Abstract
Aging is associated with structural and functional changes of the cornea. Fresh onion juice contains phenolic compounds and flavonoids that may provide an anti-aging effect. The aim of this study was to assess the ability of the onion juice to ameliorate these aging changes. Rats were grouped as adult and aged groups. Rats of both groups received eye drops of diluted onion juice in their right eyes every 8 hours for 12 weeks, while the left ones were served as control eyes. The corneas of both eyes underwent histopathological, immunohistochemical and morphometric assessments, in addition to measuring their intraocular pressure (IOP). The aged group exhibited a significantly elevated IOP, decreased tear secretion, degenerated corneal epithelium and endothelium, surface erosions and stromal edema with irregular collagen fibers. Administration of onion juice led to lowering of IOP, significant increase in tearing, restoration of most of epithelial, endothelial and stromal integrity, and increased epithelial, keratocystic and endothelial cell densities. Immunohistochemically, the epithelium and endothelium revealed positive immune reactions for both epidermal growth factor receptor (EGFR) and paired box protein-6 (PAX6) in the control and onion-treated corneas of the adult group, while these immune reactions were negative in the untreated aged ones. Onion drops in aged corneas showed a positive immune reaction for EFGR and PAX6 involving the epithelial and endothelial layers. In conclusion, topical onion juice improves corneal aging changes through its direct effect, and indirectly through lowering IOP and enhancing tear secretion.
Health care of the elderly became an emerging priority due to increased life expectancy, motivating the directions toward understanding our aging process [1]. Aging is associated with progressive accumulating tissue damage or, in other words; reduces normal tissue mass via both intrinsic and extrinsic metabolic processes [2]. The eye is a vital organ and is our window to the outer world, thus deteriorating its functions due to aging significantly harms the daily lifestyle of the aged people [3]. It consists of highly specialized tissues to provide the ability of precise vision [4]. The cornea is a part of our eye that is characterized by being transparent and avascular; serving many functions by acting as an anterior light refractive surface, protective blocking structure for the majority of ultraviolet rays and also providing a mechanical barrier against infections [5].
Aging process leads to structural alterations in the visual system affecting the corneal epithelium, stroma and endothelium [6]. These changes affect the corneal ability for light refraction and increase the eye vulnerability to infection. These aging changes of the cornea mainly include increased corneal epithelial permeability and gradually decreased corneal endothelial cell number leaving the cornea at risk for hypoxic stress [7]. In addition, irreversible age-induced endothelial cell loss affects the corneal hydration state. This leads to fluid accumulation and corneal edema losing the corneal transparency [8]. Furthermore, age is contributed to diminished levels and composition of tears secretion by the lacrimal gland leading to loss of homeostasis of the ocular surface that induces tear film instability and hyperosmolarity, and finally inflammation of the ocular surfaces [9, 10].
Onion (Allium cepa) is cultivated worldwide as a bulbous herb belonging to the Alliaceae family. For centuries, it has been used for prevention and treatment of a wide variety of diseases due to its benefits for health [11]. Onion has various concentrations of phenolic compounds and flavonoids. Fundamentally, these flavonoids in onion are glucosides and quercetin aglycone, in addition to kaempferol monoglycoside or isorhamnetin monoglycoside in some species. Due to its compounds, onion extracts show anti-aging, anti-oxidant, anti-inflammatory, anti-bacterial, anti-diarrhoeal, anti-mutagenic, anti-cancer, anti-spasmodic, anti-ulcer, and anti-proliferative properties [12-14]. One of the most important compounds of onion is quercetin that exhibits antioxidant and/or pro-oxidant as well as modulates different protective intracellular signaling cascades [15]. Moreover, onion contains 1-propenyl sulfenic acid that is converted into lachrymatory factor by the action of lachrymatory factor synthase, and this lachrymatory factor is responsible for inducing tearing in onion [16]. The tear film is critical for ocular surface comfort, environmental, mechanical and immune protection, promoting corneal epithelial health and nutrition, and providing a smooth, refracting surface for clear vision [17].
Previous studies demonstrated the beneficial effects of onion extracts on different structures of the eye. For example, Kim et al. [18] found that topical use of onion extract ointment prevents corneal haze caused by air-assisted lamellar keratectomy, in addition to Javadzadeh et al. [19] who concluded that topical diluted fresh onion juice prevents sodium-selenite induced cataract formation. Furthermore, Nejabat et al. [20] revealed that fresh onion juice topical drops application in rabbits has an inhibitory effect on the growth of normal eye flora and thus they reported that onion has antimicrobial properties to treat common eye infections. Nevertheless, Owji et al. [21] demonstrated that topical onion extract has no significant effect on blepharoplasty scar prevention.
The aim of this work was to evaluate histological, immunohistochemical and morphometric alterations of the cornea related to aging and assess the possible preventive effect of the topical onion juice on these alterations.
Thirty healthy male Sprague-Dawley rats were purchased from Animal House of Faculty of Veterinary Medicine, Suez Canal University, Ismailia, Egypt. The rats were exposed to a 12/12 hour daily light-dark cycle, kept in a controlled temperature of 20°C–22°C and humidity of 40%–60%, and allowed for free access of food and water ad libitum. All animal experiments were conducted regarding the National Institutes of Health guide for the care and use of laboratory animals (NIH Publications No. 8023, revised 1978).
All rats were allowed for 10 days for acclimatization, then were randomly divided into 2 equal groups (n=15 per group) as following:
Group I (adult group): included rats aged 5 to 6 months that received topical one diluted onion juice drop in the right eye, every 8 hours daily for 12 weeks [19], while left eyes received one distilled water drop every 8 hours daily for 12 weeks and served as the control ones.
Group II (aged group): included rats aged 22 to 24 months that were administrated topical one diluted onion juice drop in the right eye, every 8 hours daily for 12 weeks [19], while left eyes received one distilled water drop every 8 hours daily for 12 weeks conducted as the control ones.
Fresh onion (Allium cepa Linn) was purchased from the local market in Ismailia City, Egypt. Every 3 days, 150 g of the onion bulbs was cut into small pieces and then crushed in a mixing machine. The resultant crushed mixture was squeezed and filtered through a fine cloth [22]. The resultant filtrate was about 70 ml every time that was then diluted with the same volume of distilled water and quickly frozen until used. The prepared juice was utilized for only 3 days then the remaining unused juice was discarded [19].
A TonoLab rebound tonometer (Tono-Pen; TiolatOy, Helsinki, Finland) was used to measure the IOP of both eyes of all rats at the start of the experiment and then once weekly. Light anesthesia was induced by 2% to 4% isoflurane inhalation to reduce fluctuations of IOP caused by stress and movements. Measurements were performed only between 10 A.M. and 2 P.M. to minimize bias that may be caused by the circadian rhythm. Six consecutive readings were recorded, and their average was calculated for each eye [23].
Tear secretion was weekly measured in both eyes for each rat in both groups after 10 minutes of the topical application of onion juice by utilizing a modified Schirmer test. In this test, a filter paper strip (1-mm width and 20-mm length) was inserted 1 mm in the lower conjunctival fornix of the eye for 5 minutes. Finally, length of the wet portion of the paper was measured in millimeters [24].
After 3 months, all rats were euthanized by an overdose of intraperitoneal sodium pentobarbital. Both eyes of each rat were carefully enucleated and submersed into 10% paraformaldehyde then paraffin blocks were prepared. Sections of 4-μm thickness were cut to be stained with hematoxylin and eosin (H&E) and periodic acid–Schiff (PAS) stains for microscopic assessment of the cornea [25]. The transverse sections were made up to the level of the optic nerve to assess the central zone of the cornea.
Immunohistochemistry was performed to determine antigens of epidermal growth factor receptor (EGFR), as a promoter for corneal epithelial homeostasis, and paired box protein-6 (PAX6), as an indicator of corneal morphogenesis.
Paraffin-embedded specimens were deparaffinized and rehydrated. For EGFR immunohistochemistry, endogenous peroxidase activity was blocked by immersion in 0.6% hydrogen peroxide then antigen retrieval was achieved by protein kinase K. Later, sections were incubated with mouse monoclonal anti-EGFR antibody (Zymed, San Francisco, CA, USA) (1:100 dilution), as a primary antibody, overnight at 4°C. Goat anti-mouse HRP-conjugated immunoglobulin (Invitrogen; Thermo Fisher Scientific Inc., Waltham, MA, USA) (1:200 dilution) was used as a secondary antibody. 3,3’-diaminobenzidine was used for visualization of the immunoreaction and finally, sections were counterstained by Mayer’s hematoxylin [26].
For PAX6 immunohistochemistry, antigen retrieval was performed by microwave treatment for 20 minutes in Tris–EDTA buffer (pH 9.0), then blocking of the endogenous peroxidase activity was achieved by 3% hydrogen peroxide solution treatment for 10 minutes at room temperature. Subsequently, sections were incubated with rabbit anti-PAX6 polyclonal antibody (1:500) overnight at 4°C, and then incubated with Polymer Helper and poly-HRP anti-Rabbit IgG (1:200 dilution) at 37°C for 30 minutes immunostaining positive cells visualization was by using diaminobenzidine tetrahydrochloride solution (Zhongshan Golden Bridge Biotechnology, Beijing, China) and then counterstaining was achieved by hematoxylin [27].
Morphometric measurements were carried out using the ImageJ® software (Wayne Rasband NIH, Bethesda, MA, USA) at a magnification of ×400 including at least 5 sections per animal, and from each section, 5 different regions were observed. Only one investigator performed the measurements to avoid inter-observer errors. Morphometric measurements included: total corneal, epithelial layer, Bowman’s layer and stromal thicknesses in H&E-stained sections, in addition to the Descemet’s membrane thickness in PAS-stained sections.
Data analysis was done using Statistical Package for Social Sciences (SPSS) software version 27.0 for Windows (IBM Corp., Armonk, NY, USA). For comparison between multiple means of the study groups, analysis of variance (ANOVA) was used followed by Tukey post-hoc test. Results were expressed as the mean±standard deviation.
The control eyes of the aged group showed a significant increase in the level of IOP compared to those of both eyes of the adult one throughout the experiment (P<0.05). On the other hand, the level of IOP of treated eyes of the aged group showed a significant decrease compared to the control aged ones starting from the week 3 till week 12 (P<0.05) (Fig. 1).
Throughout the experiment, there was a significant increase in the tear secretion of the treated eyes in both groups compared to those of the control eyes (P<0.05). Moreover, there was a significant decrease in the tear secretion in the control eyes of the aged group compared to that of the adult one (P<0.05; Fig. 2).
Sections of H&E stain of the cornea in both eyes of the adult group consisted of 5 distinct layers from outside inwards: stratified nonkeratinized squamous epithelium, Bowman’s layer with delicate collagen fibers, stroma with regular layers of collagen fibers and keratocytes, homogenous Descemet’s membrane, and an endothelial layer (Fig. 3A, B). On the contrary, the control corneas of the aged group showed irregular surface with multiple small surface erosions, thinning of the epithelial layer with flat degenerated nuclei and loss of the nuclei in the epithelial layer specially in the superficial zones. The stroma exhibited massively irregular collagen fibers with wide spaces, areas of edema, in addition to degeneration of most of the endothelial cells (Fig. 3C). The treated corneas of the aged group revealed regular corneal surface, restoration of most of the normal features of the epithelial cells and their nuclei mainly in the superficial regions. In addition, the stroma showed mostly regular collagen fibers with narrow spaces and the lining endothelium was arranged in a continuous layer (Fig. 3D).
