offspring aged 5 years were included in this study. For OGDM, children who were born between December 2003 and August 2006 at Seoul National University Bundang Hospital and whose mothers had GDM and who have been followed after delivery were screened for this study (n=49) [16]. OGDM who did not meet the age criteria (n=19), who had a birth weight of <2.5 or >4.0 kg (n=2), or who did not complete the study (n=3) were excluded. All 25 OGDM were singleton full-term births. The inclusion criteria for control offspring (n=49) were described previously [17]. In brief, 5-year-old Korean children born to mothers without a history of GDM or pregestational diabetes, without chronic disease, not taking medications affecting body composition, and with a BMI between the 5th and 95th percentile, were enrolled in the study between June 2007 and May 2008 (n=50). One subject with incomplete data was excluded. All controls were born at full-term with birth weights of 2.5 to 4.0 kg.
The screening and diagnosis of GDM in mothers were described previously [18]. Briefly, a 50 g glucose challenge test was done at 24 to 28 weeks of gestation. A positive screen was defined as a 1-hour glucose value of 130 mg/dL or greater. Women with a positive screen were given a 3-hour, 100 g oral glucose tolerance test (OGTT). Two or more glucose concentrations over criteria were diagnosed as GDM (fasting, ≥95 mg/dL; 1-hour, ≥180 mg/dL; 2-hour, ≥155 mg/dL; 3-hour, ≥140 mg/dL). In mothers with GDM, the following parameters were also evaluated: maternal prepregnancy BMI, insulin treatment during pregnancy, glucose concentrations from the 100 g OGTT at diagnosis of GDM, and 75 g OGTT at the time of this study (postpartum 5 years). The diagnosis of prediabetes and diabetes was based on American Diabetes Association criteria (prediabetes: fasting, 100 to 125 mg/dL; 2-hour, 140 to 199 mg/dL; glycosylated hemoglobin [HbA1c], 5.7% to 6.4%) (diabetes: fasting, ≥126 mg/dL; 2-hour, ≥200 mg/dL; HbA1c, ≥6.5%) [19].
Birth weight and gender were reviewed in all subjects (offspring). BMI (kg/m2) was calculated from measured height and weight and BMI z-score was assigned according to Korean reference values [20]. A whole body DXA was performed using the Lunar Prodigy advance system with pediatric software (GE Lunar Corporation, General Electric, Madison, WI, USA) according to the standard procedure. Each scan provided total, truncal, and leg fat mass and proportion (%) as well as lean mass and bone mineral content. Height-normalized indices of body composition (kg/m2) were evaluated as fat mass index (FMI), truncal FMI, leg FMI, and lean mass index (LMI) [21]. In OGDM, standard OGTT (1.75 g/kg body weight of glucose solution [maximum 75 g]) was performed and blood samples were obtained at 0, 30, and 120 minutes.
Plasma glucose concentration was measured using the glucose oxidase method (747 clinical chemistry analyzer; Hitachi, Tokyo, Japan). Plasma insulin was measured by radioimmunoassay (Linco, St. Louis, MO, USA). HbA1c was measured using a Bio-Rad Variant II Turbo high-performance liquid chromatography analyzer (Bio-Rad, Hercules, CA, USA).
Insulin sensitivity was assessed using the Matsuda index: 10,000/√(fasting glucose×fasting insulin×mean glucose×mean insulin) [22]. The insulinogenic index was used to estimate insulin secretion: [insulin (30 minutes)–insulin (0 minute)]/[glucose (30 minutes)–glucose (0 minute)] [23]. The disposition index was used to evaluate the composite of insulin secretion considering the degree of insulin sensitivity: Matsuda index×insulinogenic index [24]. The homeostasis model assessment of insulin resistance (HOMA-IR) and β-cell function (HOMA-β) were also calculated [25].
The data were expressed as the median (range) or as the number of subjects (percentage). Differences between OGDM and control were compared using the Mann-Whitney nonparametric U test and the chi-square test or Fisher’s exact test. Spearman correlation test and partial correlation test adjusting for maternal prepregnancy BMI were performed to evaluate the association between body composition of OGDM and maternal glucose values of the 100 g OGTT. All statistical analyses were performed using SPSS software version 21.0 (IBM SPSS Inc., Armonk, NJ, USA). P≤0.05 was considered statistically significant.
A total of 25 mothers with GDM and their offspring were included in the analysis. The median age of mothers with GDM at delivery was 33.7 years and maternal prepregnancy BMI was 22.5 kg/m2. Ten mothers (38.5%) received insulin therapy during pregnancy. At the time of study, eight (32.0%) and five (20.0%) mothers had prediabetes and overt diabetes, respectively (Table 1).
To investigate whether GDM affects offspring adiposity in early childhood, we compared anthropometric phenotypes between OGDM and control at age 5 years. Baseline characteristics including age, gender, birth weight, BMI, and BMI z-scores were comparable between the two groups (Table 2). OGDM had a significantly higher total, truncal, and leg fat mass and higher height-adjusted indices, but lower lean mass and LMI than controls without significant difference in bone mineral content. OGDM whose mothers had insulin treatment during pregnancy had higher BMI compared with OGDM whose mothers did not receive insulin treatment (Supplementary Table 1).
