This article has been
cited by other articles in ScienceCentral.
Abstract
Background and Objectives
There is little emphasis on the efficacy of catheter ablation for ventricular arrhythmia (VA) when using VA burden reduction as a marker for success. We examined the efficacy of catheter ablation using VA burden, rather than VA recurrence as a marker of success, following catheter ablation of structural heart disease (SHD) related VA.
Methods
Catheter ablation of SHD related VA was performed at a single centre over 4-years. VA episodes and implantable cardioverter defibrillator (ICD) therapies were recorded over the 6-months before and after final ablation. Outcomes were reported in terms of burden reduction and compared to singular VA recurrence.
Results
Overall, 108 patients were included in the study. Mean age 64.2±13.9 years, 86% male, mean left ventricular ejection fraction (LVEF) 42±16%. Median VA episodes and ICD therapy were significantly reduced after ablation (VA before: 10 [interquartile range, IQR: 2–38] vs. VA after: 0 [IQR: 0–2], p<0.001; anti–tachycardia pacing [ATP] before: 16 (IQR: 1.5–57) vs. ATP after: 0 [IQR: 0–2], p<0.001; shocks before: 1 [IQR: 0–5] vs. shocks after: 0 [IQR: 0–0], p<0.001). Procedural success at 6-months was significantly higher when considering ≥75% reduction in VA burden, rather than a singular VA-free survival (83% vs. 67%, p=0.001).
Conclusions
The vast majority (>80%) of patients achieve reduction in VA burden (≥75% reduction) after catheter ablation for VA. This data suggests that catheter ablation is highly therapeutic when procedure success is defined as reduction in VA, rather than using a single VA recurrence as a metric for failure.
Keywords: Ventricular tachycardia, Catheter ablation, Cardiomyopathy, Sudden cardiac death, Defibrillator
INTRODUCTION
Catheter ablation is indicated to treat structural heart disease (SHD) related ventricular arrhythmias (VA) refractory to anti-arrhythmic drugs (AADs) and when implantable cardioverter defibrillator (ICD) therapies persist.
1) AADs are frequently poorly tolerated with significant side effects, and recurrent ICD shocks have been shown to be associated with increased mortality and reduced quality of life.
2)3) Catheter ablation for recurrent VA aims to cure the VA but also reduce reliance on AADs and decrease ICD therapies. The traditional marker of long-term success following ablation is freedom from VA recurrence, with one of the largest studies to date, reporting VA free survival at 6-years in 54% of patients with ischemic cardiomyopathy (ICM) and 38% of patients with non-ischemic cardiomyopathy (NICM).
4) Despite benefitting from potentially significant reductions in ICD therapies along with decreased reliance on AADs, documentation of VA recurrence is often classed as a failure, with the overall positive impact of catheter ablation potentially underestimated.
Several studies have documented VA burden reduction as a marker of long-term success following ablation. Most notably in a large ICM population in the Thermocool VT Trial,
5) and with smaller populations in the use of novel ablation techniques such as non-invasive stereotactic body radiotherapy
6) and renal sympathetic denervation therapy.
7)
In this study we describe the effect of catheter ablation on burden of VA and ICD therapies through systematic device interrogation, over a 6-month period, before and after final ablation. We sought to determine that effective VA and ICD therapy burden reduction following catheter ablation would provide a more realistic representation of the success of catheter ablation, rather than using a singular recurrence in follow up, analysed as VA-free survival.
METHODS
Between February 2016 and July 2019, 108 consecutive patients with SHD related VA and an ICD in situ, underwent catheter ablation at a single tertiary referral centre (Westmead Hospital, Sydney, Australia), and were included in the present retrospective analysis.
Transthoracic echocardiography ± cardiac magnetic resonance imaging was performed on all patients prior to procedure, to assess for SHD and define ventricular function. SHD included ICM and NICM, with the latter comprising idiopathic dilated cardiomyopathy, genetic cardiomyopathies, infiltrative cardiomyopathies, chemotherapy induced cardiomyopathy, congenital heart disease and valvular heart disease (not secondary to an ischemic aetiology). Distinction between ICM and NICM was made by the presence or absence of relevant coronary artery disease, diagnosed by coronary angiography. NICM was identified based on the absence of relevant coronary artery disease and defined as per the European Society of Cardiology Working Group on Myocardial and Pericardial Diseases.
8) Study analysis was performed according to protocols approved by the Western Sydney Local Health District Human Research Ethics Committee. All participants gave written informed consent.
Mapping
Our approach to mapping and ablation of scar-related ventricular tachycardia (VT) has been described previously.
