Abstract
Oxaliplatin, a third-generation platinum derivative, is the mainstay of current antineoplastic medications for advanced colorectal cancer therapy. However, peripheral neuropathic complications, especially cold allodynia, undermine the life-prolonging outcome of this anti-cancer agent. Rosavin, a phenylpropanoid derived originally from Rhodiola rosea, exhibits a wide range of therapeutic properties. The present study explored whether and how rosavin alleviates oxaliplatin-induced cold hypersensitivity in mice. In the acetone drop test, cold allodynia behavior was observed from days 3 to 5 after a single injection of oxaliplatin (6 mg/kg, i.p.). Cold allodynia was significantly attenuated following rosavin treatment (10 mg/kg, i.p.). Specific endogenous 5-HT depletion by three consecutive pretreatments with para-chlorophenylalanine (150 mg/kg/day, i.p.) abolished the analgesic action of rosavin; this effect was not observed following pretreatment with naloxone (opioid receptor antagonist, 10 mg/kg, i.p.). Furthermore, 5-HT1A receptor antagonist WAY-100635 (0.16 mg/kg, i.p.), but not 5-HT3 receptor antagonist MDL-72222 (1 mg/kg, i.p.), blocked rosavin-induced analgesia. These results suggest that rosavin may provide a novel approach to alleviate oxaliplatin-induced cold allodynia by recruiting the activity of 5-HT1A receptors.
Oxaliplatin, a principal third-generation platinum derivative, is a core compound constituting diverse life-saving chemotherapeutic regimens (e.g., FOLFOX), commonly used to control the proliferation of advanced colorectal cancer [1]. Nonetheless, progressive neurotoxic comorbidities occur even after a single oxaliplatin infusion [2]. These acute sensory impairments include evoked pain in the extremities, with predominant hypersensitivity to cool touch or low temperature, termed cold allodynia [3]. Most cancer survivors with painful neuropathy report aggravation of sleep disorders, paresthesia, or dysesthesia, ultimately resulting in the reduction or cessation of chemotherapy [4]. Unfortunately, there is currently no validated prophylactic or curative analgesic strategy available.
In East Asia, Cinnamomi Cortex (C. Cortex), a cocktail of bioactive ingredients (e.g., coumarin, cinnamaldehyde, and cinnamic acid), has been widely utilized owing to its reliable benefits against influenza, diarrhea, and lack of energy, among others [5,6]. In our previous studies, the water extract of C. Cortex (WECC) and its major constituents, coumarin and cinnamic acid, were shown to possess analgesic properties in oxaliplatin-induced neuropathy, especially against cold allodynia [7,8]. In a subsequent preliminary study, we evaluated the possible analgesic actions of minor components derived from WECC and observed that rosavin demonstrated an analgesic effect. Rosavin, a phenylpropanoid derivative, is one of the main phytochemical ingredients of Rhodiola rosea (a traditional medicinal plant widespread in the Northern Hemisphere) [9], and its adaptogenic, antifatigue, and anti-inflammatory activities have been previously illustrated [10-12]. Moreover, it can substantially increase serotonin (5-HT) levels in the central nervous system (CNS) and improve endogenous opioid biosynthesis in certain models [13-16]. Notably, activation of serotonergic or opioid systems satisfies a core contribution for endogenous pain modulation [17,18]. However, the curative effect of rosavin on oxaliplatin-induced neuropathic complications remains elusive.
Here, we first elucidated whether three different doses of rosavin could alleviate oxaliplatin-induced cold hypersensitivity in mice. Second, we aimed to investigate the potential serotonergic or opioid analgesic mechanism of rosavin therapy; accordingly, appropriate experiments were performed after 5-HT depletion or opioid receptor blockade. Finally, we clarified the 5-HT receptor subtype that plays a vital role in rosavin-induced analgesia by systemically administering serotonergic receptor antagonists.
The dried bark of Cinnamomum cassia (6.0 kg) was extracted with 60 L of hot water twice at 100°C in a water bath for 2 h to obtain hot water extract (WECC). Then WECC (150.0 g) was suspended in water (1.0 L) and successively extracted with EtOAc (3 × 1 L), to obtain EtOAc-soluble (20.8 g) and water-soluble extracts (128.3 g), respectively. The water-soluble extract (128.3 g) was fractionated using column chromatography over Diaion HP-20 and eluted with an acetone/H2O gradient (from 0/1 to 1/0, v/v) to afford 11 fractions (W1−W11). Rosavin (66.5 mg) was purified from subfraction W10 using a flash chromatography system with a Redi Sep-C18 cartridge.
Male C57BL/6 mice, aged six weeks postnatal were provided by the Daehan Biolink (Emsung, Korea). The animals were housed in a controlled vivarium on an artificial 12-h light-dark cycle (light cycle: 7 AM to 7 PM) with ad libitum access to chow and water (n = 5/cage). All experimental protocols were approved by the Kyung Hee University Animal Care and Use Committee (KHUASP (SE) 20-147; approved April 2020) and conducted in accordance with the ethical guidelines of the International Association for the Study of Pain [19].
One week before performing the measurements, mice were individually placed beneath an inverted, transparent plastic cage (12 × 8 × 6 cm), atop a steel mesh floor, and acclimated for 30 min by the same experimenter [20]. The acetone drop test was performed to examine cold allodynia, as previously described [21,22]. In brief, 10 μl of acetone (a usually innocuous stimulus; Daejung Chemicals & Metals Co. Ltd., Siheung, Korea) was applied to the plantar skin of the right hind paw three times at 5 min intervals. The frequencies of brisk reactions (e.g., withdrawal, licking, and shaking) were quantified for 30 s.