To evaluate whether the extent of maternal hyperglycemia is associated with adiposity in offspring, we analyzed the correlation between maternal glucose concentrations from 100 g OGTT during pregnancy and offspring regional fat mass indices. The correlation was assessed among OGDM because we could not obtain 100 g OGTT data from control mothers. In the unadjusted analysis, fat mass indices did not correlate with maternal prepregnancy BMI or with glucose concentrations in the 100 g OGTT (Supplementary Table 2). After adjusting for maternal prepregnancy BMI, total and truncal fat mass and their proportion were correlated with fasting and 3-hour 100 g OGTT glucose concentrations, respectively. Truncal FMI was associated with fasting, 1-hour, and 3-hour glucose concentrations, area under the curve (AUC) of 100 g OGTT, and HbA1c during pregnancy (Table 3). However, leg fat mass, lean mass, and bone mineral content were not correlated with glucose concentrations in the 100 g OGTT. Maternal glucose concentrations during pregnancy and offspring adiposity indices generally maintained their correlation after adjusting for gender and birth weight. However, no significant correlation between offspring fat indices and concurrent maternal glucose concentration of 75 g OGTT was observed (Supplementary Table 3).
Next, subjects were categorized into tertile groups according to the maternal glucose concentrations during 100 g OGTT. Total fat mass, total fat percentage, and trunk fat percentage were higher across increasing tertiles of maternal fasting, 1-hour, and 3-hour glucose, but not 2-hour glucose (Fig. 1A-C). These findings suggest that the extent of maternal hyperglycemia during pregnancy is associated with whole body and central adiposity of offspring.
We investigated whether maternal hyperglycemia affects glycemic indices of OGDM. Fasting and 3-hour glucose were correlated with HOMA-IR and 3-hour glucose with the Matsuda index. Insulinogenic index was also correlated with fasting and 3-hour glucose, but the disposition index was not (Table 3). The Matsuda index decreased with increasing tertiles of maternal fasting and 3-hour glucose, and compensatory increase in insulinogenic index was also observed (Fig. 1D and E). However, no trend could be found in the disposition index (Fig. 1F).
Offspring adiposity may contribute to their glycemic indices. As expected, total fat mass and proportion were correlated with a decreasing Matsuda index and an increasing insulinogenic index, but not with the disposition index (Supplementary Table 4). These findings suggest that offspring adiposity influenced by maternal hyperglycemia may contribute to both insulin resistance and compensatory insulin secretion without significant change in the composite β-cell function in early childhood.
Prenatal exposure to maternal hyperglycemia leads to fetal hyperinsulinemia [3]. Offspring born from mothers with GDM have a higher incidence of overweight and obesity in some studies [4,5], but this finding was inconsistent [9,10,12]. BMI consists of both fat and lean mass; therefore, we used DXA measurements to compare body composition in OGDM with that in controls. The total, truncal, and leg fat mass were higher in OGDM than in offspring from normoglycemic mothers. Furthermore, the higher the maternal glucose concentrations of a 100 g OGTT, the higher the fat mass of OGDM, which was significant after adjusting for maternal BMI. In particular, truncal fat mass was positively correlated with maternal glucose concentrations of the 100 g OGTT. Also, maternal glucose values during pregnancy and adiposity of offspring were positively associated with offspring’s insulin resistance. Therefore, intrauterine hyperglycemia is associated with increased adiposity, especially central obesity and insulin resistance in OGDM aged 5 years.
BMI reaches a nadir between the ages of 5 and 7 years [14]. Higher birth weight in OGDM than in controls disappears as early as a year after birth [9]. Therefore, it is difficult to detect differences in BMI of OGDM in childhood. In fact, previous studies showed inconsistent findings on BMI of OGDM (no change [3,6,9]; increase in OGDM [7,26]). In the HAPO follow-up study (mean age 11.4 years), maternal hyperglycemia during pregnancy was associated with offspring overweight and obesity [4,5]. However, no significant association between maternal hyperglycemia and obesity could be found in a subgroup analysis of HAPO data in children aged 5 to 7 years [12]. Different timing of adiposity measurements may contribute to inconsistent findings. In our study (median, 5.6 years old), although the BMIs of OGDM and controls did not differ, fat mass and proportions determined by DXA body composition analysis were both significantly higher in OGDM. These results suggest that maternal hyperglycemia during pregnancy shifts offspring body composition from muscle to fat without affecting body weight.