9)10) Procedures were performed under either conscious sedation or general anaesthesia. An SL3 sheath (Abbott Medical, Abbott Park, IL, USA) was used to perform coronary sinus venography and a decapolar catheter inserted into the coronary sinus. A quadripolar catheter was deployed to the right ventricle (RV) apex. AAD therapy was withheld for 5 half-lives pre-ablation (except in the case of an emergent procedure or with amiodarone). Systemic anticoagulation was administered after sheath insertion using intravenous unfractionated heparin to maintain an activated clotting time of ≥400 seconds prior to left ventricular (LV) access or ≥250 seconds for RV access, unless epicardial approach was planned. If an epicardial approach was planned, anticoagulation was commenced after safe epicardial access was established. ICDs were re-programmed to disable therapies prior to ablation and re-enabled at the end of the procedure. The endocardial LV was accessed either transseptally (Large curve Agilis™, Abbott Medical), or anterogradely (SL1 8.5 Fr, Abbott Medical), or both. In patients with previously failed endocardial catheter ablation or if pre-procedural imaging strongly indicated intramural/epicardial substrate, epicardial access (via a percutaneous approach) was obtained. Coronary angiography was performed prior to epicardial ablation to avoid coronary artery injury. High output pacing (10 mA and 9 ms output) was performed to exclude phrenic nerve stimulation.
Three-dimensional (3D) electroanatomic mapping (EAM) of the RV, LV (or both) was performed using either the CARTO® EAM system (Biosense Webster, Inc., Diamond Bar, CA, USA), EnSite Precision™ (Abbott Medical) or RHYTHMIA HDx™ (Boston Scientific, Natick, MA, USA: only in 1 case). High-resolution multielectrode mapping catheters were predominantly used; Advisor HD Grid™ or Livewire Duodeca™ (Abbott Medical), alternatively Pentaray® or DecaNav® (Biosense Webster Inc.) with CARTO® and the INTELLIMAP ORION™ (Boston Scientific) with RHYTHMIA HDx™. In the EAM system and Cardiolab EP recording system, band pass filtering was 30–500 Hz. An endocardial and/or epicardial 3D-shell of chamber geometry was constructed for each ventricle with electrogram recordings during the patient's native rhythm (sinus or paced rhythm) or paced rhythm (RV or biventricular pacing). Activation maps of each VT were obtained if the rhythm was sustained and hemodynamically tolerated; additional substrate-based mapping was followed in all patients.
Conventional ventricular bipolar substrate voltage parameters were used (dense scar: <0.5 mV, low voltage: 0.5–1.5 mV, normal >1.5 mV).
11) Unipolar low voltage was defined as electrogram amplitude <8.3 mV (LV),
12) and <5.5 mV (RV).
13) The chamber mapped was based on the characteristics of the induced or spontaneously occurring VT. Intracardiac echocardiography (ICE) was used whenever feasible, with image integration where possible (CARTO
®, Biosense Webster Inc.).
Ablation
Radiofrequency (RF) ablation was predominantly performed using a 3.5-millimetre tip open-irrigation catheter ThermoCool® STSF (Biosense Webster Inc.), or the Tacticath™ SE catheter (Abbott Medical). Ablation was routinely delivered with a contact force (CF) of ≥10grams. RF energy of up to 50 Watts was delivered, aiming for an impedance drop of between 10–20 Ω. When available, real time visualisation of the catheter tip using ICE, guided ablation ensuring adequate tissue contact and catheter stability along with lesion formation.
Ablation was guided by substrate, and/or activation mapping. Ablation targeted presumptive isthmus and exits, based on activation and entrainment mapping, if the VT was hemodynamically tolerated. If the VT was not tolerated or short in duration, a substrate-based ablation was performed for scar-related VTs.
10) The specific approach targeted presumptive channels and exits as determined by paced QRS morphology matched against the VT QRS morphology with a stimulus-to-QRS interval >40 ms, abnormal fractionated potentials, double potentials, late potentials during sinus and paced rhythm, and local abnormal ventricular activities.
14)
Ventricular tachycardia induction protocol
Programmed electrical stimulation (PES) was performed from at least 2 RV sites using a 400 ms drive train with 4 extra-stimuli beginning at 300 ms, decrementing by 10 ms down to ventricular refractoriness. LV stimulation was used if VA was non-inducible after RV stimulation. This was followed by burst RV pacing down to ventricular refractoriness from the RV apex. PES and burst RV pacing were then repeated from each site using the highest tolerated dose of isoprenaline (up to 40 µg/min) with hemodynamic support to maintain perfusion pressure with inotropic or mechanical circulatory support initiated at the start of the case. Isoprenaline was initially commenced at 10 µg/min, with the PES and RV burst pacing protocol repeated after incrementing the isoprenaline dose by 10 µg/min. Sustained VT was defined as monomorphic ventricular arrhythmia with duration >10 seconds. The same induction protocol was repeated post ablation.