Chemotherapy-induced peripheral neuropathy (CIPN) was established by a single intraperitoneal infusion of oxaliplatin (6 mg/kg, 2 mg/ml; Sigma, St. Louis, MO, USA) [20]. An equivalent volume of 5% glucose solution (Sigma) was administered to control animals. Rosavin was dissolved in 0.08% Tween 80 solution (Sigma), and intraperitoneally administered to mice, accompanied by neuropathic pain-like behavior, at doses of 1, 5, or 10 mg/kg. Neuropharmacological analyses were performed to elucidate whether the analgesic mechanism of rosavin was mediated via the opioid or serotonergic systems; accordingly, pretreatment with the opioid receptor antagonist naloxone (10 mg/kg, i.p.; Sigma) or 5-HT neurotoxin para-chlorophenylalanine (PCPA, 150 mg/kg/day, i.p.; Sigma) was undertaken [20,23]. Pretreatment with WAY-100635 (5-HT1A receptor antagonist, 0.16 mg/kg, i.p.; Sigma) or bemesetron (MDL-72222, a 5-HT3 receptor antagonist, 1 mg/kg, i.p.; Tocris, Cookson, UK) was performed to determine potential 5-HT receptor subtypes that mediate the analgesic action of rosavin [16,20]. Pretreatment with PCPA was performed prior to the oxaliplatin injection for three consecutive days [20]. Respective antagonists were administered 15 min before rosavin administration [16].
Statistical analysis was performed using GraphPad Prism v7.0 (GraphPad Software, La Jolla, CA, USA), and differences were considered significant at p < 0.05. Data are presented as mean ± standard error of the mean (SEM). Two-way analysis of variance (ANOVA) followed by Bonferroni’s multiple comparison test was used for all figures.
A single intraperitoneal oxaliplatin infusion elicited cold allodynia in mice over 8 days of evaluations. On day 0, no differences were observed in baseline sensitivity between groups. Consistent with previous studies [21,24], the licking and shaking frequencies of the hind paw in response to a topical evaporative cold stimulus (10 μl of acetone) were increased in oxaliplatin-administered mice when compared with controls, from days 3 to 5 (p < 0.05, day 3; p < 0.01, day 5, Fig. 1). These aberrant increments in withdrawals generated by innocuous cold stimuli are characteristic of oxaliplatin-induced cold complications in cancer survivors.
Next, to explore the potential suppressive effect of rosavin on oxaliplatin-induced cold allodynia, 31 mice were randomly allocated into four groups when behavioral hypersensitivity was significant (days 3–5, Fig. 1), receiving either rosavin (1, 5, and 10 mg/kg) or vehicle. The acetone drop test was conducted before injection and reassessed at 30, 60, and 120 min after treatment. Among the treatment regimens employed, the highest rosavin dose (10 mg/kg) significantly ameliorated the worsening of cold complications when compared with the control group, lasting for 60 min (p < 0.05, 30 and 60 min, Fig. 2). However, reductions in allodynic responses induced by the lowest or the intermediate doses were apparent only at the 30 min time point (p < 0.05). In addition, using the von Frey hair test [20,22], we found rosavin-induced analgesia against mechanical allodynia (see Supplementary Fig. 1). Our findings revealed that rosavin exhibited more pronounced effects against cold allodynia than mechanical allodynia. Accordingly, we further investigated the specific analgesic mechanism of rosavin using the acetone drop test.
We attempted to elucidate the potential opioid or serotonergic analgesic mechanism of rosavin. Accordingly, mice were pretreated with naloxone (opioid receptor antagonist, 10 mg/kg, i.p.) prior to rosavin administration or administered with PCPA (5-HT neurotoxin, 150 mg/kg/day, i.p.) before chemotherapy for three consecutive days. Cold sensitivity was assessed before rosavin (10 mg/kg, i.p.) administration and reassessed 30 min after its treatment (see Supplementary Fig. 2). As the vehicle employed for naloxone/PCPA (saline) showed a limited effect on the rosavin-induced analgesia, we merged data from the two groups as controls. Rosavin significantly attenuated the allodynic behavior in the control and naloxone groups (Vehicle + Rosavin, p < 0.001, and Naloxone + Rosavin, p < 0.001, vs. Before, Fig. 3). However, in the 5-HT depleted group, rosavin failed to ameliorate aberrant behavior (PCPA + Rosavin, p > 0.05, vs. Before). Overall, the endogenous 5-HT system, but not the opioid pathway, crucially mediated rosavin-induced analgesia against oxaliplatin-induced cold allodynia.
Finally, to further determine which 5-HT receptor subtypes mediate the ameliorative properties of rosavin, mice were intraperitoneally administered 5-HT1A receptor antagonist WAY-100635 (0.16 mg/kg), 5-HT3 receptor antagonist MDL-72222 (1 mg/kg), or the respective vehicle (0.2 ml of saline or 20% DMSO, respectively) 15 min prior to rosavin treatment (10 mg/kg). We evaluated cold allodynia twice before antagonist administration and 30 min after rosavin treatment. As vehicles employed for antagonist preparation had a limited influence on rosavin-induced analgesia, we pooled data from the two groups as controls. Pretreatment with WAY-100635, but not MDL-72222 or appropriate vehicles, abolished rosavin-induced analgesia (WAY-100635 + Rosavin, p > 0.05, MDL-72222 + Rosavin, p < 0.01, and Vehicle + Rosavin, p < 0.001, vs. Before, Fig. 4). Our findings demonstrated the specific and vital involvement of the 5-HT1A receptor-mediated pathway in the suppressive properties of rosavin against oxaliplatin-induced cold allodynia.