Regarding PAS stain sections, the basal lamina of the epithelium and Descemet’s membrane of the adult group were clearly identified with PAS stain with more thickened Descemet’s membrane in the treated cornea (Fig. 4A, B). On the other hand, the control corneas of the aged group showed that the basal lamina of the epithelium was mostly absent, while Descemet’s membrane appeared normal in thickness (Fig. 4C). The treated corneas of the aged group revealed that the basal lamina was mostly apparent in most of regions, however, Descemet’s membrane was markedly thickened (Fig. 4D).
Immunohistochemical staining of EGFR of the control cornea of the adult group showed an extensive immune expression in the basal layer of epithelium and corneal endothelium, with a minimal expression in superficial layers of the epithelium (Fig. 5A). Nonetheless, this expression in the treated cornea of the adult group was minimal in the basal layer of the epithelium and endothelium and was moderate in superficial layers of the epithelium (Fig. 5B). Moreover, the control cornea of the aged group revealed an almost negative EGFR immune reaction in all layers (Fig. 5C). The treated cornea of the aged group showed an extensive immune reaction in both superficial layers of the epithelium and endothelium, however, there was a minimal reaction in the basal layer of epithelium (Fig. 5D).
The control cornea of the adult group demonstrated a minimal PAX6 immune reaction in the basal epithelial and endothelial layers (Fig. 6A), whereas this was negative in the treated cornea of the same group (Fig. 6B). In the control cornea of the aged group, PAX6 immune reaction was negative in its all layers (Fig. 6C), while a minimal immune expression in the superficial epithelial layers and endothelium was demonstrated in the treated cornea of the aged group (Fig. 6D).
Total corneal and stromal layer thicknesses were significantly increased in the control cornea of the aged group compared to that of the adult group ones (P<0.05), while was significantly decreased in treated corneas of the aged group compared to the control ones of the same group (P<0.05). In contrast, epithelial and Bowman’s layer thicknesses were significantly decreased in the control cornea of the aged group compared to the adult group (P<0.05), while they were significantly increased in the treated cornea of the aged group compared to the control cornea of the same group (P<0.05). Whereas the Descemet’s membrane thickness was significantly increased in treated corneas of the adult and aged groups compared to the control ones of these groups (P<0.05; Table 1).
Regarding the cell densities of different corneal layers, epithelial, keratocystic and endothelial cell densities were significantly decreased in the control cornea of the aged group compared to that of the adult one (P<0.05), while significantly increased in the treated cornea of the aged group compared to the control cornea of the same group (P<0.05) (Table 2).
The current study assessed the ability of topical onion juice to ameliorate age-related corneal changes based on histopathological, immunohistochemical and histomorphometric examinations. Despite their limited number, previous experimental studies assessed the effects of onion extracts on corneal changes related to different animal models [18, 28], in addition to the effects of onion extracts on the pathological changes of other ocular structures [19, 29, 30]. Furthermore, several publications indicated that age has major changes on the cornea [31-35]. In accordance with our results, El-Sayyad et al. [33] and Halawa [34] found that the cornea of aged rats exhibited atrophied epithelium with small dark nuclei, hyalinization of the stromal collagen fibrils, keratocystic loss and degeneration of the lining endothelium. In contrast to our results, Tananuvat and Khumchoo [36] demonstrated that there is a significant inverse correlation between age and central corneal thickness in humans.
Despite continuous exposure of the eye to oxidative stress, the corneal epithelium, under normal conditions, is rich in antioxidant enzymes that can counteract the reactive oxygen species (ROS). As a result, corneal epithelial turnover is continuous and thus, cumulative senescence may occur [7]. The present study revealed that there is a significant decrease in the epithelial cell density and central corneal epithelial layer thickness with age in rats. Nonetheless and with disagreement with our results, Yang et al. [37] proved that central corneal epithelial thickness remains constant in humans with aging.
Bowman’s layer’s functions may be serving as a protective barrier for corneal stroma, and sharing in the maintenance of corneal transparency and epithelial innervation [38]. We observed that there is a significant decline in the Bowman’s layer thickness in aged corneas compared to the adult ones. This may be due to the fact that Bowman’s layer is devoid of keratocytes so its collagen turnover rates may be slow. Also, this thinning may be due to natural collagen degradation with age. Keratocytes are the main cellular component of the corneal stroma and they are considered the main key of extracellular matrix synthesis and turnover in the corneal stroma [39]. In the harmony with our results, Patel et al. [40], Niederer et al. [41], and Gambato et al. [42] concluded that there is a significant inverse relationship between age and keratocystic count in humans.
Descemet’s membrane plays a key role in the collimation of light; in addition to maintaining endothelial cell differentiation, proliferation and structural integrity [43]. Our study showed no aging change in Descemet’s membrane thickness in our study results; however, Jun et al. [43] reported that, in C57BL/6 mice, Descemet’s membrane thickness significantly increases by the age. Furthermore, Johnson et al. [44] concluded the same results but in the humans. Corneal endothelial cells have a critical function by acting as a pump that maintains stromal dehydration, thus maintaining corneal transparency [45, 46]. It was not proven that they could proliferate in vivo, thus, senescence-like changes can affect them with age [46]. In the harmony with our results, almost all studies reported that there is a significant inverse correlation between age and endothelial cell density in rodents and humans [36, 42, 43, 46, 47].
Our study concluded that there was a significant increase in IOP in aged rats. Zhang et al. [48] found the same results but in rabbit model. However, Dubicanac et al. [49] and Sandhas et al. [50] revealed that no aging effect on IOP in the gray mouse lemur or cats respectively. In addition, Melamed et al. [51] found that rabbits with artificially raised IOP develop diffuse corneal haziness and edema and massive corneal endothelial damage. It was believed that prolonged exposure of intact corneal endothelium to high IOP can lead to endothelial damage with a resultant diffuse corneal edema [51]. Elevated IOP may have these adverse effects on corneal endothelium either by direct pressure or indirectly through flow turbulence of aqueous humor [52]. On the other hand, if high IOP is controlled, corneal endothelium can be structurally and functionally recovered [53].
Quercetin is the major component of the onion bulb and one of its biological activities is decreasing the production of ROS via various mechanisms, it can also retain an anti-inflammatory effect and antioxidant potentials [54-56]. Moreover, quercetin can chelate Cu2+ and Fe2+ to exert an antioxidant role. Also, the interaction between quercetin and a variety of enzymes such as cyclooxygenase (COX), phosphodiesterase, lipoxygenase and tyrosine kinase mediates anti-inflammatory properties, fundamentally by inhibition of COX-1 and -2 expressions [57]. Recently it was found that quercetin can also directly interact with DNA. Thus, it has been reported that quercetin may protect against oxidation-induced DNA damage [58]. In addition, quercetin is capable of modification of epigenetic regulations by inhibiting DNA methylation and by influencing histone acetylation [59].
In this study, the daily treatment using onion juice drops was found to significantly decrease the age-related degenerative changes of the cornea. In accordance with these results, Kim et al. [18] revealed that, in dogs, 1% onion extract ointment due to slowing down of fibroblast differentiation into myofibroblasts. Kliuiev and Kolomiichuk [60] used eye quercetin drops in rabbits after ethyl alcohol-induced corneal damage that was also shown to significantly decrease the total corneal thickness and stromal edema.
EGFR signaling is an essential factor for the proliferation, differentiation, and survival of corneal epithelium. This event seems to be due to the activation of MAPK/ERK1/2 pathway [61]. In the present study, we found that EGFR immune expression in the corneal epithelium and endothelium was negative in untreated aged corneas, on the contrary its expression was extensively positive in the treated aged ones. In a rat model of debridement corneal wounds, Zieske et al. [62] found that EGFR expression was gradually increased after 3 hours after corneal injury with complete wound healing approximately 24 hours later and adding tyrphostin AG 1478, a specific inhibitor of EGFR kinase activity, inhibited epithelial cell migration. Thus, Zieske et al. [62] concluded that EGFR has a critical role in the maintenance of the corneal epithelial integrity.
Limbal epithelial stem cells (LESCs) are responsible for corneal epithelial regeneration. PAX6 is a key gene necessary for corneal epithelial integrity and regeneration via maintenance of the main characteristics of these cells and their direction toward the corneal epithelial cell lineage. However, PAX6 is extensively expressed in the developing cornea, this expression continues to some extent into the adult one [63, 64]. Mort et al. [64] used Pax6+/− mice as a model for low PAX6 ocular levels in which they observed that there were severe epithelial and endothelial defects shown by electron microscopic examination. In our study, the epithelial and endothelial histological changes related to the aged cornea were associated with negative immune detection of PAX6 in all layers and this immune expression was positive in the aged cornea with onion treatment. This refers to that onion juice could have a role in the activation of LESCs through PAX6 signaling pathways promoting epithelial regeneration in the aged cornea.
In the current study, the Schirmer test revealed that there is a significant increase in tearing when topical onion drops were applied. The precorneal tear film is responsible for the integrity of the ocular surface by ensuring an optically uniform corneal surface, flushing foreign matter and cellular debris from the cornea, providing a corneal nutritional function, and exerting an antibacterial function [65]. Tearing is stimulated by the lachrymatory factor compound of onion [66]. Epidermal growth factor (EGF) is one of the components of the tears, so the tears are important for normal corneal health [67]. This role is achieved by binding of EGF to its receptors enhancing the activity of intrinsic tyrosine kinase receptor, inducing DNA synthesis and production of fibronectin and hyaluronic acid content of extracellular matrix [68]. Furthermore, the hepatocyte growth factor is also a component of the tears that have a critical role in the maintenance of corneal epithelial health [69].
Regarding onion juice treatment, the observed results in the current study showed clearly notable beneficial improvement effects on most of the aged corneal parameters compared to that of the untreated aged ones. However, when we compared the control and onion juice-treated corneal parameters of the young group, there were limited changes. This may be explained by absence of the pathological alterations in the control young corneas to be improved, so their data were mostly similar.
The ameliorative effect of onion juice on aged cornea had been illustrated in the current study; however, the limitations of this study that could be assessed in the future are the direct effects of quercetin, as a potent antioxidant compound of onion, on the aged cornea. Furthermore, the isolated effect of tears on aged cornea through applying of purified lachrymatory factor stimulating tears secretion can be studied through forthcoming researches. In addition, physiological tests can be applied to assess the visual acuity in rats to examine the exact actual improvement effect level.
In conclusion, the application of topical onion juice is capable to improve aging alterations in the cornea of the rat. This may be due to its antioxidant effects; in addition to through activation of EGFR and PAX6, moreover, its lachrymatory factor component that enhances tear secretion, and has an IOP-lowering effect.
Notes
References
1. Greenwood MP, Greenwood M, Romanova EV, Mecawi AS, Paterson A, Sarenac O, Japundžić-Žigon N, Antunes-Rodrigues J, Paton JFR, Sweedler JV, Murphy D. 2018; The effects of aging on biosynthetic processes in the rat hypothalamic osmoregulatory neuroendocrine system. Neurobiol Aging. 65:178–91. DOI: 10.1016/j.neurobiolaging.2018.01.008. PMID: 29494864. PMCID: PMC5878011.