Definitions of clinical and non-clinical ventricular tachycardia
Clinical VT was defined as any inducible VT that had a 12-lead electrocardiogram (ECG) morphology and rate (within 20 ms) matching a VT documented to have occurred spontaneously before ablation; only the rate cut-off and intracardiac electrogram data from the implanted ICD (if present) were used when the 12-lead VT morphology was not available before ablation. Non-clinical VTs were inducible VTs that did not have an identical rate (>20 ms difference) or 12-lead ECG morphology to the clinical VT documented before ablation.
Outcomes
Acute procedural outcomes were reported as follows:
1. Complete success was defined as non-inducibility of any VT at the end of the procedure.
2. Partial success was defined as non-inducibility of at least one clinical VT morphology but other spontaneous or undocumented VTs remain.
3. Failure was defined as the inducibility of the clinical VT at the end of the procedure.
In follow-up, outcomes were reported as follows:
1. VA burden after the final ablation: defined as any sustained VA greater than or equal to 30 seconds, VA requiring ICD therapy, VA requiring external cardioversion.
2. ICD therapy burden: defined as any appropriate ATP or shock therapy.
3. VA free survival after the final ablation: defined as any sustained VA greater than or equal to 30 seconds, VA requiring ICD therapy or external cardioversion, VA resulting in hospitalisation or need for repeat ablation procedure.
4. All-cause mortality.
5. Cardiac transplantation.
Collection of ventricular arrhythmia burden preceding ablation and follow up
The individual burden of sustained VA, ATP and shocks were collected for a 6-month period before and after each patient's final ablation during the study period. This data was collected from the device interrogation reports, clinic consultations, inpatient hospital medical records and remote downloads. In the 9% of patients with no ICD in situ before ablation, VA burden was calculated based on captured ECG evidence (either through a Holter ECG, permanent pacemaker, implantable loop recorder or ECG monitoring during hospital admission). In all patients before and after ablation, each episode of sustained VA, ATP or shock was counted as a single episode. Likewise, in episodes of VT storm, each separate VA episode, ATP burst, or shock were counted as an individual episode.
Post ablation ICD reprogramming consisted of two VT treatment zones. The first zone was programmed below the rate of the slowest VT (with or without ATP) and the second zone programmed at a minimum detection rate >188 bpm, programmed to deliver a shock (with or without ATP). All patients were enrolled in a remote monitoring service, managed either by Westmead Hospital or referring cardiologist. All ICD activations were recorded, logged and transmitted to the clinic, which prompted an in-office visit for detailed evaluation of clinical and device data. Each patient was followed up for 6-months after their ablation, or until cardiac transplant or death.
Definition of major complications
Major complications were defined as any major vascular complications requiring transfusion, endovascular or surgical treatment, any thromboembolic event (including stroke, systemic or pulmonary emboli), atrioventricular block (inadvertent or anticipated from RF ablation), pericardial effusion requiring intervention, heart failure, electromechanical dissociation, coronary or phrenic injury.
Statistical analysis
SPSS version 25 (IBM Corp., Armonk, NY, USA) was used for analysis. Continuous variables were expressed as mean ± SD if normally distributed; median and 25–75% interquartile range (IQR) or full ranges were used if the data were clearly skewed. Continuous variables were compared using a student t-test when normally distributed, or a Mann-Whitney U test when they were not normally distributed. The χ2 or Fisher's exact test were used when comparing categorical variables. Survival free of VA was estimated using the Kaplan-Meier method and the log rank χ2 method. Cox proportional hazard models were created to determine predictors of VA recurrence. Hazard ratios and 95% confidence intervals were used to express risk of VA recurrence. A 2-tailed p value of <0.05 was considered statistically significant.
RESULTS
Study population
A total of 124 patients underwent catheter ablation of SHD related VA during the study period. There were 108 patients who had either sustained VT or premature ventricular complex (PVC) induced ventricular fibrillation (VF) as the indication for ablation and had an ICD in situ before or after final ablation. These patients were included in this retrospective analysis. There was 1 patient in whom there was no available post procedure burden data, this patient was excluded from the VA burden and recurrence analysis. Median follow up after final ablation was 183 (IQR: 183–183) days.