The presence of GDM and intrauterine hyperglycemia resulted in decreased lean mass irrespective of maternal or offspring BMI in this study. This finding is inconsistent with a previous study by Chandler-Laney et al. [26] showing the positive relationship of maternal glucose concentration during pregnancy with both fat and lean mass in children aged 5 to 10 years. Differences in population (OGDM only in our study), age, ethnicity, or adjustment for maternal BMI may explain this discrepancy. It is noteworthy that the increased fat mass, together with decreased lean mass in OGDM in this study represents a highly disadvantageous metabolic phenotype. Abnormal fat accumulation due to intrauterine hyperglycemia may reduce lean mass in OGDM, given that ectopic fat stores lead to destruction of muscle [27]. Taken together, these findings suggest that BMI may not be a good indicator for assessing adiposity or metabolic risk in prepubertal children.
The BMI (median, 16.1 kg/m2) and percent body fat of control offspring (median, 11.6%) in this study represent the upper half and the lower 25th percentile of United States children aged 5 years, respectively [14,28]. These data suggest there is an ethnic difference in fat proportion in childhood (lower in Korean), and the future metabolic impact of percent body fat in the lower range in Asian children warrants further investigation.
Subgroup analysis of HAPO data from Hong Kong reported a significant but modest increase in BMI of OGDM at age 7 years (15.3 kg/m2 in OGDM vs. 15.0 kg/m2 in offspring of normoglycemic mothers) [29]. In our study, no difference in BMI between Korean OGDM and control offspring was observed. These findings strengthen the conclusion that body composition analysis or skinfold thickness rather than BMI might better predict Asian children at risk of obesity and/or future metabolic disorders.
To our knowledge, this is the first study to evaluate body composition of OGDM using DXA among Asian children. The selection of offspring in an age range limited to 5 years allowed us to reduce age-related variability in body composition [13,14]. Another strength of this study was the exclusion of children with low (<2.5 kg) or high (>4.0 kg) birth weight, which allowed us to control for the effect of birth weight on early childhood adiposity. Furthermore, insulin resistance and β-cell function of offspring derived from OGTTs, were evaluated in addition to adiposity of offspring. Although we did not find alterations in glucose concentrations or composite β-cell function of offspring, we observed compensatory insulin secretion (increase in insulinogenic index) along with an increase in insulin resistance in the presence of higher maternal glucose concentrations.
This study has several limitations. First, the small sample size cannot exclude potential selection bias. Second, we could not assess the association between maternal glucose concentration and adiposity in control offspring due to a lack of maternal 100 g OGTT data. Third, only certain glucose concentrations during 100 g OGTT (i.e., fasting and 3-hour) were associated with offspring adiposity indices. The fact that truncal FMI was consistently associated with 1-hour glucose, HbA1c, and AUC of 100 g OGTT, strengthens our observation that intrauterine hyperglycemia is associated with central adiposity of the offspring. Fourth, maternal prepregnancy BMI was not adjusted when body composition was compared between OGDM and controls in this study. Considering that the mean BMI of healthy Korean women aged 30 to 34 years was 22.2 kg/m2 in 2005, which was similar to the BMI of GDM women in this study (median, 22.5 kg/m2) [30], this limitation may not greatly impact our results. Fifth, we could not determine whether increased fat mass at 5 years of age suggests increased fetal growth or early adiposity rebound due to the limitation of the cross-sectional design.
In summary, intrauterine hyperglycemia is associated with increased body fat composition in OGDM aged 5 years. Future mechanistic studies designed to elucidate how in utero exposure to hyperglycemia affects offspring adiposity (i.e., epigenetic, metabolomic) would provide additional insights into transgenerational heritability of metabolic phenotypes.
Supplementary Materials
Supplementary materials related to this article can be found online at https://doi.org/10.4093/dmj.2020.0154.
Supplementary Table 1.
Clinical and anthropometric phenotypes of offspring of mothers with gestational diabetes mellitus of 5 years of age by maternal insulin treatment during pregnancy
Supplementary Table 2.
Correlation between body composition of offspring of mothers with gestational diabetes mellitus and maternal glycemic indices during pregnancy
Supplementary Table 3.
Correlation between body composition of offspring of mothers with gestational diabetes mellitus and concurrent maternal glucose concentration during 75 g OGTT
Supplementary Table 4.
Correlation between body composition of offspring and their glycemic indices
Notes
ACKNOWLEDGMENTS
We thank Hyejin Kim (Seoul National University Bundang Hospital) for assistance with subject enrollment.
REFERENCES
1. HAPO Study Cooperative Research Group, Metzger BE, Lowe LP, Dyer AR, Trimble ER, Chaovarindr U, et al. Hyperglycemia and adverse pregnancy outcomes. N Engl J Med. 2008; 358:1991–2002.


