Abstract
Extended inflammation and cytokine production pathogenically contribute to a number of inflammatory disorders. Formosanin C (FC) is the major diosgenin saponin found in herb Paris formosana Hayata (Liliaceae), which has been shown to exert anti-cancer and immunomodulatory functions. In this study, we aimed to investigate anti-inflammatory activity of FC and the underlying molecular mechanism. RAW264.7 macrophages were stimulated with lipopolysaccharide (LPS) or pre-treated with FC prior to being stimulated with LPS. Thereafter, the macrophages were subjected to analysis of the expression levels of pro-inflammatory mediators, including nitric oxide (NO), prostaglandin E2 (PGE), tumor necrosis factor-α (TNF-α), interleukin-1β (IL-1β), and IL-6, as well as two relevant enzymes, inducible nitric oxide synthase (iNOS), and cyclooxygenase-2 (COX-2). The analysis revealed that FC administration blunted LPS-induced production of NO and PGE in a dose-dependent manner, while the expression of iNOS and COX-2 at both mRNA and protein levels was inhibited in LPS-stimulated macrophages pre-treated with FC. Moreover, LPS stimulation upregulated mRNA expression and medium release of TNF-α, IL-1β, and IL-6, whereas this effect was blocked upon FC pre-administration. Mechanistic studies showed that inhibitory effects of FC on LPS-induced inflammation were associated with a downregulation of IκB kinase, IκB, and p65/NF-κB pathway. Taken together, these data suggest that FC possesses an inflammation-suppressing activity, thus being a potential agent for the treatment of inflammation-associated disorders.
Inflammation, an essential part of innate immunity, constitutes the first line of defense to protect the body against invasive insults [1]. Amongst, macrophages are the characteristic cells that are activated in the early stage of pathogen infection [2]. However, excessively activated macrophages often lead to inflammatory diseases or autoimmune disorders. Thus, targeting macrophage activities is considered to be a promising strategy against excessive and extended inflammation [3,4]. Lipopolysaccharide (LPS) is a widely used stimulus extracted from the outer membrane of Gram-negative bacteria, which vigorously induces a number of inflammatory responses [5]. LPS binds to lipopolysaccharide binding protein and eventually interacts with the TLR4/MD-2 complex after being transferred to CD14. Subsequently, activation of the signaling pathways in macrophages leads to induction and secretion of inflammatory mediators and their associated enzymes, such as nitric oxide (NO) and inducible NO synthase (iNOS), prostaglandin E2 (PGE2) and cyclooxygenase-2 (COX-2), as well as interleukin-1β (IL-1β), IL-6, and tumor necrosis factor-alpha (TNF-α) [5]. Amongst, nuclear factor-kappa B (NF-κB) signaling has been identified as a common pathway for regulating the above-mentioned inflammatory responses in macrophages [6].
Paris formosana Hayata (Liliaceae) is a perennial herb mainly growing in the dark and humid forests of Taiwan, which has been used as a folk therapy for snake bite-elicited inflammation and tumors for a long time [7]. Formosanin C (FC) is the major diosgenin saponin that can be extracted with methanol from the herb. It has been suggested that FC displays inhibitory effects on many types of cancer, including liver, colorectal and lung cancers [8-10], while it promotes the effect of concanavalin A on lymphocyte proliferation [11]. Combined administration of FC and polyphyllin I or polyphyllin II produced a synergistic anti-tumor effect on cancer cells [12,13]. Recently, FC was found to be a novel ferroptosis inducer that exerts a potent anti-tumor effect through inducing ferritinophagy in human hepatocellular carcinoma cells [14]. However, it has yet to be determined whether FC has an anti-inflammatory activity.
This study aimed to investigate inhibitory effect of FC on LPS-stimulated inflammatory responses in RAW264.7 macrophages as well as the underlying mechanism. Here, we showed that FC possesses a potent anti-inflammatory activity as manifested by inhibition of multiple inflammatory mediators, enzymes and the upstream NF-κB signaling.
FC (purity ≥ 99%) and LPS (E. coli 055: B5) were purchased from the MedChemExpress (Monmouth Junction, NJ, USA) and Sigma Co. (St. Louis, MO, USA), respectively. RAW264.7 macrophages (American Type Culture Collection, Manassas, VA, USA) were cultured in Dulbecco’s Modified Eagles Medium supplemented with 10% fetal bovine serum and 1% antibiotics consisting of penicillin and streptomycin. Cells were maintained in a humidified incubator with 95% air and 5% CO2 at 37°C. 1 µg/ml LPS was used for stimulation, and FC was preincubated for 2 h.
Cell counting kit-8 (CCK8) assay was performed to evaluate the cytotoxicity of FC following the manufacturer’s instruction (Dojindo, Kumamoto, Japan). Macrophages at a density of 1 × 104 per well were seeded in 96-well plates. After an overnight culture, cells were treated as designated, and the culture medium was replaced with fresh medium containing 10% CCK-8 reagent. Following 2 h of incubation at 37°C, absorbance was read at 450 nm using a BioTek microplate reader (Winkowski, VT, USA).
Nitrite as a stable product of NO in the culture medium was measured using Griess reagents (Promega, Madison, WI, USA). Macrophages at a density of 1.5 × 104 per well were seeded in 96-well plates. After an overnight culture, cells were treated as designated. The medium was transferred to a new tube and then mixed with an equal volume of Griess reagent. Following 10 min of incubation in the darkness, absorbance was read at 540 nm using the BioTek reader.
Macrophages at a density of 1.5 × 105 per well were seeded in 24-well culture plates. After an overnight culture, cells were treated as designated, and the medium was collected. The levels of cytokines were measured by using respective ELISA kits (R&D System, Minneapolis, MN, USA) according to the enclosed protocols.
Macrophages at a density of 6 × 105 were seeded in 6-well culture plates. Following an overnight culture, cells were treated as designated and then collected for lysis in RIPA buffer (Solarbio, Beijing, China) containing phosphatase inhibitor cocktail (Sigma, Darmstadt, Germany) and 1 mM phenylmethylsulfonyl fluoride. Proteins were quantified using a BCA kit (Thermo Scientific, Waltham, MA, USA). After denaturation, the proteins (20 µg per lane) were resolved on SDS-PAGE gels and then blotted onto a PVDF membrane. After being blocked with 5% bovine serum albumin, the membrane was incubated overnight at 4°C with each of the following primary antibodies: p-IKK, IKK, p-IκB, IκB, p-p65, and p65 (Cell Signaling, Danvers, MA, USA); iNOS, COX-2 and GAPDH (Proteintech, Wuhan, China). Thereafter, the membrane was subjected to an incubation with mouse or rabbit HRP-conjugated secondary antibodies (GenDEPOT, Katy, TX, USA), and the targeted proteins were visualized by using an enhanced chemiluminescent kit (Bio-Rad Laboratories, Hercules, CA, USA). The ImageJ software (version 1.8.0; Bethesda, MD, USA) was used to quantify the density of protein bands.
Macrophages at a density of 6 × 105 per well were seeded in 6-well plates. Cells were cultured overnight and treated as designated. Thereafter, total RNA was extracted from the cells using Trizol reagent (Tiangen, Beijing, China). A reverse transcription was performed with a kit (Tiangen), and the resultant cDNA was PCR-amplified using the SYBR Mix reagents (Toyobo, Osaka, Japan). The expression level was calculated by the 2–ΔΔCT method and normalized to the reference gene GAPDH.