Baseline characteristics
The baseline patient characteristics are summarised in
Table 1. Patients were predominantly male (86%), with impaired LV ejection fraction (mean, 42±15%), secondary to comparable proportions of ICM (48%) and NICM (52%). The majority of patients (91%) had an ICD in situ prior to ablation, the remaining patients (9%) received an ICD following ablation. Before ablation, 39% of patients had failed amiodarone and median AAD use was 1 (IQR: 1–2). Within the SHD subgroups, 18/52 (35%) of the ICM patients had undergone coronary artery bypass graft surgery prior to ablation and in the heterogenous NICM subgroup, idiopathic dilated cardiomyopathy (DCM) accounted for 31/56 (55%) of the cases.
Table 1
Baseline patient characteristics
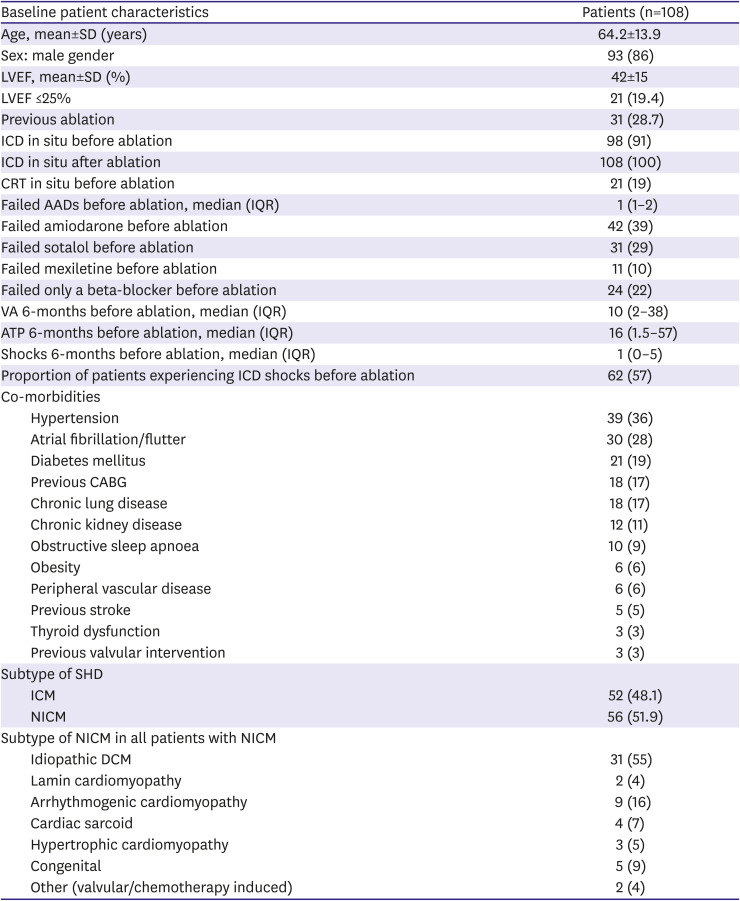
Baseline patient characteristics |
Patients (n=108) |
Age, mean±SD (years) |
64.2±13.9 |
Sex: male gender |
93 (86) |
LVEF, mean±SD (%) |
42±15 |
LVEF ≤25% |
21 (19.4) |
Previous ablation |
31 (28.7) |
ICD in situ before ablation |
98 (91) |
ICD in situ after ablation |
108 (100) |
CRT in situ before ablation |
21 (19) |
Failed AADs before ablation, median (IQR) |
1 (1–2) |
Failed amiodarone before ablation |
42 (39) |
Failed sotalol before ablation |
31 (29) |
Failed mexiletine before ablation |
11 (10) |
Failed only a beta-blocker before ablation |
24 (22) |
VA 6-months before ablation, median (IQR) |
10 (2–38) |
ATP 6-months before ablation, median (IQR) |
16 (1.5–57) |
Shocks 6-months before ablation, median (IQR) |
1 (0–5) |
Proportion of patients experiencing ICD shocks before ablation |
62 (57) |
Co-morbidities |
|
|
Hypertension |
39 (36) |
|
Atrial fibrillation/flutter |
30 (28) |
|
Diabetes mellitus |
21 (19) |
|
Previous CABG |
18 (17) |
|
Chronic lung disease |
18 (17) |
|
Chronic kidney disease |
12 (11) |
|
Obstructive sleep apnoea |
10 (9) |
|
Obesity |
6 (6) |
|
Peripheral vascular disease |
6 (6) |
|
Previous stroke |
5 (5) |
|
Thyroid dysfunction |
3 (3) |
|
Previous valvular intervention |
3 (3) |
Subtype of SHD |
|
|
ICM |
52 (48.1) |
|
NICM |
56 (51.9) |
Subtype of NICM in all patients with NICM |
|
|
Idiopathic DCM |
31 (55) |
|
Lamin cardiomyopathy |
2 (4) |
|
Arrhythmogenic cardiomyopathy |
9 (16) |
|
Cardiac sarcoid |
4 (7) |
|
Hypertrophic cardiomyopathy |
3 (5) |
|
Congenital |
5 (9) |
|
Other (valvular/chemotherapy induced) |
2 (4) |
Procedural characteristics
The procedural characteristics are summarised in
Table 2. VT storm was the most common indication for ablation (63%), followed by sustained VT (excluding storm; 34%), and PVC induced VF (3%). Overall, 65% of procedures used high-density multielectrode mapping catheters, 68% used CF-sensing ablation catheters and 60% used ICE.