2. Lee B, Jung JH, Kim HS. 2012; Assessment of red onion on antioxidant activity in rat. Food Chem Toxicol. 50:3912–9. DOI: 10.1016/j.fct.2012.08.004. PMID: 22902824.


3. Mookherjee S, Bhattacharjee A, Sengupta M. 2015; The aging eye. J Ophthalmol. 2015:832326. DOI: 10.1155/2015/832326. PMID: 25878895. PMCID: PMC4387951.


4. Lin JB, Tsubota K, Apte RS. 2016; A glimpse at the aging eye. NPJ Aging Mech Dis. 2:16003. DOI: 10.1038/npjamd.2016.3. PMID: 28721262. PMCID: PMC5515005.


5. idhar MS Sr. 2018; Anatomy of cornea and ocular surface. Indian J Ophthalmol. 66:190–4. DOI: 10.4103/ijo.IJO_1005_17. PMID: 29676317. PMCID: PMC5939165.


6. Salvi SM, Akhtar S, Currie Z. 2006; Ageing changes in the eye. Postgrad Med J. 82:581–7. DOI: 10.1136/pgmj.2005.040857. PMID: 16954455. PMCID: PMC2585730.


7. Faragher RG, Mulholland B, Tuft SJ, Sandeman S, Khaw PT. 1997; Aging and the cornea. Br J Ophthalmol. 81:814–7. DOI: 10.1136/bjo.81.10.814. PMID: 9486017. PMCID: PMC1722015.


8. Gipson IK. 2013; Age-related changes and diseases of the ocular surface and cornea. Invest Ophthalmol Vis Sci. 54:ORSF48–53. DOI: 10.1167/iovs.13-12840. PMID: 24335068.


9. Obata H. 2006; Anatomy and histopathology of the human lacrimal gland. Cornea. 25(10 Suppl 1):S82–9. DOI: 10.1097/01.ico.0000247220.18295.d3. PMID: 17001201.


10. Aragona P, Giannaccare G, Mencucci R, Rubino P, Cantera E, Rolando M. 2021; Modern approach to the treatment of dry eye, a complex multifactorial disease: a P.I.C.A.S.S.O. board review. Br J Ophthalmol. 105:446–53. DOI: 10.1136/bjophthalmol-2019-315747. PMID: 32703782. PMCID: PMC8005804.
11. Mete R, Oran M, Topcu B, Oznur M, Seber ES, Gedikbasi A, Yetisyigit T. 2016; Protective effects of onion (Allium cepa) extract against doxorubicin-induced hepatotoxicity in rats. Toxicol Ind Health. 32:551–7. DOI: 10.1177/0748233713504807. PMID: 24193056.


12. Lee SU, Lee JH, Choi SH, Lee JS, Ohnisi-Kameyama M, Kozukue N, Levin CE, Friedman M. 2008; Flavonoid content in fresh, home-processed, and light-exposed onions and in dehydrated commercial onion products. J Agric Food Chem. 56:8541–8. DOI: 10.1021/jf801009p. PMID: 18759442.


13. Nan L, Huang J, Li Y, Cui C, Peng G, Xie B, Liu H, Liu Y. 2019; Study on the extraction and antioxidant properties of flavonoids from onion. AIP Conf Proc. 2079:020005. DOI: 10.1063/1.5092383.


14. Wilson EA, Demmig-Adams B. 2007; Antioxidant, anti-inflammatory, and antimicrobial properties of garlic and onions. Nutr Food Sci. 37:178–83. DOI: 10.1108/00346650710749071.