Macrophages at a density of 5 × 103 per well were seeded in 12-well chamber slides. Cells were cultured overnight and treated as designated. Cells were fixed in cold 100% methanol for 30 min and permeabilized in 0.3% Triton ×100 (Solarbio, in PBS) for 15 min. Then non-specific binding was blocked by 3% BSA (Sigma, in PBS) incubation for 1 h at room tempreture. Subsequently, the primary NF-κB p65 antibody (Proteintech, 1:100) was applied overnigh at 4°C, followed by incubation with the secondary Alexa Fluor 488 conjugated antibody (Proteintech, 1:400) for 1 h at room tempreture. After nuclear staining with DAPI (Solarbio), the images were captured under a immunofluorescence microscopy (Olympus, Tokyo, Japan).
The software of GraphPad Prism 8 (San Diego, CA, USA) was used for data analysis. Differences within two groups and those among multiple groups were analyzed by student t-test and one-way analysis of variance followed by post-hoc Dunn's test, respectively. Data were collected from at least three independent experiments and presented as mean ± standard deviation. A p-value < 0.05 was considered statistically significant.
To determine the appropriate concentration of FC that can be used in the experiments, cellular toxicity of FC in RAW264.7 macrophages was analyzed by the CCK-8 assay. In the experiments, the macrophages were treated with various concentrations of FC (0.625, 1.25, 2.5, 5, 10, and 20 µM) for 24 h. As depicted in Fig. 1, FC had no apparent cytotoxity on Raw264.7 cells with the concentration of 0.625, 1.25, 2.5, and 5 µM, which were chosen for the following experiments.
Given that macrophages release various types of inflammatory molecules including NO in response to LPS [15], we sought to determine whether FC administration affects the NO level in LPS-treated macrophages. As illustrated in Fig. 2A, FC treatment significantly attenuated LPS-induced NO production in a dose-dependent manner. We next investigated whether iNOS is involved in the inhibitory effect of FC in LPS-induced NO production. As expected, FC restrained LPS-stimulated increases in mRNA (Fig. 2B) and protein (Fig. 2C) levels of iNOS in RAW264.7 cells, suggesting that FC inhibits the NO production through down-regulating iNOS expression [16].
Effect of FC on LPS-elicited PGE production in macrophages was evaluated using the ELISA assay. As shown in Fig. 3A, while LPS stimulation led to a significant increase in PGE2 production, this effect was inhibited by pretreatment with FC. Likewise, we observed that LPS stimulation increased the expression of COX-2, a key enzyme of PGE biosynthesis [17], while FC significantly suppressed LPS-stimulated up-regulation of COX-2 at both mRNA and protein levels (Fig. 3B, C). Collectively, these data indicate that FC downregulates LPS-stimulated PGE production presumably through inhibiting the expression of COX-2.
The qPCR and ELISA assays were performed to examine the mRNA and protein levels of pro-inflammatory cytokines, respectively. Notably, FC treatment led to a dose-dependent reduction in both mRNA expression and medium release of IL-1β (Fig. 4A, D), IL-6 (Fig. 4B, E) and TNF-α (Fig. 4C, F). This observation demonstrated that FC exerts an anti-inflammatory effect by inhibiting production of pro-inflammatory cytokines.
Given that NF-κB signaling participates in regulating various inflammatory responses [18], we further investigated whether FC could suppress activation and nuclear translocation of p65/NF-κB. As illustrated in Fig. 5A, FC decreased the phosphorylation levels of IKK, IκBα and p65 without affecting the protein expression. Moreover, FC pretreatment restrained LPS-induced nuclear translocation of p65 (Fig. 5B). Together, these data indicate that FC inhibits LPS-induced inflammation through regulating NF-κB activation.
FC has been shown to possess immunomodulatory functions. For instance, FC was found to remarkably delay the onset of experimental autoimmune uveitis and reduce inflammatory reactions of the eyes [19]. It has also been reported that FC can lead to a retarded growth of the subcutaneously transplanted hepatoma, while this effect was associated with a modification of immune functions [7]. Moreover, FC displayed an immunomodulating effect on concanavalin A-induced proliferation of mouse lymphocytes [11]. However, the mechanisms underlying anti-inflammatory effects of FC remain to be determined. The current study demonstrated that FC inhibited LPS-induced inflammation in mouse RAW264.7 macrophages by decreasing various inflammatory mediators via inhibition of NF-κB activation.
Macrophages play a pivotal role in the immune responses as well as the host defense system [20]. Upon exposure to LPS, macrophages excessively produce inflammatory mediators and cytokines, such as NO and IL-6. iNOS is an essential enzyme that catalyzes NO production during inflammation [21]. Excessively produced NO may trigger apoptosis and oxidative stress, thus increasing inflammation [22]. PGE2 is mainly synthesized by the COX-2 enzyme. High expression of COX-2 is significant positively correlated with inflammation, tumor progression and metastasis [23]. Thus, targeting iNOS and COX-2 could be an important strategy for the treatment of inflammatory disorders. In the present study, we showed that FC blunted LPS-stimulated expression of iNOS and COX-2 as well as the resulting productions of NO and PGE2, indicating that FC exerts a potent anti-inflammatory effect on LPS-stimulated macrophages.
IL-1β and IL-6 are considered to be among the most potent cytokines and the earliest responding cytokines in response to inflammatory stimuli, respectively. They are both associated with pathogenesis and progression of inflammatory or autoimmune disorders [24]. In addition, TNF-α potently activates macrophages, accelerates the production of inflammatory mediators and aggravates the risk for inflammation-associated diseases [24]. Thus, a therapeutic principle for treating inflammatory related diseases is to suppress the above-mentioned cytokines. This study demonstrated that FC pretreatment can ameliorate LPS-stimulated expression of IL-6, IL-1β, and TNF-α at both mRNA and protein levels. Combined with the inhibition of NO and PGE via iNOS and COX-2 in macrophages treated with FC, the data on the expression of cytokines suggest that FC could act as an potent anti-inflammatory agent.
NF-κB is present primarily as a heterodimeric complex formed by the p65 and p50 subunits, which modulates the transcription of a number of genes associated with inflammation, cell growth, and immune response [25]. In the basal state, NF-κB is located in the cytoplasm and remains inactive due to its binding to IκBα. Upon LPS stimulation, IκB kinase (IKK) is activated; the activated IKK then elicits IκB phosphorylation and subsequent nuclear translocation of the p65 protein [6]. Here, we found that FC remarkably attenuated LPS-stimulated activation of NF-κB signaling as manifested by the p65 nuclear translocation. Hence, inhibition of NF-κB signaling may underlie the anti-inflammatory effect of FC.
It has been shown that TLR4-mediated activation of MAPK (p38, JNK, and ERK) mediates LPS-induced activation of NF-κB, leading to the production of pro-inflammatory mediators [26]. PI3K/AKT signaling pathway acts as a crucial upstream regulator of NF-κB activation by targeting the trans-activation domain of NF-κB p65 [27]. FC was reported to attenuate VEGF-induced activation of AKT and ERK in endothelial cells [28]. It remains to be determined whether MAPK and PI3K/AKT are involved in LPS-induced NF-κB activation and inflammation. In the present study, we provided evidence that FC noticeably ameliorated LPS-induced productions of NO and PGE as well as IL-6, IL-1β, and TNF-α through inhibiting NF-κB activation, suggestive of FC as a potential therapeutic drug for inflammation-associated disorders.
ACKNOWLEDGEMENTS
The work was supported by the Wenling Municipal Science and Technology Bureau (2017C311081).
Notes
REFERENCES
1. Newton K, Manning G. 2016; Necroptosis and inflammation. Annu Rev Biochem. 85:743–763. DOI: 10.1146/annurev-biochem-060815-014830. PMID: 26865533.