Table 2
Procedural characteristics
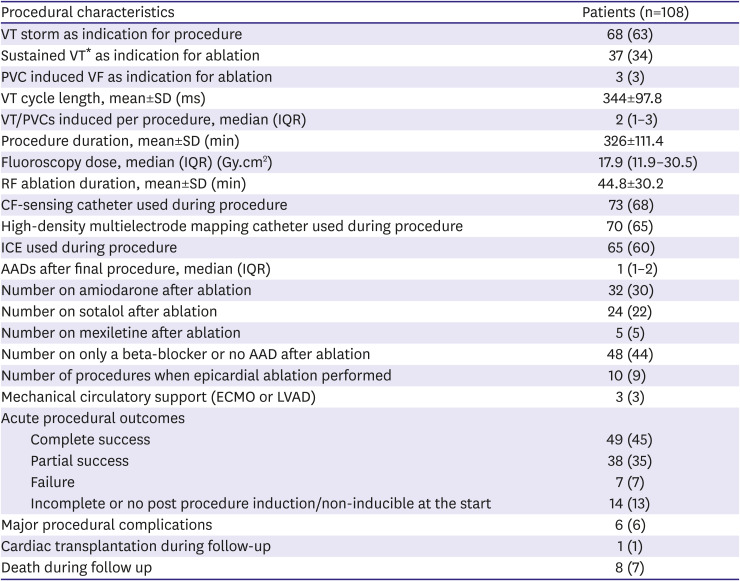
Procedural characteristics |
Patients (n=108) |
VT storm as indication for procedure |
68 (63) |
Sustained VT* as indication for ablation |
37 (34) |
PVC induced VF as indication for ablation |
3 (3) |
VT cycle length, mean±SD (ms) |
344±97.8 |
VT/PVCs induced per procedure, median (IQR) |
2 (1–3) |
Procedure duration, mean±SD (min) |
326±111.4 |
Fluoroscopy dose, median (IQR) (Gy.cm2) |
17.9 (11.9–30.5) |
RF ablation duration, mean±SD (min) |
44.8±30.2 |
CF-sensing catheter used during procedure |
73 (68) |
High-density multielectrode mapping catheter used during procedure |
70 (65) |
ICE used during procedure |
65 (60) |
AADs after final procedure, median (IQR) |
1 (1–2) |
Number on amiodarone after ablation |
32 (30) |
Number on sotalol after ablation |
24 (22) |
Number on mexiletine after ablation |
5 (5) |
Number on only a beta-blocker or no AAD after ablation |
48 (44) |
Number of procedures when epicardial ablation performed |
10 (9) |
Mechanical circulatory support (ECMO or LVAD) |
3 (3) |
Acute procedural outcomes |
|
|
Complete success |
49 (45) |
|
Partial success |
38 (35) |
|
Failure |
7 (7) |
|
Incomplete or no post procedure induction/non-inducible at the start |
14 (13) |
Major procedural complications |
6 (6) |
Cardiac transplantation during follow-up |
1 (1) |
Death during follow up |
8 (7) |
Ventricular arrhythmia burden in follow up
Median number of VA episodes, ICD delivered ATP and shocks per patient were all significantly reduced following ablation (VA episodes before: 10 [IQR: 2–38] vs. VA episodes after: 0 [IQR: 0–2], p<0.001
Figure 1A; ATP before: 16 [IQR: 1.5–57] vs. ATP after: 0 [IQR: 0–2], p<0.001
Figure 1B; ICD shocks before: 1 [IQR: 0–5] vs. shocks after: 0 [IQR: 0–0], p<0.001
Figure 1C). When a 1 month blanking period after ablation was implemented, there was no difference in the burden of VA, ATP or shocks compared to the total 6-month post ablation period (VA with blanking period: 0 [IQR: 0–1] vs. VA no blanking period: 0 [IQR: 0–2], p=0.42; ATP with blanking period: 0 [IQR: 0–0] vs. ATP no blanking period: 0 [IQR: 0–2], p=0.36; Shocks with blanking period: 0 [IQR: 0–0] vs. Shocks no blanking period: 0 [IQR: 0–0], p=0.7).