2. Kim MH, Kwak SH, Kim SH, Hong JS, Chung HR, Choi SH, et al. Pregnancy outcomes of women additionally diagnosed as gestational diabetes by the International Association of the Diabetes and Pregnancy Study Groups criteria. Diabetes Metab J. 2019; 43:766–75.


4. Lowe WL Jr, Lowe LP, Kuang A, Catalano PM, Nodzenski M, Talbot O, et al. Maternal glucose levels during pregnancy and childhood adiposity in the Hyperglycemia and Adverse Pregnancy Outcome Follow-up Study. Diabetologia. 2019; 62:598–610.


5. Lowe WL Jr, Scholtens DM, Lowe LP, Kuang A, Nodzenski M, Talbot O, et al. Association of gestational diabetes with maternal disorders of glucose metabolism and childhood adiposity. JAMA. 2018; 320:1005–16.


6. Wright CS, Rifas-Shiman SL, Rich-Edwards JW, Taveras EM, Gillman MW, Oken E. Intrauterine exposure to gestational diabetes, child adiposity, and blood pressure. Am J Hypertens. 2009; 22:215–20.


7. Crume TL, Ogden L, West NA, Vehik KS, Scherzinger A, Daniels S, et al. Association of exposure to diabetes in utero with adiposity and fat distribution in a multiethnic population of youth: the Exploring Perinatal Outcomes among Children (EPOCH) Study. Diabetologia. 2011; 54:87–92.