2. Bruscia EM, Bonfield TL. 2016; Cystic fibrosis lung immunity: the role of the macrophage. J Innate Immun. 8:550–563. DOI: 10.1159/000446825. PMID: 27336915. PMCID: PMC5089923.


3. Liu XH, Pan LL, Jia YL, Wu D, Xiong QH, Wang Y, Zhu YZ. 2013; A novel compound DSC suppresses lipopolysaccharide-induced inflammatory responses by inhibition of Akt/NF-κB signalling in macrophages. Eur J Pharmacol. 708:8–13. DOI: 10.1016/j.ejphar.2013.01.013. PMID: 23353591.


4. Moncada S. 1999; Nitric oxide: discovery and impact on clinical medicine. J R Soc Med. 92:164–169. DOI: 10.1177/014107689909200402. PMID: 10450191. PMCID: PMC1297136.


5. Miller SI, Ernst RK, Bader MW. 2005; LPS, TLR4 and infectious disease diversity. Nat Rev Microbiol. 3:36–46. DOI: 10.1038/nrmicro1068. PMID: 15608698.


6. Viatour P, Merville MP, Bours V, Chariot A. 2005; Phosphorylation of NF-kappaB and IkappaB proteins: implications in cancer and inflammation. Trends Biochem Sci. 30:43–52. DOI: 10.1016/j.tibs.2004.11.009. PMID: 15653325.
7. Wu RT, Chiang HC, Fu WC, Chien KY, Chung YM, Horng LY. 1990; Formosanin-C, an immunomodulator with antitumor activity. Int J Immunopharmacol. 12:777–786. DOI: 10.1016/0192-0561(90)90042-L. PMID: 2292459.
8. Lee JC, Su CL, Chen LL, Won SJ. 2009; Formosanin C-induced apoptosis requires activation of caspase-2 and change of mitochondrial membrane potential. Cancer Sci. 100:503–513. DOI: 10.1111/j.1349-7006.2008.01057.x. PMID: 19154411.