15. Upadhyay RK. 2016; Nutraceutical, pharmaceutical and therapeutic uses of Allium cepa: a review. Int J Green Pharm. 10(Suppl):S46–64.
16. Eady CC, Kamoi T, Kato M, Porter NG, Davis S, Shaw M, Kamoi A, Imai S. 2008; Silencing onion lachrymatory factor synthase causes a significant change in the sulfur secondary metabolite profile. Plant Physiol. 147:2096–106. DOI: 10.1104/pp.108.123273. PMID: 18583530. PMCID: PMC2492635.


17. Dartt DA, Willcox MD. 2013; Complexity of the tear film: importance in homeostasis and dysfunction during disease. Exp Eye Res. 117:1–3. DOI: 10.1016/j.exer.2013.10.008. PMID: 24280033. PMCID: PMC4225770.


18. Kim S, Park YW, Lee E, Park SW, Park S, Noh H, Kim JW, Seong JK, Seo K. 2016; Effect of onion extract on corneal haze suppression after air assisted lamellar keratectomy. J Vet Med Sci. 78:419–25. DOI: 10.1292/jvms.15-0455. PMID: 26607134. PMCID: PMC4829509.


19. Javadzadeh A, Ghorbanihaghjo A, Bonyadi S, Rashidi MR, Mesgari M, Rashtchizadeh N, Argani H. 2009; Preventive effect of onion juice on selenite-induced experimental cataract. Indian J Ophthalmol. 57:185–9. DOI: 10.4103/0301-4738.49391. PMID: 19384011. PMCID: PMC2683439.


20. Nejabat M, Salehi A, Noorani Azad P, Ashraf MJ. 2014; Effects of onion juice on the normal flora of eyelids and conjunctiva in an animal model. Jundishapur J Microbiol. 7:e9678. DOI: 10.5812/jjm.9678. PMID: 25147716. PMCID: PMC4138639.


21. Owji N, Khalili MR, Khademi B, Shirvani M, Sadati MS. 2020; Comparison of the effectiveness of onion extract, topical steroid, and petrolatum emollient in cosmetic appearance of upper blepharoplasty scar. J Curr Ophthalmol. 32:408–13. DOI: 10.4103/JOCO.JOCO_39_20. PMID: 33553845. PMCID: PMC7861112.
22. El-Demerdash FM, Yousef MI, El-Naga NI. 2005; Biochemical study on the hypoglycemic effects of onion and garlic in alloxan-induced diabetic rats. Food Chem Toxicol. 43:57–63. DOI: 10.1016/j.fct.2004.08.012. PMID: 15582196.


23. Yu H, Zhong H, Chen J, Sun J, Huang P, Xu X, Huang S, Zhong Y. 2020; Efficacy, drug sensitivity, and safety of a chronic ocular hypertension rat model established using a single intracameral injection of hydrogel into the anterior chamber. Med Sci Monit. 26:e925852. DOI: 10.12659/MSM.925852. PMID: 32997651. PMCID: PMC7534505.


24. Dias AC, Módulo CM, Jorge AG, Braz AM, Jordão AA Jr, Filho RB, de Paula JS, Rocha EM. 2007; Influence of thyroid hormone on thyroid hormone receptor beta-1 expression and lacrimal gland and ocular surface morphology. Invest Ophthalmol Vis Sci. 48:3038–42. DOI: 10.1167/iovs.06-1309. PMID: 17591870.
25. Bancroft JD, Gamble M. 2008. Theory and practice of histological techniques. 6th ed. Churchill Livingstone Elsevier;Philadelphia: p. 72.
26. Hwang HB, Oh TH, Kim HS. 2013; Effect of ethanol-treated mid-peripheral epithelium on corneal wound healing in rabbits. BMC Ophthalmol. 13:27. DOI: 10.1186/1471-2415-13-27. PMID: 23822645. PMCID: PMC3703275.


27. Lei H, Liu H, Ding Y, Ge L. 2014; Immunohistochemical localization of Pax6 in the developing tooth germ of mice. J Mol Histol. 45:373–9. DOI: 10.1007/s10735-014-9564-5. PMID: 24477661.


28. Burgess D, Zhang Y, Siefker E, Vaca R, Kuracha MR, Reneker L, Overbeek PA, Govindarajan V. 2010; Activated Ras alters lens and corneal development through induction of distinct downstream targets. BMC Dev Biol. 10:13. DOI: 10.1186/1471-213X-10-13. PMID: 20105280. PMCID: PMC2828409.


29. Wang G, Chen P, Wang Y, Wang Y, Reinach PS, Xue Y, Liu Z, Li C. 2020; Onion epithelial membrane scaffolds transfer corneal epithelial layers in reconstruction surgery. Adv Healthc Mater. 9:e2000469. DOI: 10.1002/adhm.202000469. PMID: 32548957.


30. Kumar S, Modgil S, Bammidi S, Minhas G, Shri R, Kaushik S, Singh V, Anand A. 2020; Allium cepa exerts neuroprotective effect on retinal ganglion cells of pterygopalatine artery (PPA) ligated mice. J Ayurveda Integr Med. 11:489–94. DOI: 10.1016/j.jaim.2019.08.002. PMID: 32088091. PMCID: PMC7772493.


31. Pradeep SR, inivasan K Sr. 2018; Ameliorative influence of dietary fenugreek (Trigonella foenum-graecum) seeds and onion (Allium cepa) on eye lens abnormalities via modulation of crystallin proteins and polyol pathway in experimental diabetes. Curr Eye Res. 43:1108–18. DOI: 10.1080/02713683.2018.1484146. PMID: 29856678.


32. Inomata T, Mashaghi A, Hong J, Nakao T, Dana R. 2017; Scaling and maintenance of corneal thickness during aging. PLoS One. e0185694. DOI: 10.1371/journal.pone.0185694. PMID: 28985226. PMCID: PMC5630165.


33. El-Sayyad HIH, El-Mansi AA, Guida MS, Mohammed EA. 2015; Markers characterizing corneal damage during aging of rat. J Adv Chem. 11:3532–9. DOI: 10.24297/jac.v11i5.4471.


34. Halawa AM. 2011; Age-associated changes in the cornea, lens and retina of the albino rat eye: a histological and immuno-histochemical study. Egyptian J Anat. 34:1–13. DOI: 10.21608/ejana.2011.3649.


35. Baroody RA, Bito LZ, DeRousseau CJ, Kaufman PL. 1987; Ocular development and aging. 1. Corneal endothelial changes in cats and in free-ranging and caged rhesus monkeys. Exp Eye Res. 45:607–22. DOI: 10.1016/S0014-4835(87)80070-2. PMID: 3428387.


36. Tananuvat N, Khumchoo N. 2020; Corneal thickness and endothelial morphology in normal Thai eyes. BMC Ophthalmol. 20:167. DOI: 10.1186/s12886-020-01385-1. PMID: 32345246. PMCID: PMC7187506.


37. Yang Y, Hong J, Deng SX, Xu J. 2014; Age-related changes in human corneal epithelial thickness measured with anterior segment optical coherence tomography. Invest Ophthalmol Vis Sci. 55:5032–8. DOI: 10.1167/iovs.13-13831. PMID: 25052994.


38. Lagali N, Germundsson J, Fagerholm P. 2009; The role of Bowman's layer in corneal regeneration after phototherapeutic keratectomy: a prospective study using in vivo confocal microscopy. Invest Ophthalmol Vis Sci. 50:4192–8. DOI: 10.1167/iovs.09-3781. PMID: 19407024.
39. Zhou Q, Wang Y, Yang L, Wang Y, Chen P, Wang Y, Dong X, Xie L. 2008; Histone deacetylase inhibitors blocked activation and caused senescence of corneal stromal cells. Mol Vis. 14:2556–65. PMID: 19122829. PMCID: PMC2613076.
40. Patel S, McLaren J, Hodge D, Bourne W. 2001; Normal human keratocyte density and corneal thickness measurement by using confocal microscopy in vivo. Invest Ophthalmol Vis Sci. 42:333–9. PMID: 11157863.
41. Niederer RL, Perumal D, Sherwin T, McGhee CN. 2007; Age-related differences in the normal human cornea: a laser scanning in vivo confocal microscopy study. Br J Ophthalmol. 91:1165–9. DOI: 10.1136/bjo.2006.112656. PMID: 17389741. PMCID: PMC1954900.
42. Gambato C, Longhin E, Catania AG, Lazzarini D, Parrozzani R, Midena E. 2015; Aging and corneal layers: an in vivo corneal confocal microscopy study. Graefes Arch Clin Exp Ophthalmol. 253:267–75. DOI: 10.1007/s00417-014-2812-2. PMID: 25311652.
43. Jun AS, Chakravarti S, Edelhauser HF, Kimos M. 2006; Aging changes of mouse corneal endothelium and Descemet's membrane. Exp Eye Res. 83:890–6. DOI: 10.1016/j.exer.2006.03.025. PMID: 16777092.