8. Chandler-Laney PC, Bush NC, Granger WM, Rouse DJ, Mancuso MS, Gower BA. Overweight status and intrauterine exposure to gestational diabetes are associated with children’s metabolic health. Pediatr Obes. 2012; 7:44–52.


9. Whitaker RC, Pepe MS, Seidel KD, Wright JA, Knopp RH. Gestational diabetes and the risk of offspring obesity. Pediatrics. 1998; 101:E9.


10. Boney CM, Verma A, Tucker R, Vohr BR. Metabolic syndrome in childhood: association with birth weight, maternal obesity, and gestational diabetes mellitus. Pediatrics. 2005; 115:e290–6.


11. Philipps LH, Santhakumaran S, Gale C, Prior E, Logan KM, Hyde MJ, et al. The diabetic pregnancy and offspring BMI in childhood: a systematic review and meta-analysis. Diabetologia. 2011; 54:1957–66.


12. Thaware PK, McKenna S, Patterson CC, Hadden DR, Pettitt DJ, McCance DR. Untreated mild hyperglycemia during pregnancy and anthropometric measures of obesity in offspring at age 5-7 years. Diabetes Care. 2015; 38:1701–6.


13. Barlow SE; Expert Committee. Expert committee recommendations regarding the prevention, assessment, and treatment of child and adolescent overweight and obesity: summary report. Pediatrics. 2007; 120 Suppl 4:S164–92.


14. Cole TJ, Lobstein T. Extended international (IOTF) body mass index cut-offs for thinness, overweight and obesity. Pediatr Obes. 2012; 7:284–94.


15. World Medical Association. World Medical Association Declaration of Helsinki. Ethical principles for medical research involving human subjects. Bull World Health Organ. 2001; 79:373–4.
16. Kim E, Kwak SH, Chung HR, Ohn JH, Bae JH, Choi SH, et al. DNA methylation profiles in sibling pairs discordant for intrauterine exposure to maternal gestational diabetes. Epigenetics. 2017; 12:825–32.


17. Lim JS, Hwang JS, Cheon GJ, Lee JA, Kim DH, Park KD, et al. Gender differences in total and regional body composition changes as measured by dual-energy X-ray absorptiometry in Korean children and adolescents. J Clin Densitom. 2009; 12:229–37.


18. Moon JH, Kwak SH, Jung HS, Choi SH, Lim S, Cho YM, et al. Weight gain and progression to type 2 diabetes in women with a history of gestational diabetes mellitus. J Clin Endocrinol Metab. 2015; 100:3548–55.


19. American Diabetes Association. 2. Classification and diagnosis of diabetes: standards of medical care in diabetes-2020. Diabetes Care. 2020; 43(Suppl 1):S14–31.
20. Moon JS, Lee SY, Nam CM, Choi JM, Choe BK, Seo JW, et al. 2007 Korean national growth charts: review of developmental process and an outlook. Korean J Pediatr. 2008; 51:1–25.


21. VanItallie TB, Yang MU, Heymsfield SB, Funk RC, Boileau RA. Height-normalized indices of the body’s fat-free mass and fat mass: potentially useful indicators of nutritional status. Am J Clin Nutr. 1990; 52:953–9.


22. Matsuda M, DeFronzo RA. Insulin sensitivity indices obtained from oral glucose tolerance testing: comparison with the euglycemic insulin clamp. Diabetes Care. 1999; 22:1462–70.


23. Tura A, Kautzky-Willer A, Pacini G. Insulinogenic indices from insulin and C-peptide: comparison of beta-cell function from OGTT and IVGTT. Diabetes Res Clin Pract. 2006; 72:298–301.


24. Retnakaran R, Qi Y, Goran MI, Hamilton JK. Evaluation of proposed oral disposition index measures in relation to the actual disposition index. Diabet Med. 2009; 26:1198–203.