9. Li Y, Man S, Li J, Chai H, Fan W, Liu Z, Gao W. 2014; The antitumor effect of formosanin C on HepG2 cell as revealed by 1H-NMR based metabolic profiling. Chem Biol Interact. 220:193–199. DOI: 10.1016/j.cbi.2014.06.023. PMID: 25014414.


10. Man S, Gao W, Zhang Y, Liu Z, Yan L, Huang L, Liu C. 2011; Formosanin C-inhibited pulmonary metastasis through repression of matrix metalloproteinases on mouse lung adenocarcinoma. Cancer Biol Ther. 11:592–598. DOI: 10.4161/cbt.11.6.14668. PMID: 21304274.


11. Chiang HC, Wang JJ, Wu RT. 1992; Immunomodulating effects of the hydrolysis products of formosanin C and beta-ecdysone from Paris formosana Hayata. Anticancer Res. 12:1475–1478. PMID: 1444209.
12. Cui J, Man S, Cui N, Yang L, Guo Q, Ma L, Gao W. 2019; The synergistic anticancer effect of formosanin C and polyphyllin VII based on caspase-mediated cleavage of Beclin1 inhibiting autophagy and promoting apoptosis. Cell Prolif. 52:e12520. DOI: 10.1111/cpr.12520. PMID: 30338602. PMCID: PMC6430456.


13. Liu J, Man S, Liu Z, Ma L, Gao W. 2016; A synergistic antitumor effect of polyphyllin I and formosanin C on hepatocarcinoma cells. Bioorg Med Chem Lett. 26:4970–4975. DOI: 10.1016/j.bmcl.2016.09.005. PMID: 27623551.


14. Lin PL, Tang HH, Wu SY, Shaw NS, Su CL. 2020; Saponin formosanin C-induced ferritinophagy and ferroptosis in human hepatocellular carcinoma cells. Antioxidants (Basel). 9:682. DOI: 10.3390/antiox9080682. PMID: 32751249. PMCID: PMC7463707.


15. Gu I, Brownmiller C, Stebbins NB, Mauromoustakos A, Howard L, Lee SO. 2020; Berry phenolic and volatile extracts inhibit pro-inflammatory cytokine secretion in LPS-stimulated RAW264.7 cells through suppression of NF-κB signaling pathway. Antioxidants (Basel). 9:871. DOI: 10.3390/antiox9090871. PMID: 32942640. PMCID: PMC7554842.


16. Rod-In W, Monmai C, Shin IS, You S, Park WJ. 2020; Neutral lipids, glycolipids, and phospholipids, isolated from sandfish (Arctoscopus japonicus) eggs, exhibit anti-inflammatory activity in LPS-stimulated RAW264.7 cells through NF-κB and MAPKs pathways. Mar Drugs. 18:480. DOI: 10.3390/md18090480. PMID: 32967264. PMCID: PMC7550997.


17. Tao MQ, Ji CL, Wu YJ, Dong JY, Li Y, Olatunji OJ, Zuo J. 2020; 1,7-Dihydroxy-3,4-dimethoxyxanthone inhibits lipopolysaccharide-induced inflammation in RAW264.7 macrophages by suppressing TLR4/NF-κB signaling cascades. Inflammation. 43:1821–1831. DOI: 10.1007/s10753-020-01256-3. PMID: 32468498.


18. Gao XH, Zhang SD, Wang LT, Yu L, Zhao XL, Ni HY, Wang YQ, Wang JD, Shan CH, Fu YJ. 2020; Anti-inflammatory effects of neochlorogenic acid extract from mulberry leaf (Morus alba L.) against LPS-stimulated inflammatory response through mediating the AMPK/Nrf2 signaling pathway in A549 cells. Molecules. 25:1385. DOI: 10.3390/molecules25061385. PMID: 32197466. PMCID: PMC7144357.
19. Wu RT, Lin WJ, Chiang HC, Horng LY, Chung YM. 1990; Modulation of experimental autoimmune uveitis with formosanin-C in guinea pigs. J Ocul Pharmacol. 6:301–311. DOI: 10.1089/jop.1990.6.301. PMID: 2097314.


20. Gao H, Liu X, Sun W, Kang N, Liu Y, Yang S, Xu QM, Wang C, Chen X. 2017; Total tanshinones exhibits anti-inflammatory effects through blocking TLR4 dimerization via the MyD88 pathway. Cell Death Dis. 8:e3004. DOI: 10.1038/cddis.2017.389. PMID: 28817116. PMCID: PMC5596575.


21. Gao H, Cui Y, Kang N, Liu X, Liu Y, Zou Y, Zhang Z, Li X, Yang S, Li J, Wang C, Xu QM, Chen X. 2017; Isoacteoside, a dihydroxyphenylethyl glycoside, exhibits anti-inflammatory effects through blocking toll-like receptor 4 dimerization. Br J Pharmacol. 174:2880–2896. DOI: 10.1111/bph.13912. PMID: 28616865. PMCID: PMC5554315.


22. Chen CC, Lin MW, Liang CJ, Wang SH. 2016; The anti-inflammatory effects and mechanisms of eupafolin in lipopolysaccharide-induced inflammatory responses in RAW264.7 macrophages. PLoS One. 11:e0158662. DOI: 10.1371/journal.pone.0158662. PMID: 27414646. PMCID: PMC4945065.


23. Yoo S, Kim MY, Cho JY. 2017; Beauvericin, a cyclic peptide, inhibits inflammatory responses in macrophages by inhibiting the NF-κB pathway. Korean J Physiol Pharmacol. 21:449–456. DOI: 10.4196/kjpp.2017.21.4.449. PMID: 28706459. PMCID: PMC5507784.