44. Johnson DH, Bourne WM, Campbell RJ. 1982; The ultrastructure of Descemet's membrane. I. Changes with age in normal corneas. Arch Ophthalmol. 100:1942–7. DOI: 10.1001/archopht.1982.01030040922011. PMID: 7150061.
45. Xiao X, Wang Y, Gong H, Chen P, Xie L. 2009; Molecular evidence of senescence in corneal endothelial cells of senescence-accelerated mice. Mol Vis. 15:747–61. PMID: 19381346. PMCID: PMC2669445.
46. Cheng H, Jacobs PM, McPherson K, Noble MJ. 1985; Precision of cell density estimates and endothelial cell loss with age. Arch Ophthalmol. 103:1478–81. DOI: 10.1001/archopht.1985.01050100054017. PMID: 4051849.


47. Jorge J, Queirós A, Blasco TF, González-Méijome JM. Peixoto de Matos SC. 2010; Age-related changes of corneal endothelium in normal eyes with a non-contact specular microscope. J Emmetropia. 1:132–9.
48. Zhang H, Qin X, Cao X, Zhang D, Li L. 2017; Age-related variations of rabbit corneal geometrical and clinical biomechanical parameters. Biomed Res Int. 2017:3684971. DOI: 10.1155/2017/3684971. PMID: 29104870. PMCID: PMC5574220.


49. Dubicanac M, Joly M, Strüve J, Nolte I, Mestre-Francés N, Verdier JM, Zimmermann E. 2018; Intraocular pressure in the smallest primate aging model: the gray mouse lemur. Vet Ophthalmol. 21:319–27. DOI: 10.1111/vop.12434. PMID: 27624923.


50. Sandhas E, Merle R, Eule JC. 2018; Consider the eye in preventive healthcare- ocular findings, intraocular pressure and Schirmer tear test in ageing cats. J Feline Med Surg. 20:1063–71. DOI: 10.1177/1098612X17742528. PMID: 29172875.
51. Melamed S, Ben-Sira I, Ben-Shaul Y. 1980; Corneal endothelial changes under induced intraocular pressure elevation: a scanning and transmission electron microscopic study in rabbits. Br J Ophthalmol. 64:164–9. DOI: 10.1136/bjo.64.3.164. PMID: 7387948. PMCID: PMC1039380.


52. Janson BJ, Alward WL, Kwon YH, Bettis DI, Fingert JH, Provencher LM, Goins KM, Wagoner MD, Greiner MA. 2018; Glaucoma-associated corneal endothelial cell damage: a review. Surv Ophthalmol. 63:500–6. DOI: 10.1016/j.survophthal.2017.11.002. PMID: 29146208.


53. Yu ZY, Wu L, Qu B. 2019; Changes in corneal endothelial cell density in patients with primary open-angle glaucoma. World J Clin Cases. 7:1978–85. DOI: 10.12998/wjcc.v7.i15.1978. PMID: 31423429. PMCID: PMC6695540.


54. Beesk N, Perner H, Schwarz D, George E, Kroh LW, Rohn S. 2010; Distribution of quercetin-3,4'-O-diglucoside, quercetin-4'-O-monoglucoside, and quercetin in different parts of the onion bulb (Allium cepa L.) influenced by genotype. Food Chem. 122:566–71. DOI: 10.1016/j.foodchem.2010.03.011.


55. Boots AW, Wilms LC, Swennen EL, Kleinjans JC, Bast A, Haenen GR. 2008; In vitro and ex vivo anti-inflammatory activity of quercetin in healthy volunteers. Nutrition. 24:703–10. DOI: 10.1016/j.nut.2008.03.023. PMID: 18549926.
56. Ozgen S, Kilinc OK, Selamoglu Z. 2016; Antioxidant activity of quercetin: a mechanistic review. Turkish J Agric Food Sci Technol. 4:1134–8. DOI: 10.24925/turjaf.v4i12.1134-1138.1069.


57. Zhao L, Wang H, Du X. 2021; The therapeutic use of quercetin in ophthalmology: recent applications. Biomed Pharmacother. 137:111371. DOI: 10.1016/j.biopha.2021.111371. PMID: 33561647.


58. Song X, Wang Y, Gao L. 2020; Mechanism of antioxidant properties of quercetin and quercetin-DNA complex. J Mol Model. 26:133. DOI: 10.1007/s00894-020-04356-x. PMID: 32399900.


59. Xiao X, Shi D, Liu L, Wang J, Xie X, Kang T, Deng W. 2011; Quercetin suppresses cyclooxygenase-2 expression and angiogenesis through inactivation of P300 signaling. PLoS One. 6:e22934. DOI: 10.1371/journal.pone.0022934. PMID: 21857970. PMCID: PMC3152552.


60. Kliuiev GO, Kolomiichuk SG. 2017; The research of the effect of eye drops containing quercetin for regeneration of the cornea in the experiment. J Tradit Med Clin Natur. 6:222. DOI: 10.4172/2573-4555.1000222.
61. Maugeri G, D'Amico AG, Castrogiovanni P, Saccone S, Federico C, Reibaldi M, Russo A, Bonfiglio V, Avitabile T, Longo A, D'Agata V. 2019; PACAP through EGFR transactivation preserves human corneal endothelial integrity. J Cell Biochem. 120:10097–105. DOI: 10.1002/jcb.28293. PMID: 30548314.


62. Zieske JD, Takahashi H, Hutcheon AE, Dalbone AC. 2000; Activation of epidermal growth factor receptor during corneal epithelial migration. Invest Ophthalmol Vis Sci. 41:1346–55. PMID: 10798649.
63. Davis J, Duncan MK, Robison WG Jr, Piatigorsky J. 2003; Requirement for Pax6 in corneal morphogenesis: a role in adhesion. J Cell Sci. 116(Pt 11):2157–67. DOI: 10.1242/jcs.00441. PMID: 12692153.


64. Mort RL, Bentley AJ, Martin FL, Collinson JM, Douvaras P, Hill RE, Morley SD, Fullwood NJ, West JD. 2011; Effects of aberrant Pax6 gene dosage on mouse corneal pathophysiology and corneal epithelial homeostasis. PLoS One. 6:e28895. DOI: 10.1371/journal.pone.0028895. PMID: 22220198. PMCID: PMC3248408.


65. Chen HB, Yamabayashi S, Ou B, Tanaka Y, Ohno S, Tsukahara S. 1997; Structure and composition of rat precorneal tear film. A study by an in vivo cryofixation. Invest Ophthalmol Vis Sci. 38:381–7. PMID: 9040471.
66. Cramer CS, Mandal S, Sharma S, Nourbakhsh SS, Goldman I, Guzman I. 2021; Recent advances in onion genetic improvement. Agronomy. 11:482. DOI: 10.3390/agronomy11030482.


67. Wilson SE, Lloyd SA, Kennedy RH. 1991; Epidermal growth factor messenger RNA production in human lacrimal gland. Cornea. 10:519–24. DOI: 10.1097/00003226-199111000-00010. PMID: 1723672.


68. Kang SS, Li T, Xu D, Reinach PS, Lu L. 2000; Inhibitory effect of PGE2 on EGF-induced MAP kinase activity and rabbit corneal epithelial proliferation. Invest Ophthalmol Vis Sci. 41:2164–9. PMID: 10892858.
69. Wilson SE, Liu JJ, Mohan RR. 1999; Stromal-epithelial interactions in the cornea. Prog Retin Eye Res. 18:293–309. DOI: 10.1016/S1350-9462(98)00017-2. PMID: 10192515.


