Abstract
Objective
To estimate the projected cancer risk attributable to diagnostic cone-beam computed tomography (CBCT) performed under different exposure settings for orthodontic purposes in children and adults.
Methods
We collected a list of CBCT machines and their specifications from 38 orthodontists. Organ doses were estimated using median and maximum exposure settings of 105 kVp/156.8 mAs and 130 kVp/200 mAs, respectively. The projected cancer risk attributable to CBCT procedures performed 1–3 times within 2 years was calculated for children (aged 5 and 10 years) and adult (aged 20, 30, and 40 years) male and female patients.
Results
For maximum exposure settings, the mean lifetime fractional ratio (LFR) was 14.28% for children and 0.91% for adults; this indicated that the risk to children was 16 times the risk to adults. For median exposure settings, the mean LFR was 5.25% and 0.58% for children and adults, respectively. The risk of cancer decreased with increasing age. For both median and maximum exposure settings, females showed a higher risk of cancer than did males in all age groups. Cancer risk increased with an increase in the frequency of CBCT procedures within a given period.
Conclusions
The projected dental CBCT-associated cancer risk spans over a wide range depending on the machine parameters and image acquisition settings. Children and female patients are at a higher risk of developing cancer associated with diagnostic CBCT. Therefore, the use of diagnostic CBCT should be justified, and protective measures should be taken to minimize the harmful biological effects of radiation.
Cone-beam computed tomography (CBCT) has emerged as an important adjunctive radiographic technique for orthodontic diagnosis and treatment in the last decade. Limitations of conventional radiographs, such as lateral cephalograms and panoramic radiographs, include image magnification, superimposition of bilateral anatomical structures, and image distortion due to image acquisition mechanisms.1,2 CBCT enables three-dimensional evaluation of the dentofacial structures at a radiation dose lower than that from conventional multi-slice computed tomography (MSCT), although it exhibits comparable dimensional accuracy.3,4 Clinical indications for CBCT include diagnosis and treatment of skeletal malocclusions, impacted teeth, and temporomandibular joint arthritis.5 Moreover, CBCT may aid in the diagnosis of external root resorption and the placement of temporary anchorage devices.5
The improved diagnostic capabilities of CBCT provide considerable benefits to patients. However, compared with conventional radiography, CBCT exposes patients to increased doses of ionizing radiation.6 Previous studies on CBCT dosimetry have shown that the mean organ dose (84–212 μSv)7 is significantly higher than that delivered for the acquisition of lateral cephalograms and panoramic radiographs.6 It has been reported that the organ dose depends on the manufacturer and image acquisition settings such as tube current (mA), peak tube voltage (kVp), field of view (FOV), and voxel size.7-9
Carcinogenesis is a significant biological effect of ionizing radiation. Studies have reported a significant association of pediatric computed tomography (CT) with leukemia and brain tumors.10,11 In South Korea, patients aged < 19 years who have been exposed to ionizing radiation for diagnostic purposes showed a significantly higher incidence of lymphoid, hematopoietic, and solid cancers.12 The increased use of CBCT in orthodontics has raised health concerns, especially for children and adolescents, who comprise the majority of the orthodontic patient population. However, there are limited studies on the biological effects of ionizing radiation from diagnostic CBCT. Previous studies have mostly focused on the organ dose, and the consequences associated with radiation exposure, such as cancer, have not been fully explored.6-9
Therefore, the aim of this study was to estimate the projected cancer risk attributable to diagnostic craniofacial CBCT performed for orthodontic purposes in children and adults, based on the organ doses calculated from the CBCT machine specifications. In addition, we aimed to compare the risk of cancer according to age, sex, and exposure settings such as the radiation dose and the number of exposures.
To estimate the organ doses delivered by diagnostic CBCT machines used in dental clinics and hospitals in South Korea, we collected a list of CBCT machines and their specifications by sending questionnaires to 72 orthodontists who belonged to the Korean Society of Digital Orthodontists. Thirty-eight orthodontists responded; thus, the response rate was 53%. Descriptive statistics for the exposure parameters of the CBCT machines, such as kVp, mA, and exposure time (s), are shown in Table 1. According to the collected data pertaining to CBCT machine parameters, the median exposure settings were 105 kVp/156.8 mAs while the maximum exposure settings were 130 kVp/200 mAs.