24. Rossol M, Heine H, Meusch U, Quandt D, Klein C, Sweet MJ, Hauschildt S. 2011; LPS-induced cytokine production in human monocytes and macrophages. Crit Rev Immunol. 31:379–446. DOI: 10.1615/CritRevImmunol.v31.i5.20. PMID: 22142165.


25. Oeckinghaus A, Ghosh S. 2009; The NF-kappaB family of transcription factors and its regulation. Cold Spring Harb Perspect Biol. 1:a000034. DOI: 10.1101/cshperspect.a000034. PMID: 20066092. PMCID: PMC2773619.
26. Xu Z, Lin R, Hou X, Wu J, Zhao W, Ma H, Fan Z, Li S, Zhu Y, Zhang D. 2020; Immunomodulatory mechanism of a purified polysaccharide isolated from Isaria cicadae Miquel on RAW264.7 cells via activating TLR4-MAPK-NF-κB signaling pathway. Int J Biol Macromol. 164:4329–4338. DOI: 10.1016/j.ijbiomac.2020.09.035. PMID: 32926903.


27. Madrid LV, Mayo MW, Reuther JY, Baldwin AS Jr. 2001; Akt stimulates the transactivation potential of the RelA/p65 Subunit of NF-kappa B through utilization of the Ikappa B kinase and activation of the mitogen-activated protein kinase p38. J Biol Chem. 276:18934–18940. DOI: 10.1074/jbc.M101103200. PMID: 11259436.
28. Xiao X, Yang M, Xiao J, Zou J, Huang Q, Yang K, Zhang B, Yang F, Liu S, Wang H, Bai P. 2014; Paris Saponin II suppresses the growth of human ovarian cancer xenografts via modulating VEGF-mediated angiogenesis and tumor cell migration. Cancer Chemother Pharmacol. 73:807–818. DOI: 10.1007/s00280-014-2408-x. PMID: 24638862.