Despite extensive investigations and remarkable achievements in recent years, oxaliplatin-induced dose-limiting impediments such as cold-dominant neuropathic pain remain a challenge for cancer survivors beyond chemotherapy completion [2]. In oriental medicine, natural plants and animals afford potential sources for the design of emerging analgesics, which may possess more promising analgesic features than conventional painkillers in certain pain-inducing disorders [25]. Recently, we investigated the substantial analgesic effects of phytocompounds present in C. Cortex (i.e., coumarin and cinnamic acid) in CIPN rodent models [7,8]. Additionally, rosavin, another constituent derived from WECC, was shown to markedly alter the concentrations of endogenous 5-HT and enkephalins [14,15]. This evidence suggests that rosavin may also possess analgesic effects against CIPN. In the present study, we assessed rosavin-induced analgesia and elucidated its underlying mechanisms against oxaliplatin-induced cold allodynia in mice.
Our chemotherapeutic regimen successfully mimicked the cold allodynia observed in cancer survivors, with a single intraperitoneal injection of oxaliplatin (6 mg/kg) increasing withdrawal behavior in the acetone test (Fig. 1). Rosavin therapy has reported analgesic benefits in different pre-clinical models (e.g., osteoarthritis, inflammatory, or diabetic pain model) [16,26,27]. However, our study is the first to introduce its possible indication in CIPN. Among the treatment doses employed (1, 5, and 10 mg/kg), the highest dose exhibited the most prolonged relief in terms of cold hypersensitivity (60 min vs. 30 min, Fig. 2). Previously, we revealed that cinnamic acid exhibits the most efficacious action at a dose of 20 mg/kg, markedly mitigating both cold and mechanical hypersensitivity induced by oxaliplatin [7]; however rosavin therapy yielded limited beneficial effects on mechanical allodynia (Supplementary Fig. 1), and coumarin had no effect on mechanical allodynia [8]. This discrepancy could imply that distinct underlying mechanisms are involved in CIPN regulation mediated via each phytocompound of C. Cortex.
Few studies have elucidated rosavin-induced effect on cold hypersensitivity, with little known regarding its precise analgesic mechanism. In this context, we observed that intraperitoneal pre-administration of PCPA, but not naloxone, markedly abolished rosavin analgesia (Fig. 3), indicating that the endogenous serotonergic mechanism plays a key role in mediating its effect. Furthermore, antagonism of 5-HT1A, but not 5-HT3 receptors blocked the anti-allodynic effect of rosavin (Fig. 4). Data from previous publications could explain our findings. In the formalin test, the antinociceptive effect of Rhodiola rosea extract, containing 2.7% rosavin, could be significantly counteracted by the subcutaneous pretreatment with WAY100635 (0.16 mg/kg), but not naltrexone (1 mg/kg, another opioid receptor antagonist) [16]. Rhodiola rosea extract effectively reverted 5-HT levels in the rat hippocampus under conditions of chronic mild stress [14]. Stancheva and Mosharrof demonstrated that Rhodiola rosea modulates biogenic monoamines at the CNS, where dopamine and norepinephrine decreased in the cerebral cortex as well as in the brainstem, but a considerable increase in 5-HT levels was observed [13]. Thus, it is likely that the serotonergic, but not the dopaminergic or noradrenergic, mechanisms are involved in the effects of rosavin. Moreover, in streptozotocin-induced diabetic rats, subcutaneous injection of Rhodiola rosea extract at the dorsal surface of hind paw decreased the rate of formalin-induced flinches, at a dose half that of the anticonvulsant medication, gabapentin, which achieved almost identical analgesia (10 mg/paw vs. 20 mg/paw) [27]. Herein, we found the involvement of 5-HT1A receptors in analgesic effect of rosavin. The 5-HT1A receptor is enriched in a variety of brain regions, such as the limbic areas and hippocampus [28]. Also, this receptor is one of the most prevalent and most plastic serotonergic receptors in the spinal cord, with the highest density in laminae III–VI [29]. Activations of this receptor could suppress nociception in diverse rodent models [30,31]. More importantly, the growth inhibitory effects of rosavin on bladder cancer, Ehrlich's tumor, and Pliss' lymphosarcoma have been previously reported [32,33]. Collectively, these results suggest that adjunct rosavin therapy could be employed as an ideal strategy to alleviate oxaliplatin-induced neuropathy without diminishing chemotherapy efficacy, further providing better life-saving benefits in clinical settings.
Supplementary data including two figures can be found with this article online at https://doi.org/10.4196/kjpp.2021.25.5.489.
ACKNOWLEDGEMENTS
This work was supported by the National Research Foundation of Korea (NRF) grant funded by the Korean government (NRF-2017M3A9E4057926).
Notes
REFERENCES
1. Zajączkowska R, Kocot-Kępska M, Leppert W, Wrzosek A, Mika J, Wordliczek J. 2019; Mechanisms of chemotherapy-induced peripheral neuropathy. Int J Mol Sci. 20:1451. DOI: 10.3390/ijms20061451. PMID: 30909387. PMCID: PMC6471666.