Figure 1
Burden of VA episodes (A), ATP (B), and ICD shocks (C) per patient during the 6-months before (red), and after (blue) catheter ablation. Each line represents a patient, with the burden of episodes after ablation arranged from highest (at the bottom) to lowest (at the top). The figure demonstrates significant reductions in the number of VA episodes, ATP and shocks in the majority of patients following catheter ablation. The upper values have been capped at 500 in VA and ATP episodes (A, B), and 100 in the number of shocks (C).
ATP = anti-tachycardia pacing; ICD = implantable cardioverter defibrillator; VA = ventricular arrhythmia.
In subgroup analysis of SHD aetiologies, median number of sustained VA episodes and ICD therapies per patient were all significantly reduced in both the ICM (ICM VA episodes before: 14 [IQR: 2–33] vs. ICM VA episodes after: 0 [IQR: 0–2], p<0.001
Figure 2A; ICM ATP before: 17 [IQR: 1–78] vs. ICM ATP after: 0 [IQR: 0–2], p<0.001
Figure 2C; ICM shocks before: 2 [IQR: 0–7] vs. ICM shocks after: 0 [IQR: 0–0], p<0.001
Figure 2E) and NICM (NICM VA episodes before: 8.5 [IQR: 2–38.5] vs. NICM VA episodes after: 0 [IQR: 0–2], p<0.001
Figure 2B; NICM ATP before: 15 [IQR: 3–44.5] vs. NICM ATP after: 0 [IQR: 0–1], p<0.001
Figure 2D; NICM shocks before: 1 [IQR: 0–3] vs. NICM shocks after: 0 [IQR: 0–0], p<0.001
Figure 2F) groups following ablation.
Figure 2
Burden of VA episodes (A), ATP (B), and ICD shocks (C) per patient in ICM and NICM subgroups during the 6-months before (red), and after (blue) catheter ablation in the SHD subgroups ICM and NICM. Each line represents a patient, with the burden of episodes after ablation arranged from highest (at the bottom) to lowest (at the top).
The figure demonstrates significant reductions in the number of VA episodes, ATP and shocks in the majority of patients, in both SHD subgroups following catheter ablation. The upper values have been capped at 500 in the VA and ATP episodes (A-D), and 100 in the number of shocks (E, F).
ATP = anti-tachycardia pacing; ICD = implantable cardioverter defibrillator; ICM = ischemic cardiomyopathy; NICM = non-ischemic cardiomyopathy; SHD = structural heart disease; VA = ventricular arrhythmia.
Following ablation, patients who experienced VA recurrence (n=36, excluding the 1 patient with no ICD interrogation data), still benefitted from a significant reduction in VA burden, ICD delivered ATP and shocks (VA episodes before: 44 [IQR: 10.5–101.5] vs. VA episodes after: 4 [IQR: 2–28.75], p<0.001; ATP before: 49 [IQR: 16–191.5] vs. ATP after: 5 [IQR: 2–13.5], p<0.001; ICD shocks before: 3 [IQR: 0–9] vs. shocks after: 0 [IQR: 0–1], p<0.001).
Reduction in ventricular arrhythmia burden
The proportion of patients who experienced a ≥50%, ≥75%, ≥95%, and ≥99% reduction in VA burden at 6-months after ablation were 90%, 83%, 75%, and 68%, respectively. When using reduction in VA burden as a marker of successful ablation, survival at 6-months with a reduction in VA burden of ≥50%, ≥75%, and ≥95%, were all significantly higher compared to VA-free survival at 6-months (≥50% VA reduction: 90% vs. VA-free survival: 67%, p<0.001; ≥75% VA reduction: 83% vs. VA-free survival: 67%, p=0.001; ≥95% VA reduction: 75% vs. VA-free survival: 67%, p=0.046). Survival at 6-months with a ≥99% reduction in VA burden was comparable to VA-free survival at 6-months (≥99% VA reduction: 68% vs. VA-free survival: 67%, p=0.25).
Acute procedural success and ventricular arrhythmia burden reduction
Acute procedural outcomes, including complications, are shown in
Table 2. Complete success was associated with 86% of patients experiencing ≥75% VA burden reduction compared to 61% VA-free survival at 6-months (p=0.002). In the partial success group, 77% experienced ≥75% VA burden reduction compared to 69% VA-free survival (p=0.2). In those patients with failed procedures, 56% experienced both a ≥75% VA burden reduction and VA-free survival at 6-months (p=0.6).