Fig. 1
Changes of IOP of study groups (n=15). Values are presented as means. IOP, intraocular pressure. aP<0.05 vs. control and treated eyes of adult group; bP<0.05 vs. control eye of aged group as determined by a one-way ANOVA followed by Tukey’s post-hoc test.
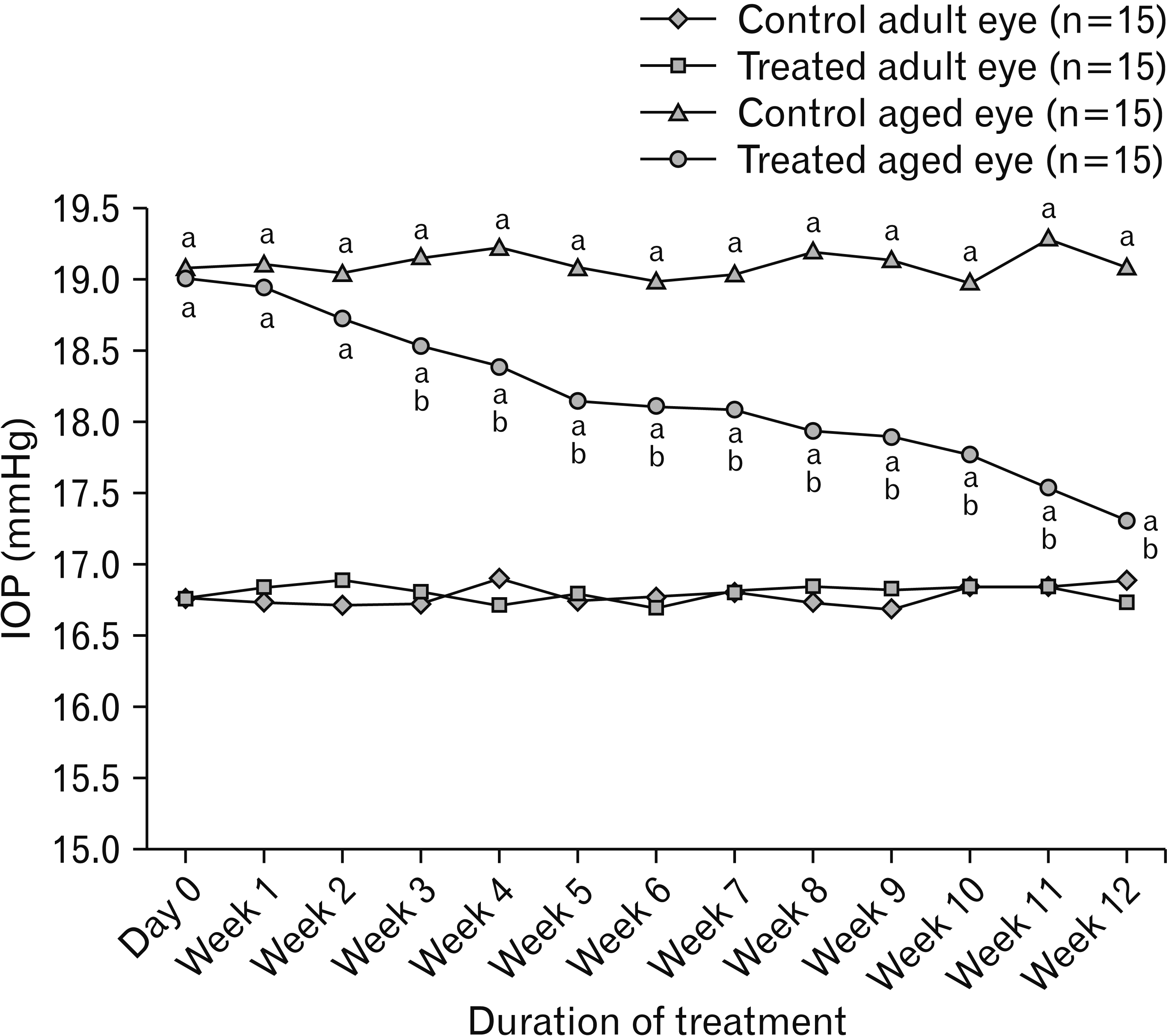
Fig. 2
Modified Schirmer test results of study groups (n=15). Values are presented as means. aP<0.05 vs. control eye of adult group; bP<0.05 vs. treated eye of adult group, cP<0.05 vs. control eye of aged group as determined by a one-way ANOVA followed by Tukey’s post-hoc test.
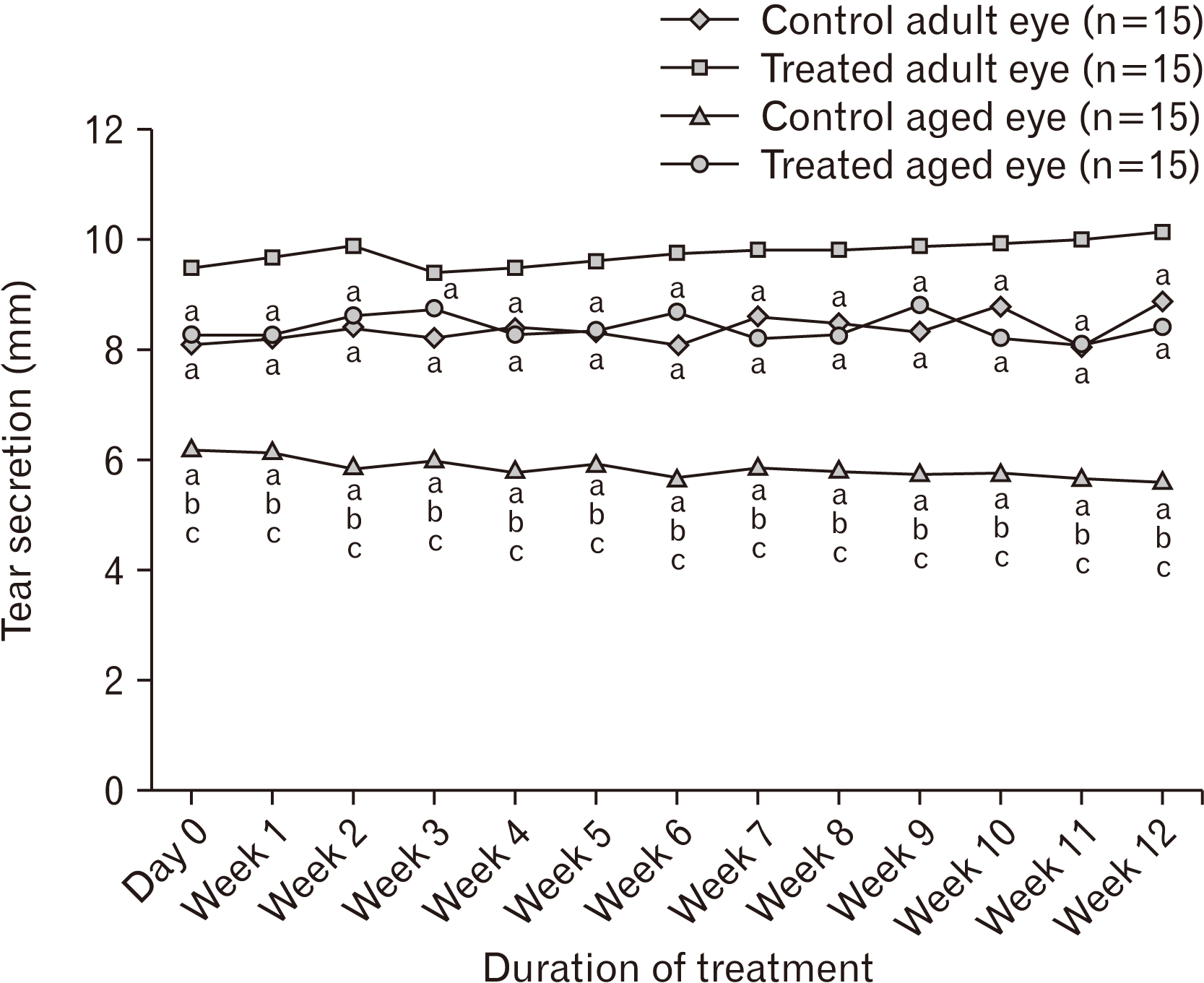
Fig. 3
Photomicrographs of sections of corneas of different study groups. (A, B) Control and treated corneas of the adult group respectively showing normally arranged 5 layers; stratified nonkeratinized epithelium (Ep), Bowman’s layer (B), stroma (S) with keratocytes (K), Descemet’s membrane (D) and endothelial layer (En). (C) Control cornea of the aged group showing irregular eroded surface (s), thin epithelial layer with flat epithelial cells and degenerated nuclei (n) specially in the deep regions and absent nuclei in the superficial ones (a), thickened stroma with irregular collagenous fibers (c) and wide spaces in between (Sp), areas of edema (e), and areas of degenerated endothelial cells (l). (D) Treated cornea of the aged group showing regular surface (s), restoration of most of the epithelial