2. Boyette-Davis JA, Hou S, Abdi S, Dougherty PM. 2018; An updated understanding of the mechanisms involved in chemotherapy-induced neuropathy. Pain Manag. 8:363–375. DOI: 10.2217/pmt-2018-0020. PMID: 30212277. PMCID: PMC6462837.


3. Starobova H, Vetter I. 2017; Pathophysiology of chemotherapy-induced peripheral neuropathy. Front Mol Neurosci. 10:174. DOI: 10.3389/fnmol.2017.00174. PMID: 28620280. PMCID: PMC5450696.


4. Kerckhove N, Collin A, Condé S, Chaleteix C, Pezet D, Balayssac D. 2017; Long-term effects, pathophysiological mechanisms, and risk factors of chemotherapy-induced peripheral neuropathies: a comprehensive literature review. Front Pharmacol. 8:86. DOI: 10.3389/fphar.2017.00086. PMID: 28286483. PMCID: PMC5323411.


5. Choi HM, Jung Y, Park J, Kim HL, Youn DH, Kang J, Jeong MY, Lee JH, Yang WM, Lee SG, Ahn KS, Um JY. 2016; Cinnamomi cortex (Cinnamomum verum) suppresses testosterone-induced benign prostatic hyperplasia by regulating 5α-reductase. Sci Rep. 6:31906. DOI: 10.1038/srep31906. PMID: 27549514. PMCID: PMC4994048.


6. Hayashi K, Imanishi N, Kashiwayama Y, Kawano A, Terasawa K, Shimada Y, Ochiai H. 2007; Inhibitory effect of cinnamaldehyde, derived from Cinnamomi cortex, on the growth of influenza A/PR/8 virus in vitro and in vivo. Antiviral Res. 74:1–8. DOI: 10.1016/j.antiviral.2007.01.003. PMID: 17303260.


7. Chae HK, Kim W, Kim SK. 2019; Phytochemicals of Cinnamomi Cortex: cinnamic acid, but not cinnamaldehyde, attenuates oxaliplatin-induced cold and mechanical hypersensitivity in rats. Nutrients. 11:432. DOI: 10.3390/nu11020432. PMID: 30791474. PMCID: PMC6412559.


8. Kim C, Lee JH, Kim W, Li D, Kim Y, Lee K, Kim SK. 2016; The suppressive effects of Cinnamomi Cortex and its phytocompound coumarin on oxaliplatin-induced neuropathic cold allodynia in rats. Molecules. 21:1253. DOI: 10.3390/molecules21091253. PMID: 27657030. PMCID: PMC6274362.


9. Perfumi M, Mattioli L. 2007; Adaptogenic and central nervous system effects of single doses of 3% rosavin and 1% salidroside Rhodiola rosea L. extract in mice. Phytother Res. 21:37–43. DOI: 10.1002/ptr.2013. PMID: 17072830.
10. Chiang HM, Chen HC, Wu CS, Wu PY, Wen KC. 2015; Rhodiola plants: chemistry and biological activity. J Food Drug Anal. 23:359–369. DOI: 10.1016/j.jfda.2015.04.007. PMID: 28911692.


11. Park JS, Choi J, Kwon JY, Jung KA, Yang CW, Park SH, Cho ML. 2018; A probiotic complex, rosavin, zinc, and prebiotics ameliorate intestinal inflammation in an acute colitis mouse model. J Transl Med. 16:37. DOI: 10.1186/s12967-018-1410-1. PMID: 29466999. PMCID: PMC5822606.


12. Panossian AG, Efferth T, Shikov AN, Pozharitskaya ON, Kuchta K, Mukherjee PK, Banerjee S, Heinrich M, Wu W, Guo DA, Wagner H. 2021; Evolution of the adaptogenic concept from traditional use to medical systems: pharmacology of stress- and aging-related diseases. Med Res Rev. 41:630–703. DOI: 10.1002/med.21743. PMID: 33103257. PMCID: PMC7756641.


13. Stancheva SL, Mosharrof A. 1987; Effect of the extract of Rhodiola rosea L. on the content of the brain biogenic monamines. Med Physiol. 40:85–87.
14. Chen QG, Zeng YS, Qu ZQ, Tang JY, Qin YJ, Chung P, Wong R, Hägg U. 2009; The effects of Rhodiola rosea extract on 5-HT level, cell proliferation and quantity of neurons at cerebral hippocampus of depressive rats. Phytomedicine. 16:830–838. DOI: 10.1016/j.phymed.2009.03.011. PMID: 19403286.


15. Lishmanov IuB, Trifonova ZhV, Tsibin AN, Maslova LV, Dement'eva LA. 1987; Plasma beta-endorphin and stress hormones in stress and adaptation. Biull Eksp Biol Med. 103:422–424. Russian. DOI: 10.1007/BF00842472. PMID: 2952180.
16. Montiel-Ruiz RM, González-Trujano ME, Déciga-Campos M. 2013; Synergistic interactions between the antinociceptive effect of Rhodiola rosea extract and B vitamins in the mouse formalin test. Phytomedicine. 20:1280–1287. DOI: 10.1016/j.phymed.2013.07.006. PMID: 23920277.


17. Millan MJ. 2002; Descending control of pain. Prog Neurobiol. 66:355–474. DOI: 10.1016/S0301-0082(02)00009-6. PMID: 12034378.


18. Nguyen LM, Rhondali W, De la Cruz M, Hui D, Palmer L, Kang DH, Parsons HA, Bruera E. 2013; Frequency and predictors of patient deviation from prescribed opioids and barriers to opioid pain management in patients with advanced cancer. J Pain Symptom Manage. 45:506–516. DOI: 10.1016/j.jpainsymman.2012.02.023. PMID: 22940562. PMCID: PMC3856203.


19. Zimmermann M. 1983; Ethical guidelines for investigations of experimental pain in conscious animals. Pain. 16:109–110. DOI: 10.1016/0304-3959(83)90201-4. PMID: 6877845.


20. Li D, Lee JH, Choi CW, Kim J, Kim SK, Kim W. 2019; The analgesic effect of venlafaxine and its mechanism on oxaliplatin-induced neuropathic pain in mice. Int J Mol Sci. 20:1652. DOI: 10.3390/ijms20071652. PMID: 30987090. PMCID: PMC6479607.