Fig. 1
Cytotoxicity analysis of FC in macrophages.
The cytotoxicity of FC in RAW264.7 macrophages was measured by CCK-8 assay. The macrophages were treated with various concentrations of FC (0, 0.625, 1.25, 2.5, 5, 10, and 20 μM) for 24 h. n = 3; data were presented as means ± SD. FC, formosanin C; CCK, cell counting kit-8. *Indicates p < 0.05 vs. the control sample.
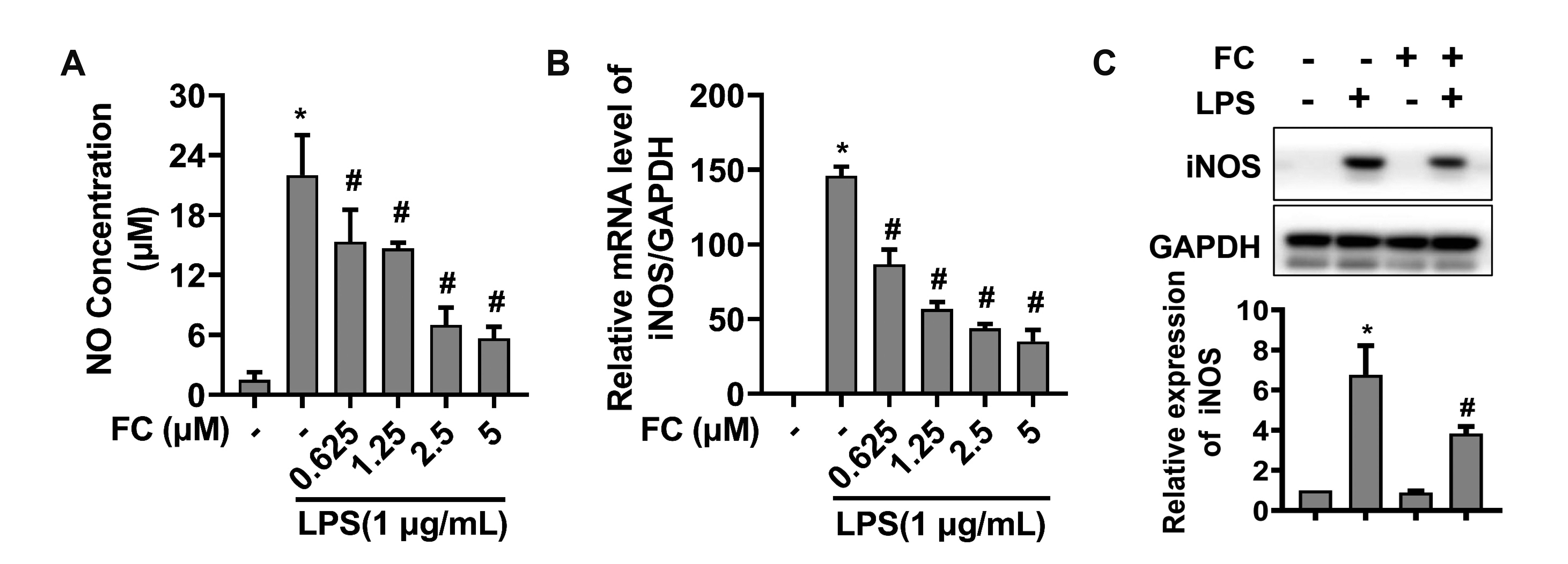
Fig. 2
Effects of FC on LPS-elicited NO production and iNOS expression.
(A) NO production was examined by Griess assay. (B) The mRNA expression of iNOS was evaluated by qPCR. (C) The protein expression of iNOS was assessed by Western blotting. RAW264.7 macrophages were treated with FC for 2 h and then subjected to an stimulation with 1 μg/ml LPS for 22 h. n = 3; data were presented as means ± SD. FC, formosanin C; LPS, lipopolysaccharide; NO, nitric oxide; iNOS, inducible nitric oxide synthase. *Indicates p < 0.05 vs. the control group; #indicates p < 0.05 vs. the LPS alone group.