cell nuclei (n), stroma with regular collagenous fibers (c) and relatively narrow spaces in-between (Sp), markedly thickened Descemet’s membrane (D) and a continuous layer of endothelium (En) (H&E, ×400; scale bar=50 μm).
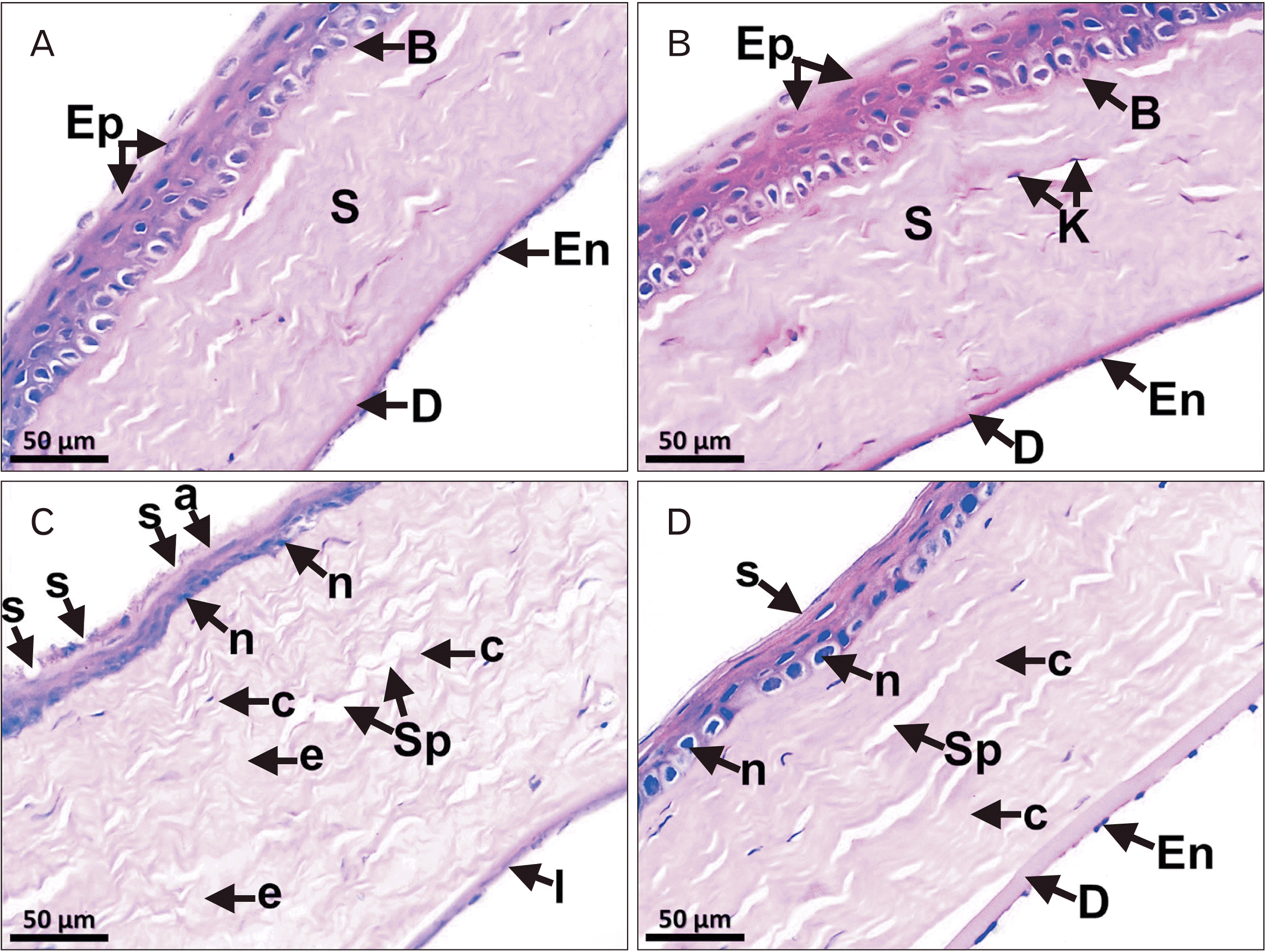
Fig. 4
Photomicrographs of sections of corneas of different study groups. (A) Control corneas of the adult group showing well distinct basal lamina of the epithelium (arrowheads) in most of areas and normally appearing Descemet’s membrane (arrows). (B) Treated corneas of the adult group showing well distinct basal lamina of the epithelium (arrowheads) in most of areas and slightly thickened Descemet’s membrane (arrows). (C) Control cornea of the aged group showing disappeared basal lamina of the epithelium and normal thickness of Descemet’s membrane (arrows). (D) Treated cornea of the aged group showing apparent basal lamina of the epithelium (arrowheads) and markedly thickened Descemet’s membrane (arrows) (periodic acid–Schiff stain, ×400; Scale bar=50 μm).
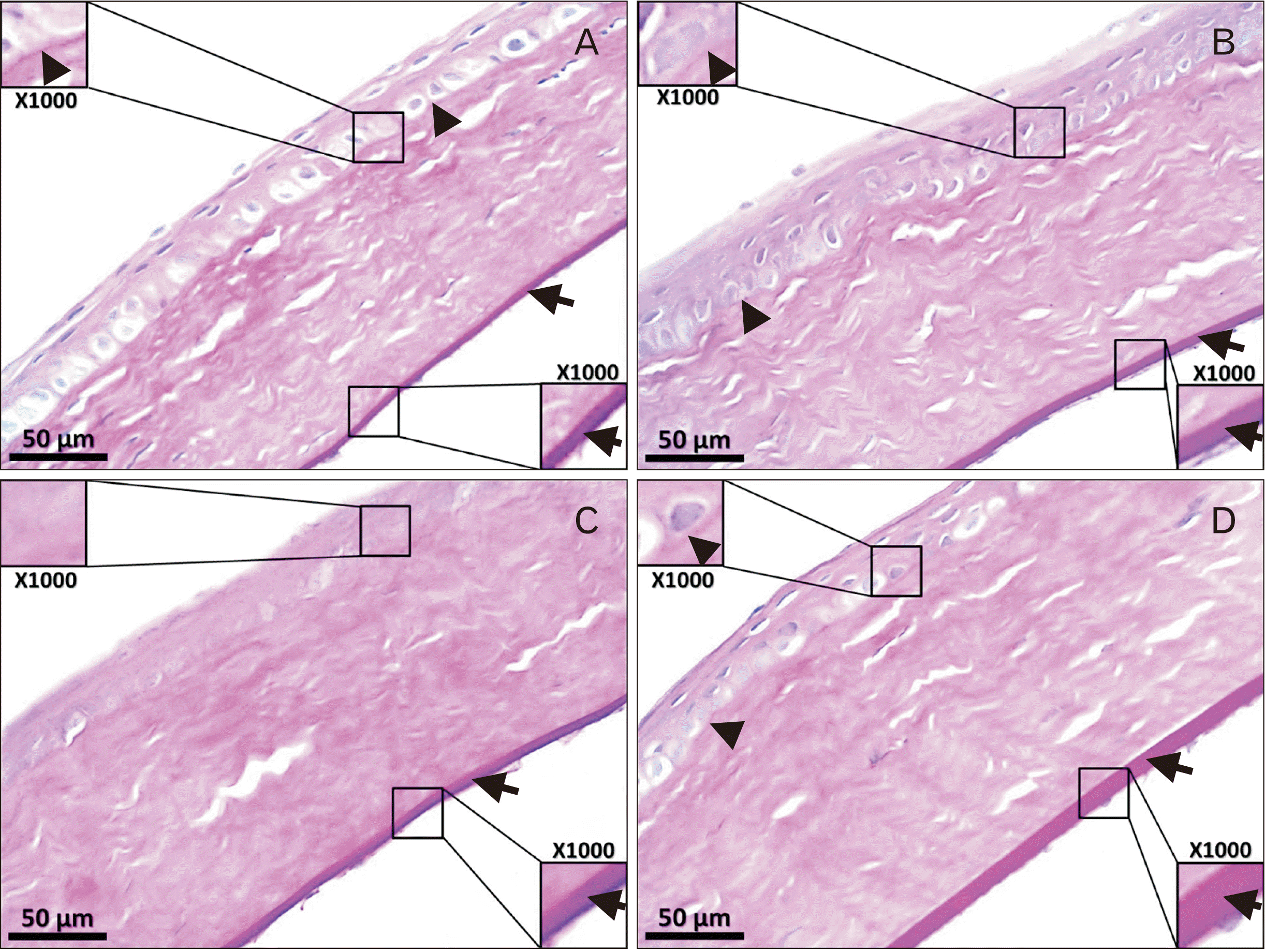
Fig. 5
Photomicrographs of epidermal growth factor receptor (EGFR) immuno-stained sections of cornea of study groups. (A) Control cornea of the adult group showing an endothelial (arrow) and basal epithelial (black arrowhead) extensive cytoplasmic immune reactions. The superficial layers of the epithelium show a minimal cytoplasmic expression (white