There were a total of 7 procedural complications, specifically these included: 3 incidences of anticipated atrioventricular block following ablation in the LV basal septum, a coronary sinus perforation and subsequent cardiac tamponade during mapping and ablation of an LV summit VT (required cardiac surgical repair), pericardial bleeding in the immediate post-operative period (managed with a pericardial drain) and 2 retroperitoneal bleeds (one required vascular surgical intervention and the other was managed with medical therapy). There were no intra-procedural deaths during the study.
Limited or no reduction in ventricular arrhythmia burden
Overall, 20 (19%) patients failed to experience ≥75% VA burden reduction following final ablation (
Supplementary Table 1). These patients were more likely to have received a prior catheter ablation for recurrent VA (30% vs. 10%, p=0.02) and there were non-significant trends towards higher incidences of VT storm (75% vs. 61%, p=0.24), diabetes (35% vs. 16%, p=0.06), and underlying atrial arrhythmias (40% vs. 23%, p=0.12). When considering procedural characteristics there were trends towards longer procedures (364.7±127.7 vs. 315.8±106.2 minutes, p=0.08) and longer RF ablation times (54.4±41.5 vs. 41.9±26.6 minutes, p=0.09), with a significantly lower use of CF-sensing catheters (45% vs. 70%, p=0.04) and a higher frequency of procedural failure (20% vs. 6%, p=0.045), compared to the patients experiencing ≥75% VA burden reduction.
There were 11 (10%) patients who experienced <50% reduction in VA burden following ablation (
Supplementary Table 2). These patients had comparable numbers of VA episodes, ATP and shocks before and after ablation (VA before: 13.5 [IQR: 4.8–50.3] vs. VA after: 35 [IQR: 17.3–81.8], p=0.34; ATP before: 36 [IQR: 2–94] vs. ATP after: 41 [IQR: 11.3–52], p=0.7; Shocks before: 1.5 [IQR: 0–3.5] vs. Shocks after: 1 [IQR: 0–4], p=0.69).
Mortality and cardiac transplantation
Overall survival at 6-months follow up was 94%. There was 1 patient who received cardiac transplantation, 79 days after ablation. This patient had a progressive idiopathic NICM with advanced heart failure and had experienced 69 episodes of sustained VT in the 6-months before ablation. His final ablation was acutely successful at rendering all VT non-inducible, but he experienced a recurrence at 30 days after ablation with 1 episode of sustained VT, which required 2 ATP therapies and 1 shock. His overall VA burden was reduced by ≥95% before he underwent cardiac transplantation.
There was no difference in the combined endpoint of freedom from all-cause mortality and cardiac transplantation at 6-months after ablation between patients with ≥50% reduction in VA burden and those with no VA recurrence (94% vs. 94%, p=0.85).
DISCUSSION
This study describes the burden of VA and ICD therapies experienced by patients with SHD related VA, before and after catheter ablation. The study conveys the following important information:
1. The vast majority (>80%) of patients with SHD achieve a large reduction in VA burden (≥75%) following catheter ablation.
2. Overall procedural success at 6-months, was significantly higher when considering ≥75% reduction in VA burden as a metric of efficacy, rather than a singular endpoint of VA-free survival in follow up.
3. Acute non-inducibility of all VT was associated with significantly higher success at 6-months when considering ≥75% reduction in VA burden as a metric of efficacy, rather than a singular VA-free survival.
Traditionally, long-term outcomes following catheter ablation of SHD related VA, have been reported in terms of VA-free survival. Whilst the complete cure of recurrent VA is clearly important to strive towards, the advanced and often progressive nature of many cardiomyopathies, particularly once VA occur, mean that recurrence is common, even when ablation is performed in the most experienced centres.
4)
In the catheter ablation of atrial fibrillation (AF), reduction in arrhythmia burden is well recognised as a reasonable marker of success, with >98% reduction in AF burden achieved, compared to a singular AF recurrence rate of 51–54% at 1-year.
15)
In the treatment of SHD related VA, the Thermocool VT Trial
5) is the largest trial to date to use VA burden as a marker of outcome. Irrigated catheter ablation of ICM related VT was performed in 249 patients, with VA burden recorded in 139 patients who had an ICD (excluding those patients with incessant VT). Of these, 82% benefitted from ≥75% reduction in VT and the proportion of patients with ICD shocks decreased from 81.25% to 26.8%, (p<0.001), over a 6-month period after ablation. In our study we systematically analysed VA burden following catheter ablation of recurrent VA in all patients with SHD, including those with incessant VT. Additionally, we demonstrate the potential for VA burden reduction to be used as a more informative marker of long-term procedural success compared to singular VA-free survival.
Several smaller studies involving novel ablation technologies have used burden as a marker of success. Armaganijan et al.