25. Matthews DR, Hosker JP, Rudenski AS, Naylor BA, Treacher DF, Turner RC. Homeostasis model assessment: insulin resistance and beta-cell function from fasting plasma glucose and insulin concentrations in man. Diabetologia. 1985; 28:412–9.
26. Chandler-Laney PC, Bush NC, Rouse DJ, Mancuso MS, Gower BA. Maternal glucose concentration during pregnancy predicts fat and lean mass of prepubertal offspring. Diabetes Care. 2011; 34:741–5.


27. Unger RH. Minireview: weapons of lean body mass destruction: the role of ectopic lipids in the metabolic syndrome. Endocrinology. 2003; 144:5159–65.


28. Laurson KR, Eisenmann JC, Welk GJ. Body fat percentile curves for U. S. children and adolescents. Am J Prev Med. 2011; 41(4 Suppl 2):S87–92.
29. Tam WH, Ma RCW, Ozaki R, Li AM, Chan MHM, Yuen LY, et al. In utero exposure to maternal hyperglycemia increases childhood cardiometabolic risk in offspring. Diabetes Care. 2017; 40:679–86.


30. Ministry of Health and Welfare: The Third Korea National Health and Nutrition Examination Survey (KNHANES III). Available from: https://knhanes.cdc.go.kr (cited 2021 Jan 12).
Fig. 1.
Offspring adiposity and glycemic indices across categories of maternal glucose levels during pregnancy. (A) Total fat mass, (B) total fat %, (C) truncal fat %, (D) Matsuda index, (E) insulinogenic index, and (F) disposition index. Offspring of mothers with gestational mellitus diabetes (n=25) were divided into tertiles of maternal glucose concentrations of 100 g oral glucose tolerance test (OGTT) during pregnancy. Glucose categories of 100 g OGTT during pregnancy are defined as follows: fasting glucose (category 1, <90 mg/dL; category 2, 90 to 101 mg/dL; category 3, >101 mg/dL); 1-hour glucose (category 1, <190 mg/dL; category 2, 190 to 209 mg/dL; category 3, >209 mg/dL); 2-hour glucose (category 1, <165 mg/dL; category 2, 165 to 184 mg/dL; category 3, >184 mg/dL); 3-hour glucose (category 1, <140 mg/dL; category 2, 140 to 158 mg/dL; category 3, >158 mg/dL). Error bar represents standard error of mean.
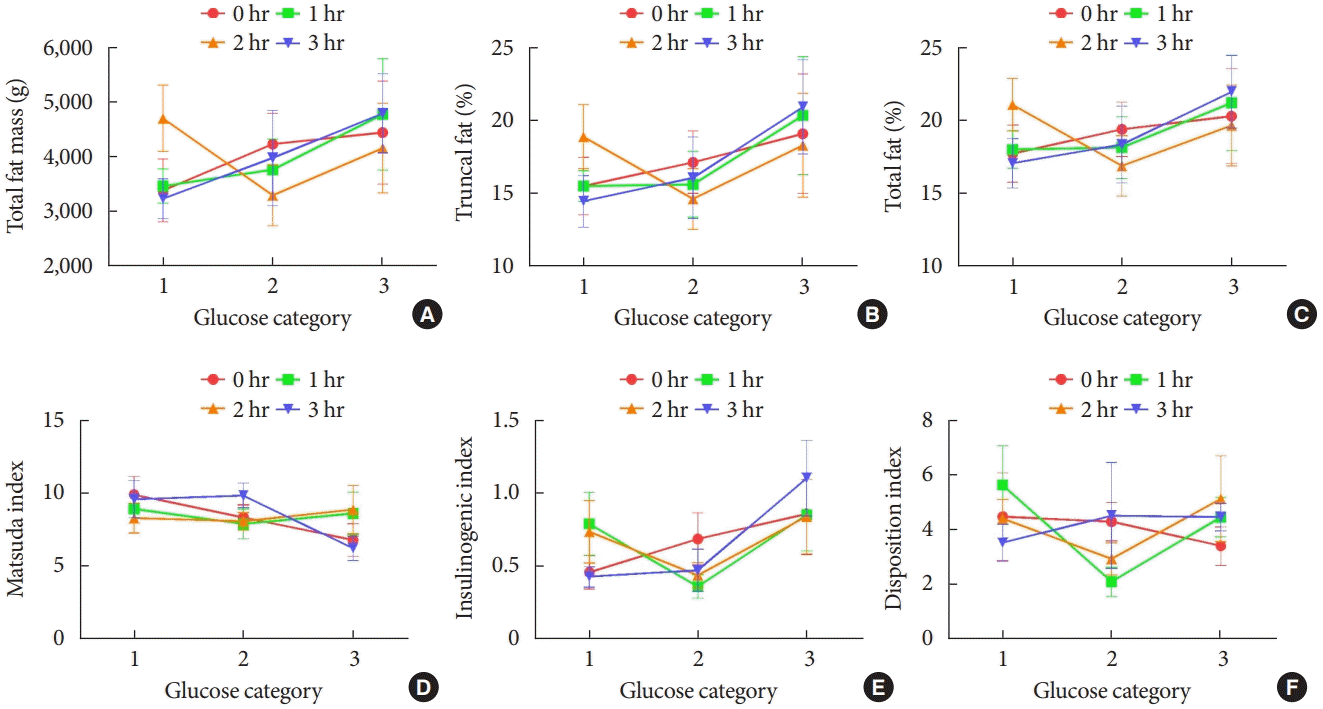
Table 1.