21. Li D, Lee Y, Kim W, Lee K, Bae H, Kim SK. 2015; Analgesic effects of Bee Venom derived phospholipase A2 in a mouse model of oxaliplatin-induced neuropathic pain. Toxins (Basel). 7:2422–2434. DOI: 10.3390/toxins7072422. PMID: 26131771. PMCID: PMC4516921.
22. Li D, Chung G, Kim SK. 2020; The involvement of central noradrenergic pathway in the analgesic effect of bee venom acupuncture on vincristine-induced peripheral neuropathy in rats. Toxins (Basel). 12:775. DOI: 10.3390/toxins12120775. PMID: 33291335. PMCID: PMC7762247.


23. Nakagawa T, Minami M, Katsumata S, Ienaga Y, Satoh M. 1995; Suppression of naloxone-precipitated withdrawal jumps in morphine-dependent mice by stimulation of prostaglandin EP3 receptor. Br J Pharmacol. 116:2661–2666. DOI: 10.1111/j.1476-5381.1995.tb17223.x. PMID: 8590986. PMCID: PMC1909143.
24. Choi S, Chae HK, Heo H, Hahm DH, Kim W, Kim SK. 2019; Analgesic effect of melittin on oxaliplatin-induced peripheral neuropathy in rats. Toxins (Basel). 11:396. DOI: 10.3390/toxins11070396. PMID: 31288453. PMCID: PMC6669583.


25. Sung SH, Kim JW, Han JE, Shin BC, Park JK, Lee G. 2021; Animal venom for medical usage in pharmacopuncture in Korean medicine: current status and clinical implication. Toxins (Basel). 13:105. DOI: 10.3390/toxins13020105. PMID: 33535603. PMCID: PMC7912904.


26. Kwon JY, Lee SH, Jhun J, Choi J, Jung K, Cho KH, Kim SJ, Yang CW, Park SH, Cho ML. 2018; The combination of probiotic complex, rosavin, and zinc improves pain and cartilage destruction in an osteoarthritis rat model. J Med Food. 21:364–371. DOI: 10.1089/jmf.2017.4034. PMID: 29346012.


27. Déciga-Campos M, González-Trujano ME, Ventura-Martínez R, Montiel-Ruiz RM, Ángeles-López GE, Brindis F. 2016; Antihyperalgesic activity of Rhodiola rosea in a diabetic rat model. Drug Dev Res. 77:29–36. DOI: 10.1002/ddr.21289. PMID: 26763184.
28. Polter AM, Li X. 2010; 5-HT1A receptor-regulated signal transduction pathways in brain. Cell Signal. 22:1406–1412. DOI: 10.1016/j.cellsig.2010.03.019. PMID: 20363322. PMCID: PMC2903656.


29. Otoshi CK, Walwyn WM, Tillakaratne NJ, Zhong H, Roy RR, Edgerton VR. 2009; Distribution and localization of 5-HT1A receptors in the rat lumbar spinal cord after transection and deafferentation. J Neurotrauma. 26:575–584. DOI: 10.1089/neu.2008.0640. PMID: 19260781. PMCID: PMC2828940.
30. Oyama T, Ueda M, Kuraishi Y, Akaike A, Satoh M. 1996; Dual effect of serotonin on formalin-induced nociception in the rat spinal cord. Neurosci Res. 25:129–135. DOI: 10.1016/0168-0102(96)01034-6. PMID: 8829149.


31. Lee JH, Min D, Lee D, Kim W. 2021; Zingiber officinale roscoe rhizomes attenuate oxaliplatin-induced neuropathic pain in mice. Molecules. 26:548. DOI: 10.3390/molecules26030548. PMID: 33494465. PMCID: PMC7866215.
32. Liu Z, Li X, Simoneau AR, Jafari M, Zi X. 2012; Rhodiola rosea extracts and salidroside decrease the growth of bladder cancer cell lines via inhibition of the mTOR pathway and induction of autophagy. Mol Carcinog. 51:257–267. DOI: 10.1002/mc.20780. PMID: 21520297. PMCID: PMC3144985.