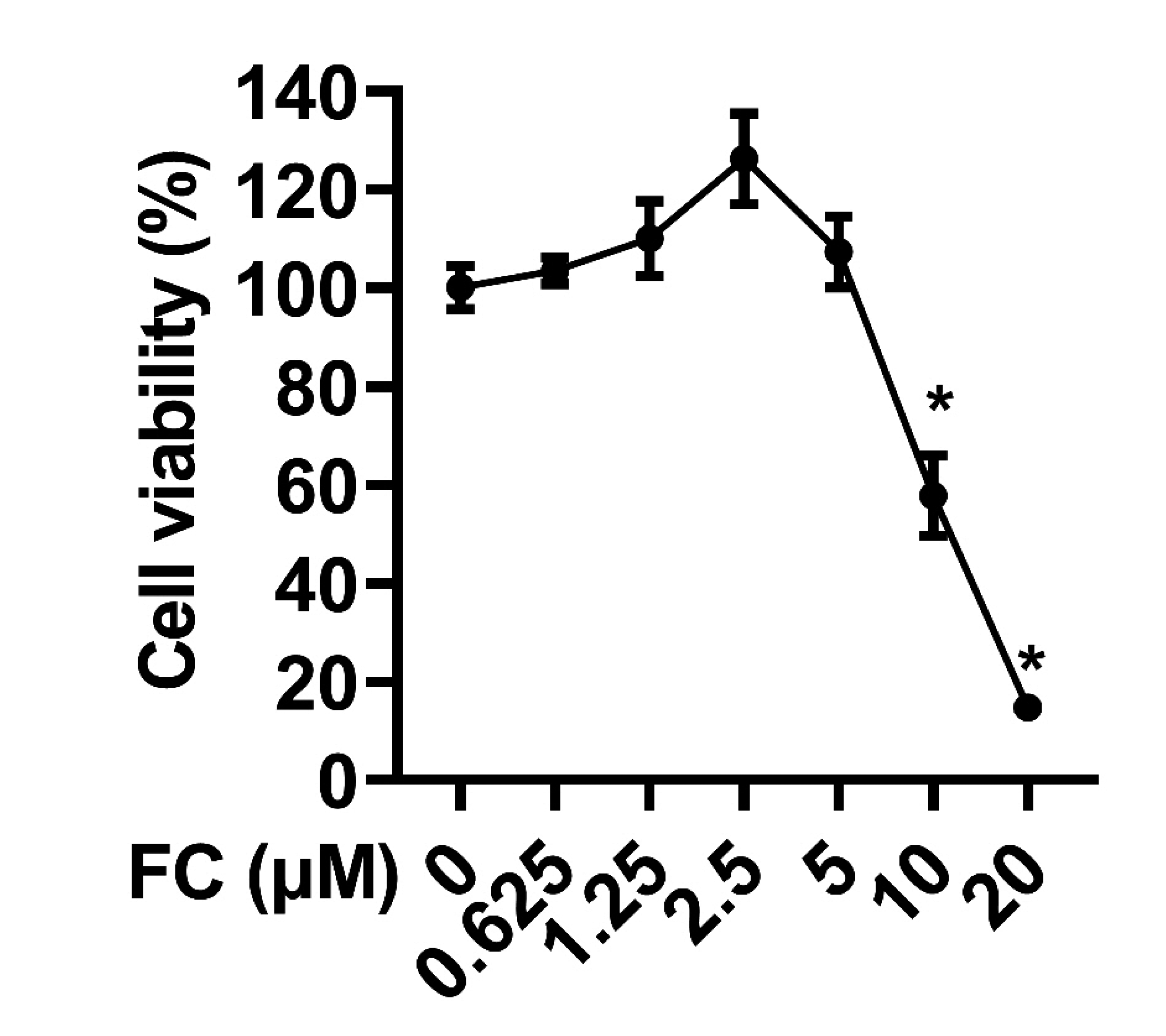
Fig. 3
Effects of FC on LPS-elicited PGE production and COX-2 expression.
(A) PGE production was examined by ELISA assay. (B) The mRNA levels of COX-2 was assessed by qPCR. (C) The protein levels of COX-2 was determined using Western blot analysis. RAW264.7 macrophages were pretreated with FC for 2 h and then subjected to an stimulation with 1 μg/ml LPS for 22 h. n = 3; data were presented as means ± SD. FC, formosanin C; LPS, lipopolysaccharide; PGE, prostaglandin E2; COX-2, cyclooxygenase-2. *Indicates p < 0.05 vs. the control group; #indicates p < 0.05 vs. the LPS alone group.
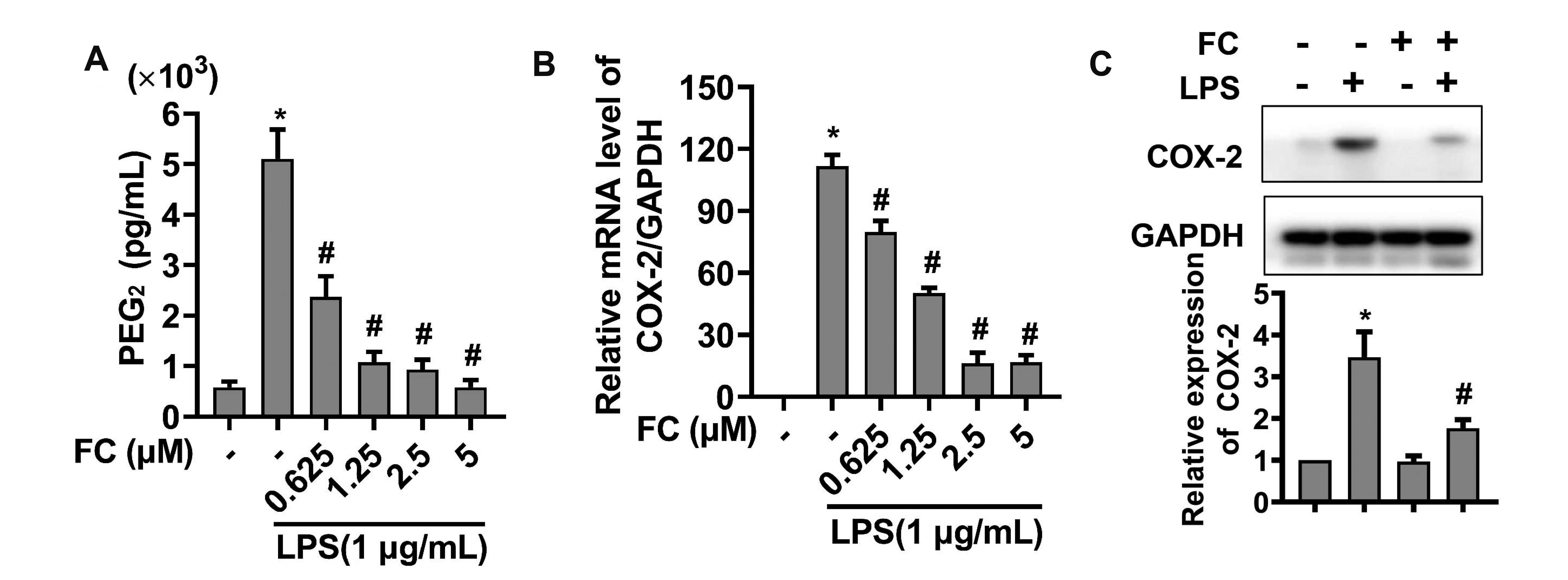
Fig. 4
Effects of FC on LPS-elicited releases of pro-inflammatory cytokines.
(A–C) The mRNA expression of IL-1β (A), IL-6 (B) and TNF-α (C) was evaluated by qPCR. (D–F) The production of IL-1β (D), IL-6 (E) and TNF-α. (F) was assessed by ELISA assays. RAW264.7 macrophages were pretreated with FC for 