Metabolic characteristics of mothers with gestational diabetes mellitus and their offspring
Table 2.
Clinical and anthropometric phenotypes between offspring of mothers with gestational diabetes mellitus and control of 5 years of age
Variable | OGDM (n=25) | Control (n=49) | P value |
---|---|---|---|
Sex, male:female | 12:13 | 26:23 | 0.806 |
Age at study, yr | 5.6 (5.00–6.70) | 5.9 (5.00–6.70) | 0.087 |
Birth weight, kg | 3.34 (2.81–4.00) | 3.15 (2.58–4.00) | 0.084 |
Height, cm | 112.9 (101.7–128.0) | 114.0 (105.0–128.0) | 0.407 |
Weight, kg | 20.5 (14.6–28) | 21.1 (17.0–33.9) | 0.219 |
BMI, kg/m2 | 16.0 (14.1–18.4) | 16.1 (13.7–23.1) | 0.273 |
BMI z-score | 0.33 (–1.16 to 1.53) | 0.37 (–1.57 to 2.96) | 0.285 |
DXA body composition | |||
Total fat mass, g | 3,769 (1,600–9,315) | 2,245 (1,037–9,795) | 0.004a |
Total fat, % | 18.0 (9.9–36.6) | 11.6 (4.7–33.4) | <0.001a |
FMI, kg/m2 | 2.68 (1.40–7.28) | 1.75 (0.68–7.73) | 0.002a |
Truncal fat mass, g | 1,289 (578–4,615) | 870 (314–5,292) | 0.017a |
Truncal fat, % | 16.0 (8.1–40.5) | 10.0 (4.0–36.0) | 0.002a |
Truncal FMI, kg/m2 | 1.00 (0.49–3.61) | 0.67 (0.24–4.22) | 0.009a |
Leg fat mass, g | 1,638 (606–3,674) | 961 (333–3,850) | 0.002a |
Leg fat, % | 23.5 (12.9–39.0) | 15.4 (4.9–35.7) | <0.001a |
Leg FMI, kg/m2 | 1.17 (0.53–2.47) | 0.75 (0.22–2.48) | 0.001a |
Lean mass, g | 15,688 (11,291–19,566) | 16,941 (14,522–21,943) | 0.001a |
LMI, kg/m2 | 12.1 (10.9–13.2) | 13.0 (11.6–15.2) | <0.001a |
BMC, g | 675 (488–846) | 698 (487–991) | 0.081 |
Table 3.
Correlation between body composition of offspring of mothers with gestational diabetes mellitus and maternal glycemic indices during pregnancy
Variable | Value |
100 g OGTT |
HbA1c | ||||
---|---|---|---|---|---|---|---|
Fasting | 1-hr | 2-hr | 3-hr | AUC | |||
Total fat mass, g | r | 0.49 | 0.337 | –0.074 | 0.473 | 0.298 | 0.371 |
P | 0.018a | NC | NC | 0.023a | NC | 0.081 | |
Total fat, % | r | 0.463 | 0.336 | –0.029 | 0.466 | 0.313 | 0.361 |
P | 0.026a | NC | NC | 0.025a | NC | 0.091 | |
FMI, kg/m2 | r | 0.524 | 0.381 | 0.01 | 0.504 | 0.367 | 0.407 |
P | 0.01a | 0.073 | NC | 0.014a | 0.085 | 0.054 | |