33. Udintsev SN, Shakhov VP. 1989; Decrease in the growth rate of Ehrlich's tumor and Pliss' lymphosarcoma with partial hepatectomy. Vopr Onkol. 35:1072–1075. Russian.
Fig. 1
Changes in cold hypersensitivity over time in oxaliplatin and control groups.
Animals were randomly administered 5% glucose solution or oxaliplatin (vehicle or OXA, n = 8/group). Mice were subjected to the acetone drop test immediately before the administration on day 0 and from days 1 to 7, respectively (timeline: days 0, 1, 3, 5, and 7). The acetone-elicited responses were counted over 30 s post-applications. Data are expressed as mean ± SEM; *p < 0.05, **p < 0.01, vs. vehicle; by Bonferroni post-hoc test after two-way analysis of variance (ANOVA).
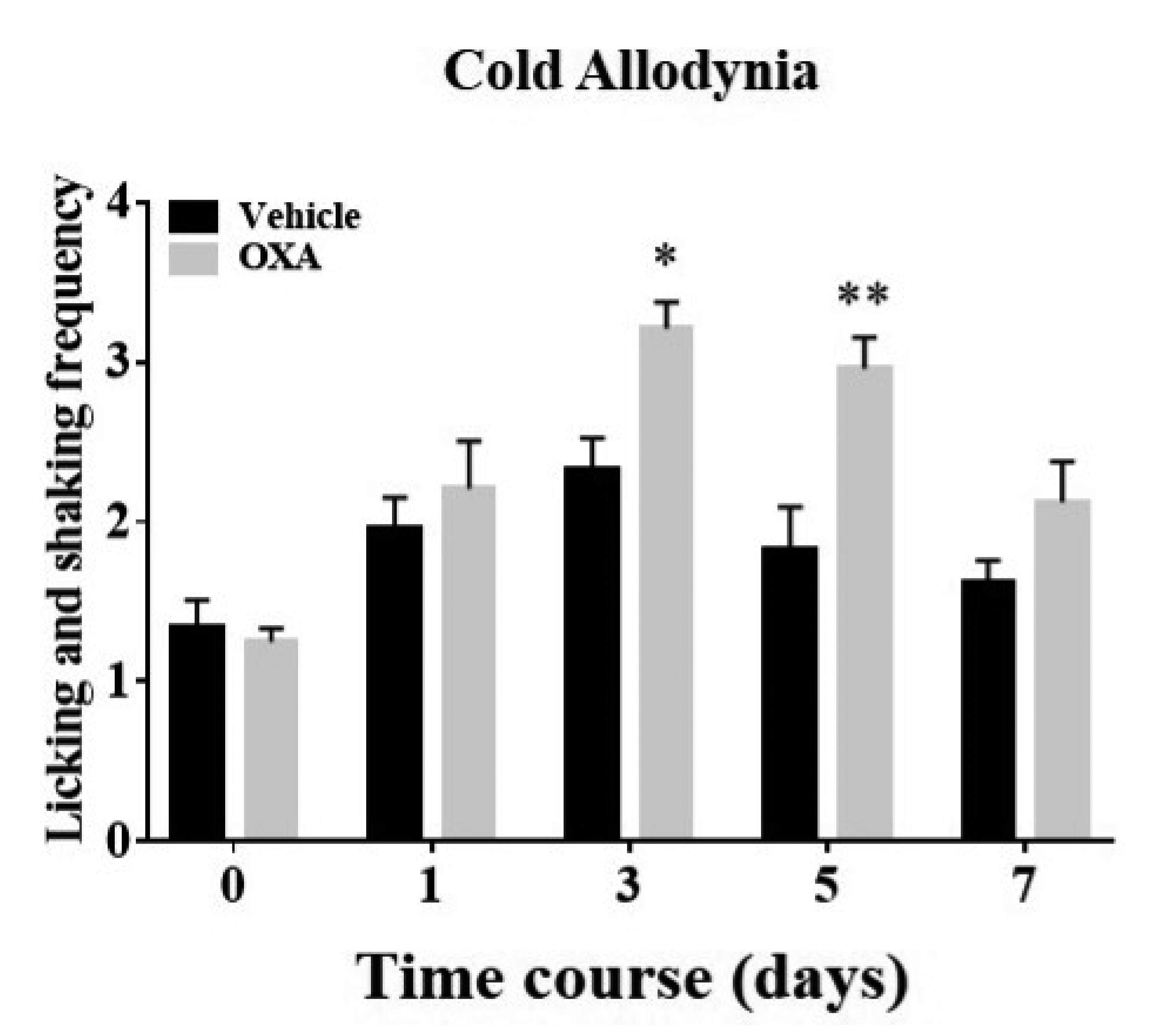
Fig. 2
Rosavin inhibits cold stimuli‐evoked behavioral hypersensitivity in the oxaliplatin group.