7) reported the efficaciousness of renal sympathetic denervation in AAD and catheter ablation refractory VA by demonstrating VA burden reduction in 10 high risk patients. Median burden of VA, ATP and shocks were all significantly reduced at 6-months after compared to the 6-months before renal sympathetic denervation; VA episodes: 28.5 (IQR: 1–106) to 0 (IQR: 0–9), ATP: 20.5 (IQR: 0–52) to 0 (IQR: 0–7), shocks: 8 (IQR: 0–88) to 0 (IQR: 0–3). Cuculich et al.
6) reported the first clinical trial using non-invasive stereotactic body radiotherapy in 5 patients with recurrent VA refractory to AADs and catheter ablation. Despite early recurrences (680 during a 6-week blanking period), there was a marked reduction in VA and ICD therapy burden over the preceding 46 patient-months, with a relative reduction of 99.9% from baseline. In our study, we found no difference in post-procedure VA burden whether a 1-month blanking period was implemented or not, potentially making an argument against the use of such a period (in the setting of catheter ablation) and highlighting the importance of treating early high burdens of VA recurrence with prompt repeat ablation.
Overall, 90% of patients in our study experienced ≥50% reduction in VA episodes and 94% of patients experienced a reduction in ICD shocks. With regards to the 2 patients who experienced an increase in shocks following ablation; 1 patient had no ICD in situ before ablation and experienced 1 shock after, whereas the other patient was elderly and deemed too frail to undergo a repeat epicardial procedure after a failed endocardial attempt at ablation of NICM related VT originating from the basal lateral LV and lateral mitral annulus.
There were 20 (19%) patients in whom catheter ablation failed to reduce VA burden by ≥75%. When comparing these patients with those who experienced ≥75% VA burden reduction, they seemingly represented a more arrhythmogenic population, with significantly higher proportions of previously failed ablations and trends towards increased VT storm presentations and higher incidences of underlying atrial arrhythmias. Procedural differences included trends towards increased RF ablation and procedure times, less use of CF-sensing catheters, along with higher numbers of failed procedures, in the <75% VA burden reduction group. It seems plausible to suggest that these patients may have represented a more complex spectrum of SHD pathology, with VT substrate possibly located in intramural locations, potentially reflecting the longer RF ablation times and higher failure rates. The less frequent use of CF-sensing catheters may also have affected sufficient lesion delivery, with higher CF known to correlate with larger lesions.
16)
The clinical implication of our study focuses on the realignment of both patient and healthcare provider expectations. This is particularly relevant when counselling patients regarding catheter ablation for VT. Whilst a singular recurrence is relatively common, the vast majority of patients still benefit from a large reduction in VA episodes and ICD therapies following ablation. Importantly this can be achieved without an increased risk of all-cause mortality or cardiac transplantation, when comparing ≥75% reduction versus no VA recurrence. Failure to report such reductions in VA episodes and ICD therapy following ablation, risks under recognition of catheter ablation for VT as an efficacious procedure, potentially impacting future healthcare funding and limiting its availability for patients who might otherwise benefit from the procedure.
This was a retrospective analysis of patients treated in a single tertiary hospital. There is potential for procedural selection bias and therefore our results may not be applicable to all patient populations. Patients who did not receive an ICD were excluded from this analysis, there is potential for differing results amongst this presumably lower risk cohort. Additionally, accurate collection of VA burden in patients without an ICD is challenging and as such the representation of VA burden as a marker of ablation outcome may be most appropriate for the high risk SHD population. Whilst ICD settings could be standardised in all patients after ablation, the same could not be guaranteed prior to ablation, with some patients referred from other centres, where alternative device settings may have been implemented compared to our centre. Collection of VA burden was limited to 6-months before and after final ablation in order to optimise collection of device interrogation records. It is possible that longer follow up would have altered the mortality and cardiac transplantation results between the ≥50% VA reduction and no VA recurrence groups. In the reporting of VA burden, it is important to appreciate that not all VA episodes are the same, particularly when comparing incessant VT to a shorter sustained VT episode. However, for the purpose of this study the length of episode was not used as a differentiating factor and each individual episode was counted equally. Quality of life data was not collected in our study but would be important additional information for future trials to collect prospectively, with a small number of prior studies demonstrating positive association with reduced VA burden and quality of life.
5)17)
In conclusion, the vast majority of patients (>80%) with SHD achieve a large reduction in VA burden (≥75%) following catheter ablation. Procedural efficacy is significantly higher when considering VA burden reduction as a metric of efficacy, rather than a singular endpoint of VA-free survival in follow up. This data suggests that catheter ablation is highly efficacious for the treatment of VA, and its therapeutic effect is best estimated by using reduction in VA burden as a marker of efficacy.