arrowhead). (B) Treated cornea of the adult group showing moderate cytoplasmic immune reaction in the superficial epithelial layers (white arrowhead) with a membranous minimal basal epithelial (black arrowhead) and cytoplasmic endothelial (arrow) immune reactions. (C) Control cornea of the aged group showing a negative immune reaction in its all layers. (D) Treated cornea of the aged group showing an extensive cytoplasmic endothelial (arrow) and superficial epithelial (white arrowhead) immune reactions, and a minimal cytoplasmic basal epithelial reaction (black arrowhead) (EGFR immuno-stain, ×400; scale bar=50 μm).
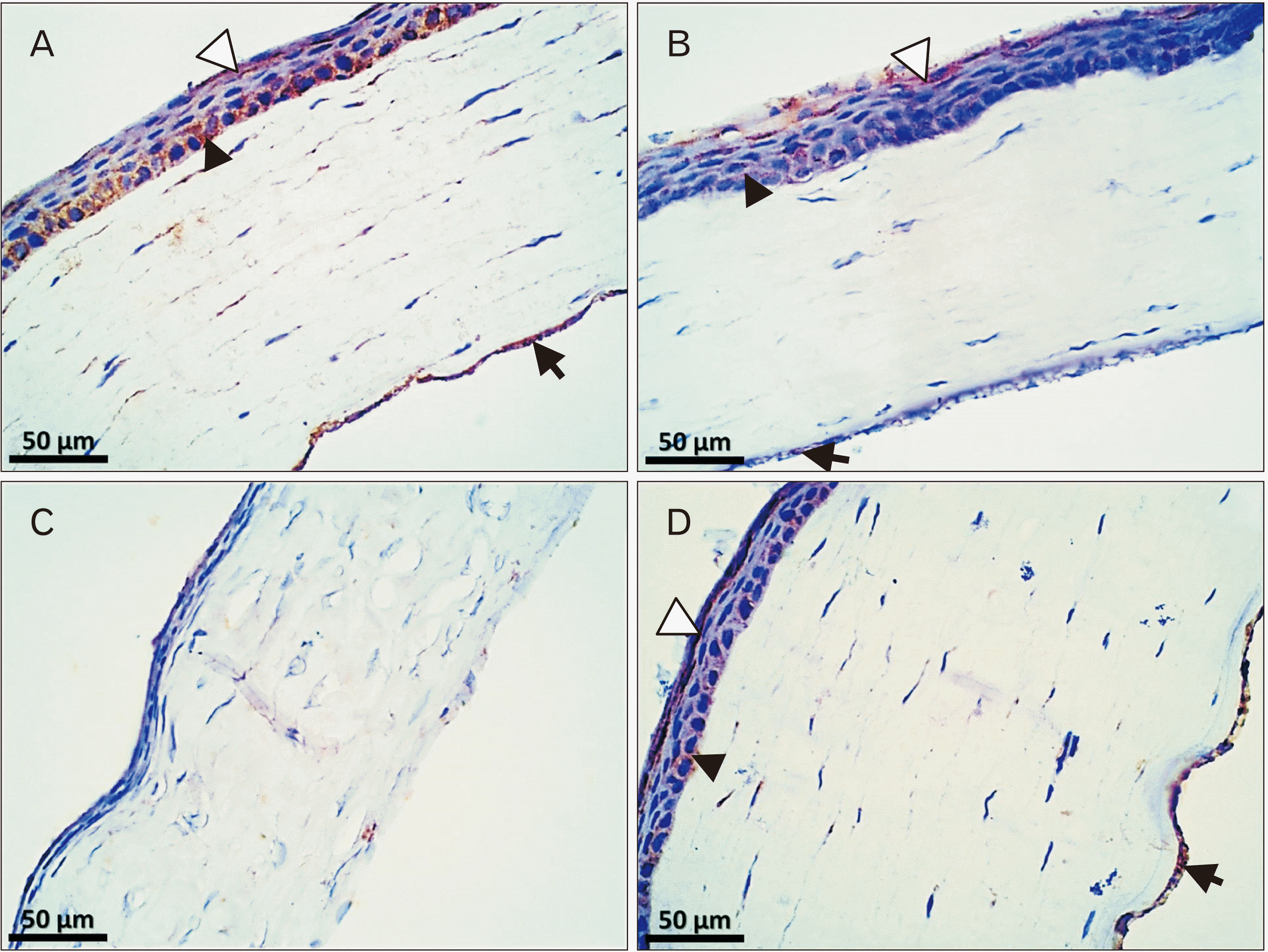
Fig. 6
Photomicrographs of paired box protein-6 (PAX6) immuno-stained sections of cornea of study groups. (A) Control cornea of the adult group showing minimal cytoplasmic basal epithelial (arrowhead) and endothelial (arrow) immune reactions. (B) Treated cornea of the adult group showing a negative immune reaction. (C) Control cornea of the aged group showing a negative immune reaction in all layers. (D) Treated cornea of the aged group showing a minimal cytoplasmic superficial epithelial (arrowhead) and endothelial (arrow) immune reactions (PAX6 immuno-stain, ×400; scale bar=50 μm).
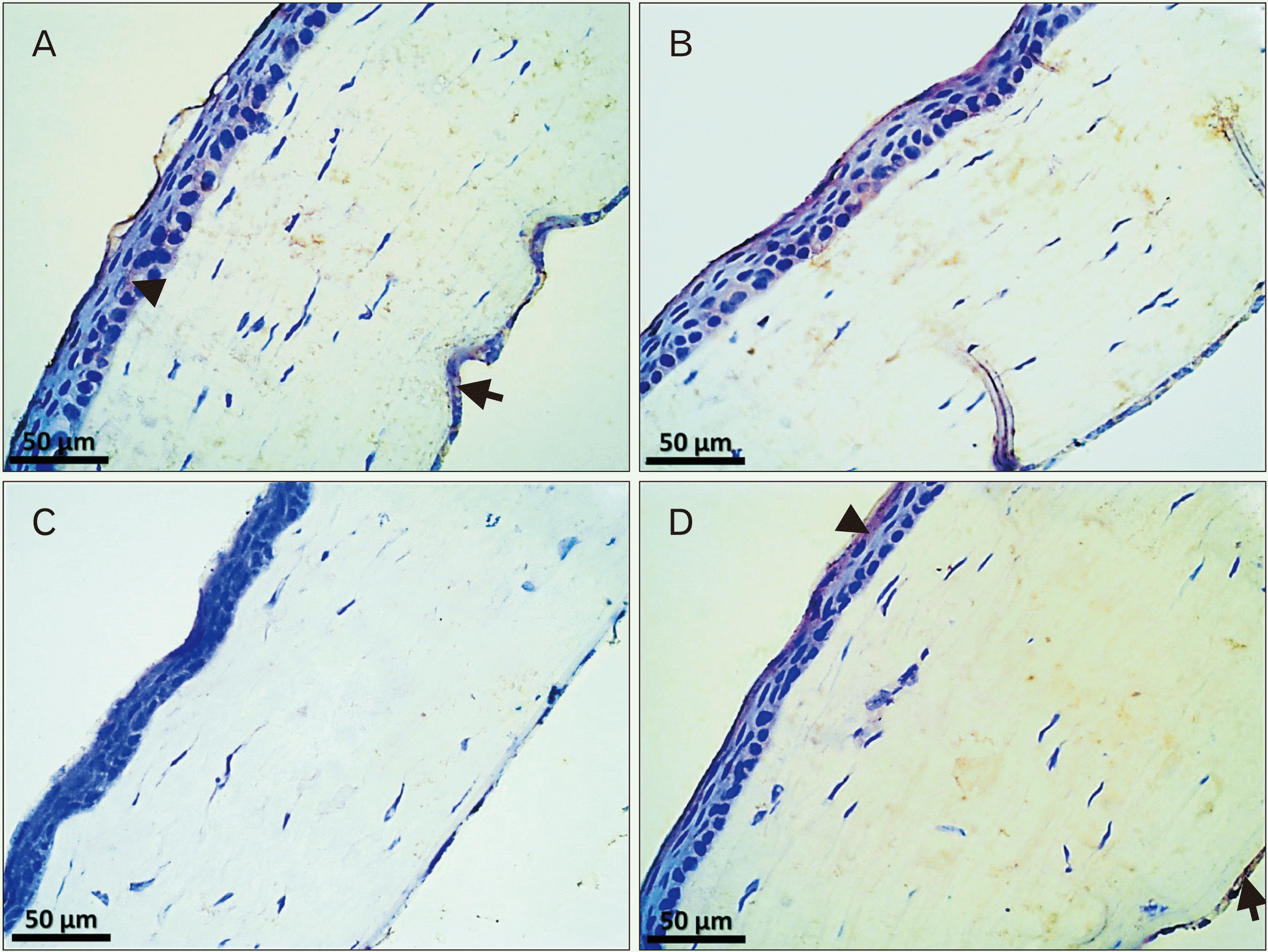
Table 1
Thicknesses of different layers of the cornea of study groups
Table 2
Cell densities of different layers of the cornea of study groups