Truncal fat mass, g | r | 0.571 | 0.401 | 0.028 | 0.558 | 0.402 | 0.427 |
P | 0.004a | 0.058 | NC | 0.006a | 0.057 | 0.042a | |
Truncal fat, % | r | 0.52 | 0.397 | 0.042 | 0.545 | 0.397 | 0.408 |
P | 0.011a | 0.061 | NC | 0.007a | 0.061 | 0.053 | |
Truncal FMI, kg/m2 | r | 0.593 | 0.429 | 0.098 | 0.582 | 0.454 | 0.452 |
P | 0.003a | 0.041a | NC | 0.004a | 0.03a | 0.03a | |
Leg fat mass, g | r | 0.376 | 0.23 | –0.217 | 0.336 | 0.141 | 0.279 |
P | 0.077 | NC | NC | NC | NC | NC | |
Leg fat, % | r | 0.378 | 0.245 | –0.112 | 0.352 | 0.197 | 0.281 |
P | 0.075 | NC | NC | 0.099 | NC | NC | |
Leg FMI, kg/m2 | r | 0.423 | 0.285 | –0.135 | 0.371 | 0.216 | 0.328 |
P | 0.044a | NC | NC | 0.081 | NC | NC | |
Lean mass, g | r | 0.074 | –0.141 | –0.277 | 0.077 | –0.164 | 0.122 |
P | NC | NC | NC | NC | NC | NC | |
LMI, kg/m2 | r | 0.071 | –0.009 | –0.051 | –0.094 | –0.038 | 0.191 |
P | NC | NC | NC | NC | NC | NC | |
BMC, g | r | 0.264 | 0.017 | –0.104 | 0.37 | 0.074 | 0.34 |
P | NC | NC | NC | 0.082 | NC | NC | |
AUC (1.75 g/kg OGTT) | r | 0.172 | 0.171 | 0.216 | 0.32 | 0.228 | 0.093 |
P | NC | NC | NC | NC | NC | NC | |
HOMA-IR | r | 0.414 | 0.178 | –0.061 | 0.429 | 0.28 | 0.41 |
P | 0.05a | NC | NC | 0.046a | NC | 0.052 | |
HOMA-β | r | –0.201 | –0.104 | –0.064 | 0.208 | –0.045 | –0.083 |
P | NC | NC | NC | NC | NC | NC | |
Matsuda index | r | –0.366 | –0.225 | 0.011 | –0.504 | –0.298 | –0.346 |
P | 0.086 | NC | NC | 0.017a | NC | NC | |
Insulinogenic index | r | 0.587 | 0.269 | 0.275 | 0.67 | 0.475 | 0.411 |
P | 0.003a | NC | NC | 0.001a | 0.025a | 0.052 | |
Disposition index | r | 0.216 | 0.041 | 0.226 | 0.296 | 0.199 | 0.213 |
P | NC | NC | NC | NC | NC | NC |
Correlation coefficient was calculated by partial correlation test adjusting for maternal prepregnancy body mass index.
OGTT, oral glucose tolerance test; AUC, area under the curve; HbA1c, glycosylated hemoglobin; NC, not correlated; FMI, fat mass index; LMI, lean mass index; BMC, bone mineral content; HOMA-IR, homeostasis model assessment value of insulin resistance; HOMA-β, homeostasis model assessment value of β-cell function.