Organ doses under the median and maximum exposure settings were calculated for adult male and female patients using ALARA-Dental software (Kyung Hee University, Seoul, Korea; Korea Centers for Disease Control and Prevention, Cheongju, Korea; Figure 1).13 ALARA-Dental is a software that provides estimated organ doses and effective doses delivered for the acquisition of dental radiographs, including intraoral radiographs, panoramic radiographs, and CBCT images. It uses the Monte Carlo (MC) method, a computer simulation technique for modeling the transport of particles based on the probability distribution for radiation interaction with matter. The MC method is commonly used for radiation dosimetry calculations.14,15 Among the various codes for the MC method, ALARA-Dental used the Monte Carlo N Particle code, known as the MCNP code and developed at the Los Alamos National Laboratory (New Mexico, United States).16 The MCNP code is the most commonly used code for MC simulation in the medical field, and it has shown reliability in the estimation of organ doses for CT examinations.16,17 Using ALARA-Dental software, the organ dose was estimated using parameters associated with radiation exposure, such as kVp, mAs, the FOV, the filter thickness, the degree of revolution of the X-ray source, and the beam geometry (full beam versus half beam). The following variables were set as fixed values for both median and maximum exposure settings: filter thickness, 2 mm; FOV, 19 × 20 cm; full beam projection; and beam revolution, 360°.
Clinical scenarios were hypothesized for orthodontic patients undergoing CBCT. The treatment period for patients was assumed to be 2 years. Cancer risks were estimated for male and female patients aged 5, 10, 20, 30, and 40 years. In addition, the risk according to the frequency of CBCT procedures (once, twice, or thrice) during a 2-year period (2018–2020) was estimated.
The organ doses for median and maximum exposure settings calculated by the ALARA-Dental software were used to estimate the excess lifetime risk of cancers using the radiation risk assessment tool (RadRAT, version 4.1.1; National Institutes of Health, Bethesda, MD, USA, Figure 2).18 RadRAT is an open-source software for estimating the lifetime cancer risk attributable to radiation exposure based on the Biological Effects of Ionizing Radiation (BEIR) VII report rolled out by the National Research Council of the United States.19
For each exposure scenario, the mean lifetime attributable risk (LAR), which is the probability of premature incidence of cancer attributable to radiation exposure in a representative member of the population (i.e., the probability that an exposed population will develop radiation-induced cancer during their lifetime), was estimated as excess cases (n) per 100,000. The lifetime baseline risk (LBR) for cancer, which is the cumulative baseline probability of developing cancer during an individual’s lifetime that is not associated with the additional radiation from CBCT (n), was calculated based on the cancer incidence rates in South Korea in 2010. The Lifetime fractional ratio (LFR), which is the ratio of LAR to LBR (%), was presented to express the attributable risk relative to the baseline risk. The LFR is more reliable than the LAR because it considers differences in the population structure and cancer incidence rates among different populations.20
The organs and tissues for which specific radiation doses were estimated using ALARA-Dental software included the brain, salivary gland, oral mucosa, esophagus, thyroid, skin, thymus, airway, skeletal muscles, bone, red bone marrow, and remaining tissues. In the RadRAT software, organ doses were entered for the years 2018–2020. Date, sex, and year of birth were entered to represent patients aged 5, 10, 20, 30, and 40 years. Sex- and age-specific incidence rates in South Korea in 2010 were used for determining the baseline incidence rates, and survival function was based on the Korean population in 2010.
As a result, the LAR for site-specific cancers, including those of the oral cavity and pharynx, esophagus, lung, nervous system, thyroid, bone marrow, and other ill-defined sites, were estimated. The LAR of all cancers combined was calculated as the sum of all risks to individual organs. The excess cancer risk was calculated with 90% uncertainty intervals to incorporate both statistical and subjective uncertainties computed by MC simulations using the RadRAT software. Risk calculation was performed with 300 MC iterations and a random number of seed equal to 99.