Rosavin (1 mg/kg, n = 7; 5 mg/kg, n = 7; 10 mg/kg, n = 8) or vehicle (control, n = 9) was administrated to randomly assigned animals. The acetone drop assay was performed four times, once before application and reassessments at 30, 60, and 120 min post-dosing, respectively (timeline: 0, 30, 60, and 120). Data are expressed as mean ± SEM; *p < 0.05, vs. vehicle; by Bonferroni post-hoc test after two-way analysis of variance (ANOVA).
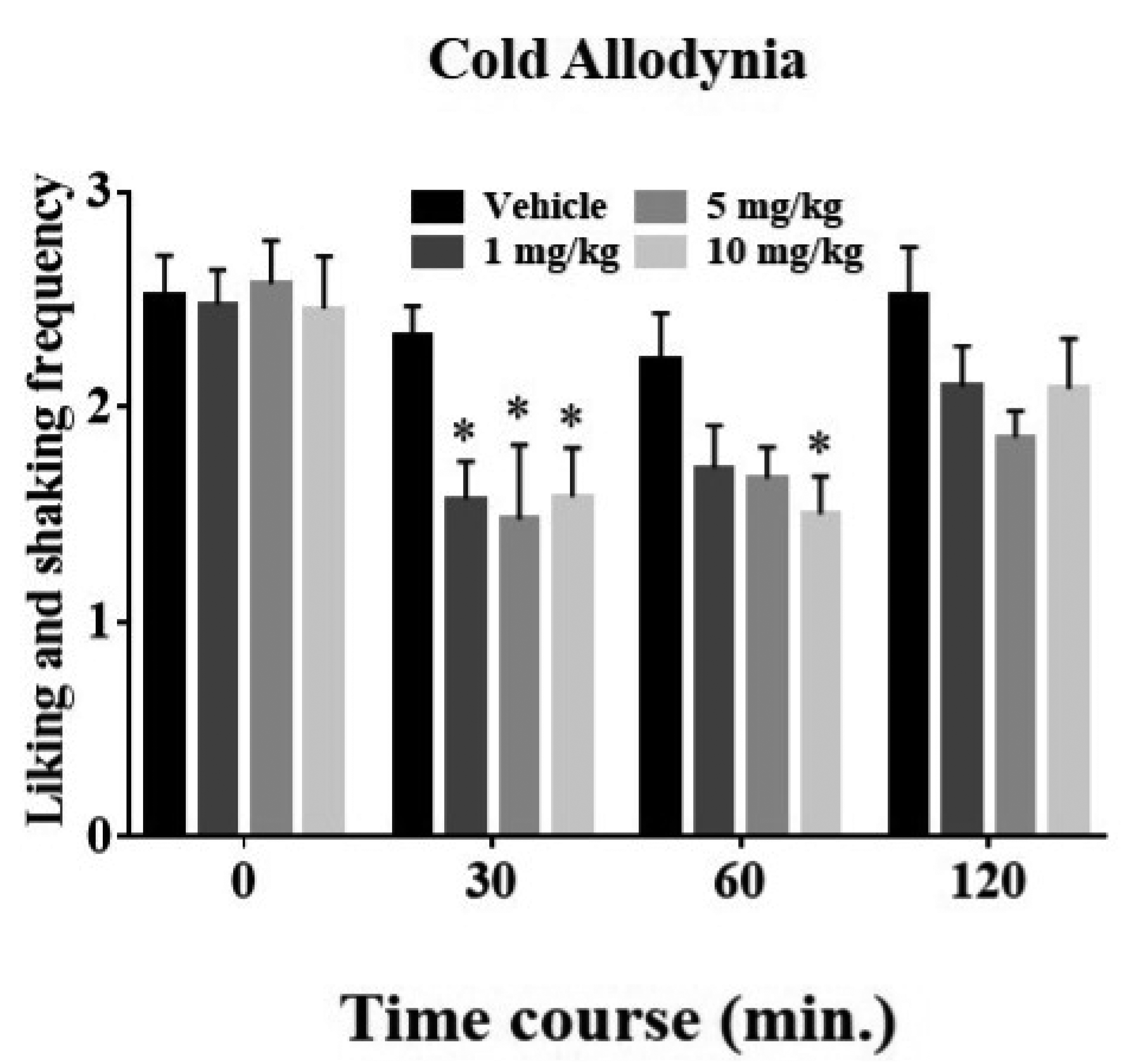
Fig. 3
Roles of the serotonergic system in mediating ameliorative effects of rosavin against oxaliplatin-induced cold allodynia in mice.
Pretreatment with para-chlorophenylalanine (PCPA) (n = 8) was performed prior to oxaliplatin injection for three consecutive days, and naloxone (n = 6) was administered 15 min before rosavin administration. An equivalent volume of saline was pre-administered to controls (n = 12). Mice presenting neuropathic pain-like behavior were subjected to the acetone drop assay before rosavin treatment (10 mg/kg, Before) and 30 min after administration (After). Data are expressed as mean ± SEM; ***p < 0.001, vs. Before; by Bonferroni post-hoc test after two-way analysis of variance (ANOVA).
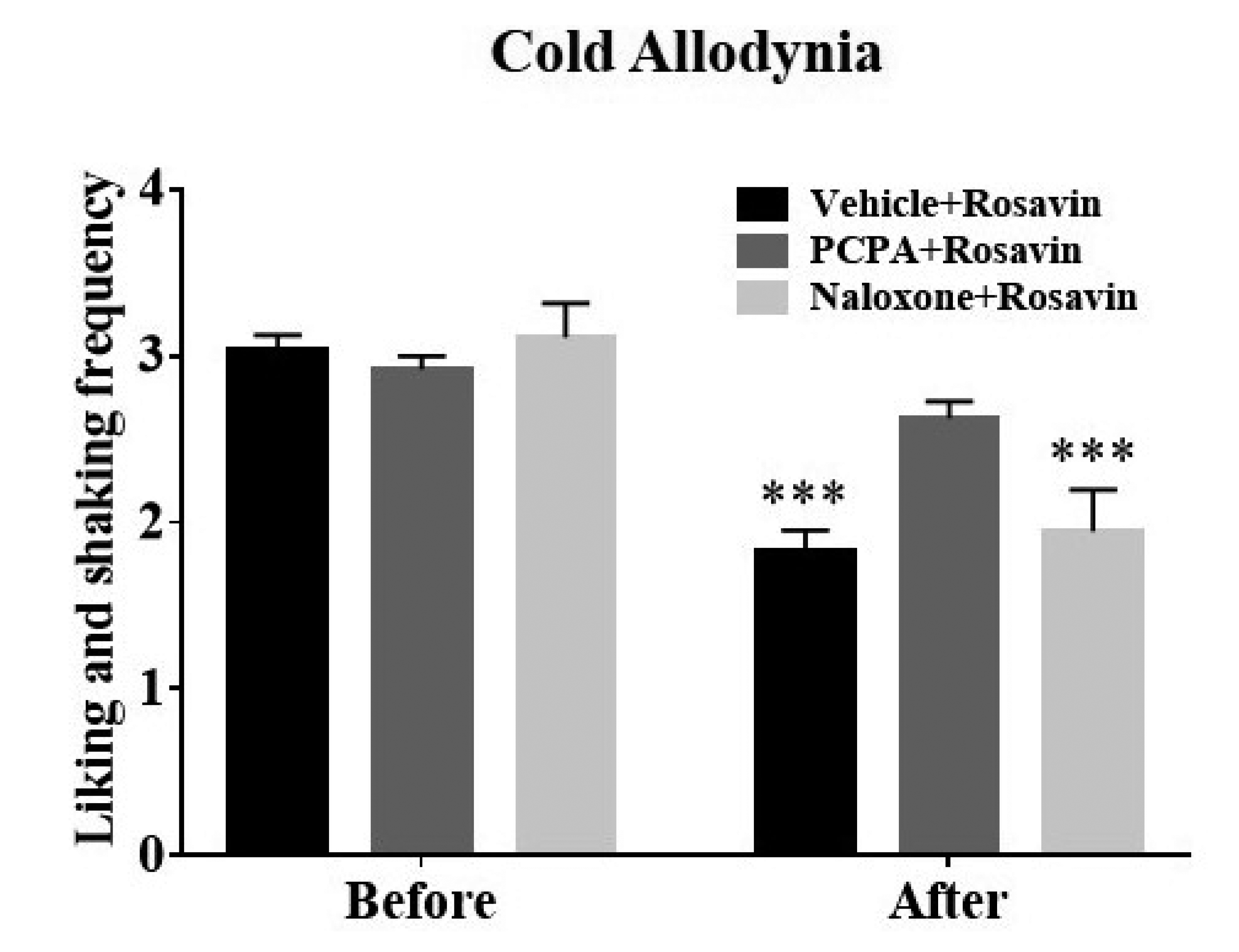
Fig. 4
Effects of 5-HT receptor antagonism on the suppressive properties of rosavin against oxaliplatin-induced cold allodynia in mice.
Mice with allodynia were randomly administered with WAY-100635 (n = 6), MDL-72222 (n = 6), or an equivalent volume of the vehicles (saline, the vehicle of WAY-100635, n = 5 or 20% DMSO, the vehicle of MDL-72222, n = 5) 15 min prior to rosavin treatment at days 3 to 5. Cold sensitivity was assessed before antagonist administration (Before) and 30 min after rosavin application (After). Data are expressed as mean ± SEM; **p < 0.01, ***p < 0.001, vs. Before; by Bonferroni post-hoc test after two-way analysis of variance (ANOVA).
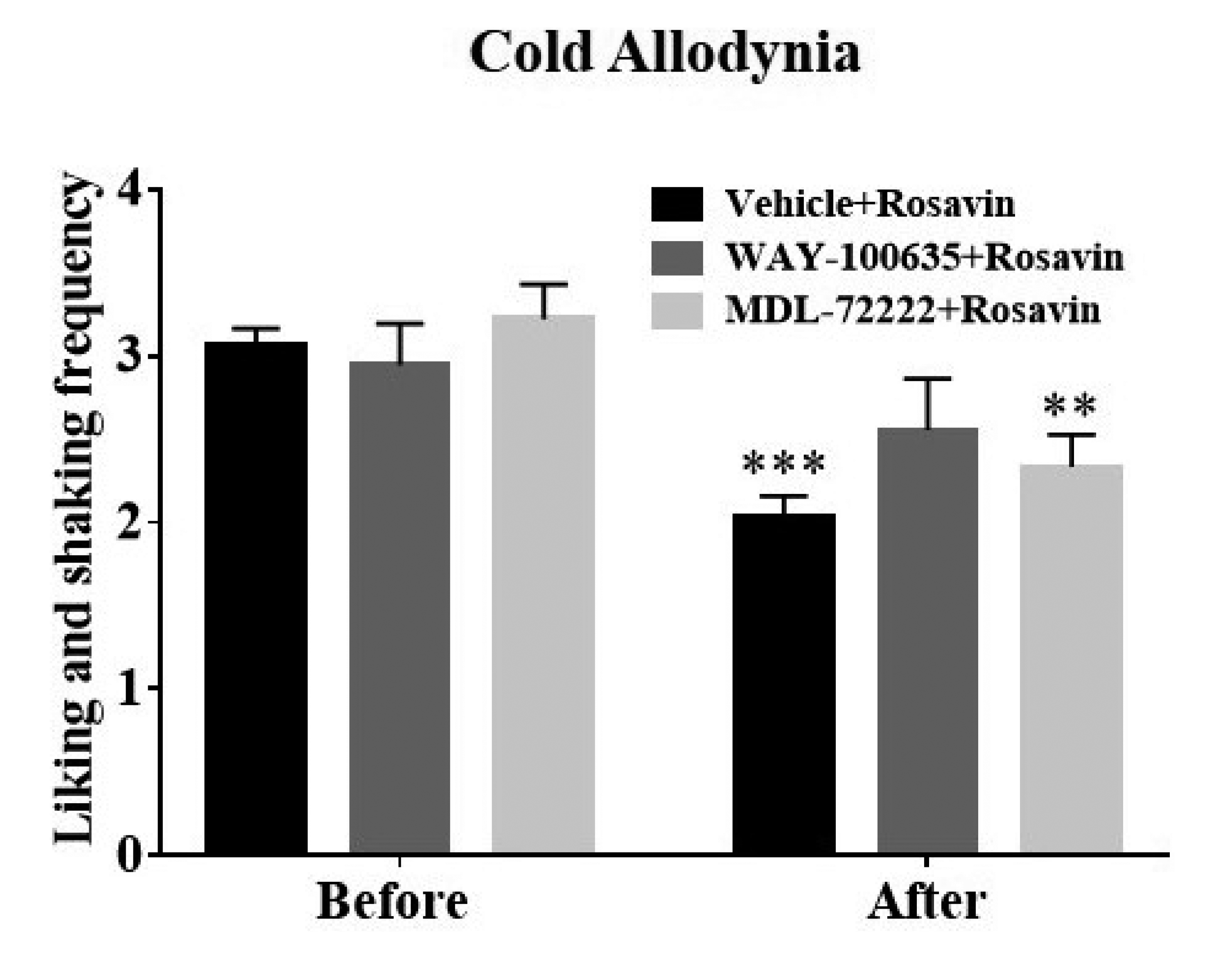