2 h and then subjected to an stimulation with 1 μg/ml LPS for 22 h. n = 3; data were presented as means ± SD. FC, formosanin C; LPS, lipopolysaccharide; TNF-α, tumor necrosis factor-α; IL-1β, interleukin-1β. *Indicates p < 0.05 vs. the control group; #indicates p < 0.05 vs. the LPS alone group.
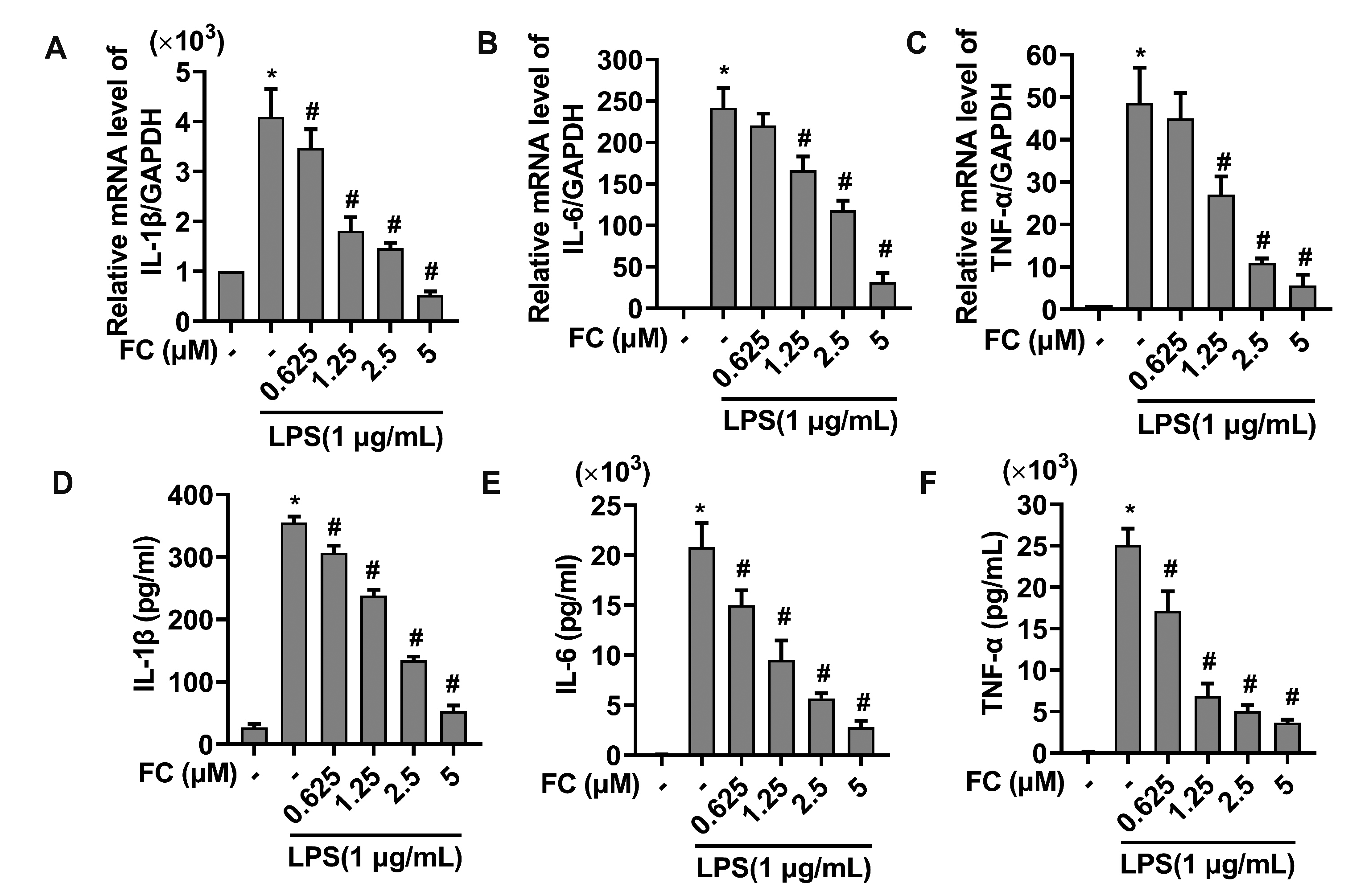
Fig. 5
FC inhibited the activation of NF-κB pathway.
(A) The phosphorylation levels of IKK, IκBα and p65 were assessed by Western blot analysis. (B) The fluorescence microscopy imaging was taken. Blue and green indicate nuclei and p65, respectively; scale bar, 50 μm. RAW264.7 macrophages were pretreated with FC for 2 h and then subjected to an stimulation with 1 μg/ml LPS for 18 h. n = 3; data were presented as means ± SD. FC, formosanin C; NF-κB, nuclear factor-kappa B; IKK, IκB kinase; LPS, lipopolysaccharide. *Indicates p < 0.05 vs. the control group; #indicates p < 0.05 vs. the LPS alone group.
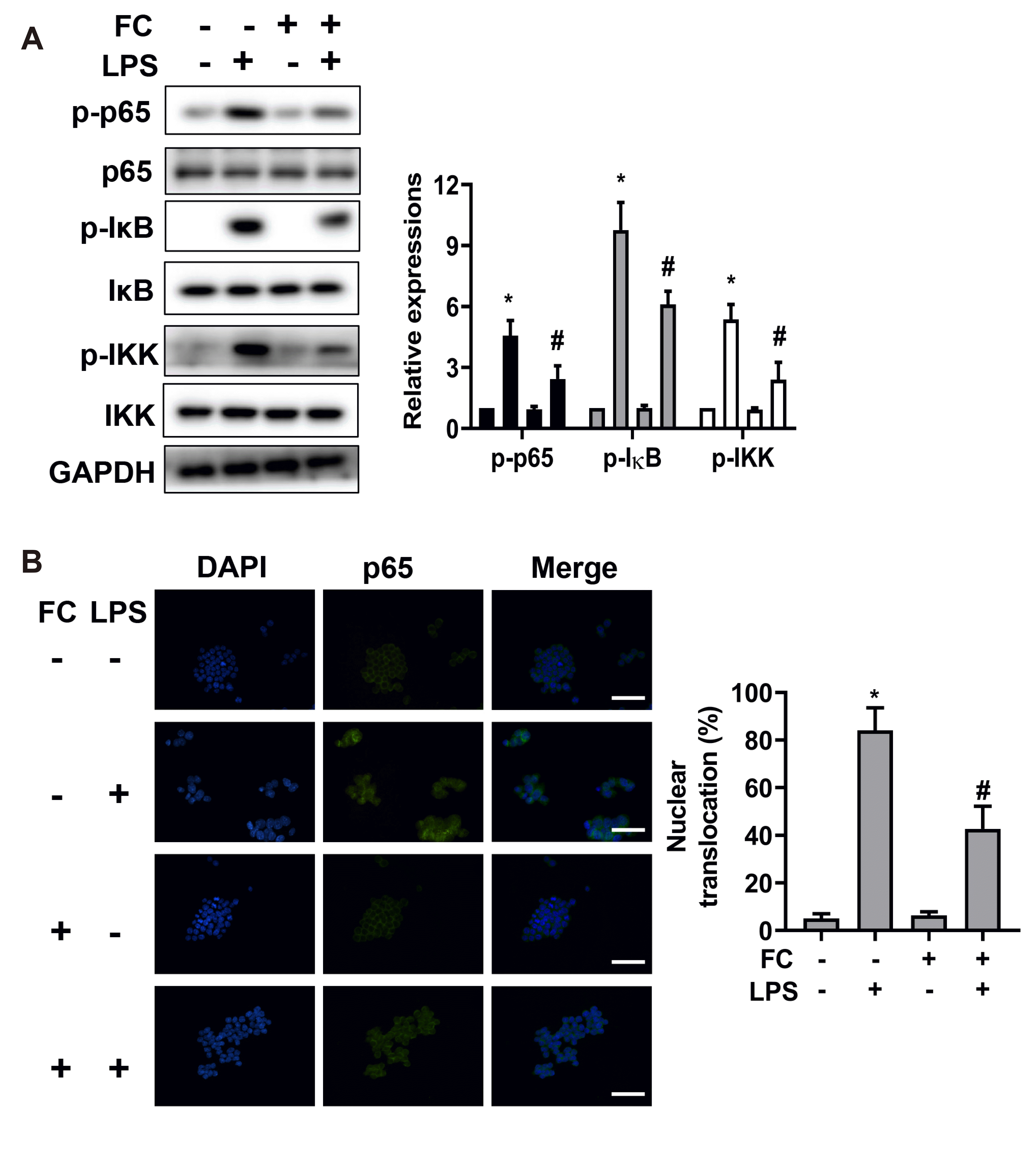