The estimated organ doses absorbed and the distribution among different organs are shown in Table 2 and Figure 3. For adults, the highest organ dose was observed for the salivary glands, followed by the oral mucosa, brain, thyroid gland, and skin. For children, the highest organ dose was observed for the salivary glands, followed by the oral mucosa, brain, skin, and thyroid gland.
The mean LAR and LFR according to age, sex, and the frequency of exposures (number of CBCT procedures) under median and maximum exposure settings are shown in Tables 3 and 4, respectively. Children (5 and 10 years old) demonstrated a higher cancer risk than did adults (20, 30, and 40 years old). For median exposure settings, the LFRs were 1.1–16.4% for children and 0.1–2.0% for adults. For maximum exposure settings, the LFRs were 2.4–24.6% for children and 0.3–2.5% for adults. The risk of cancer decreased with increasing age, as depicted in Figure 4 based on the LFRs for each age group. The LFR also increased with an increase in the number of exposures. For example, at maximum exposure settings for a 20-year-old female, the LFR increased from 1.68% for one exposure to 1.71% and 2.54% for two and three exposures, respectively (Table 4). With regard to sex, the LFR for females was higher than that for men at all exposure settings (Figure 5).
The cancer risk estimation for each site showed that both adults and children had the highest risk of developing thyroid cancer due to radiation. However, the risk of thyroid cancer was approximately 3.5 times higher for children than for adults (Figure 6).
With advances in medical imaging technology, radiation exposure due to medical diagnostic imaging has doubled over the past two decades.9 Journy et al.21 conducted a nationwide study and reported that even a small increase in the number of annual CT examinations for patients aged < 20 years would significantly increase the number of new cancer cases. In addition, an epidemiological study on the cancer risk due to chronic occupational exposure to low-dose radiation among medical radiation workers, doctors, and dentists have shown a significant increase in the prevalence of solid cancers and leukemia.22 The occupational risk from low-dose radiation has been studied using dosimetry data collected from radiation workers and their medical records. However, dosimetry data from diagnostic medical imaging procedures performed for patients cannot be obtained directly to determine the effects of radiation exposure on health. Therefore, dosimetry data can be estimated by using the mean organ doses from imaging devices and applying them to risk projection models developed from a life-span study of atomic bomb survivors and nuclear power plant accidents.23,24 In the present study, we collected a list of CBCT machines and their specifications from 38 orthodontists and evaluated the organ doses using the median and maximum kVp and mAs values for the machines. Although we have calculated the cancer risk using minimum exposure parameters, we concluded that these parameters may not represent the average clinical values used in orthodontic clinics. In addition, these parameters might not yield a clinically valid image. Therefore, the LFR for minimum exposure settings was low (Supplementary Table 1) and consequently excluded from the results.
Our results showed that radiation from CBCT may induce a high risk of thyroid, oral, and pharyngeal cancers in both adults and children (Figure 6). With regard to the effects of age and sex, we found that female and young patients developed a higher cancer risk than did male and adult patients. Our findings are consistent with those of previous reports.25-28 Children exhibit high radiosensitivity due to the increased turnover rate of cells. The prevalence of thyroid cancer has been shown to be higher in females than in males,29 with females being more susceptible to thyroid cancer because of additive exposure to radiation. Other factors such as the position of the X-ray beam and the FOV may also be associated with the risk of cancer. Pauwels et al.25 compared the absorbed organ doses based on skin dosimetry and concluded that the FOV should be placed as high as possible to avoid direct exposure to the thyroid, a vital organ affecting the total cancer risk from radiation exposure. The oral mucosa is also directly exposed to ionizing radiation during a maxillofacial CBCT scan. Yeh and Chen26 showed that organ doses vary depending on the vertical position of the central beam. When the central beam was positioned at the level of the teeth, the salivary glands received the highest organ dose. This observation is in line with our results, which showed that the oral cavity and pharynx received the highest organ doses. Stratis et al.27 also reported the highest organ dose for the salivary glands and oral mucosa in pediatric patients undergoing CBCT.
The projected risk of cancer was calculated according to the linear no-threshold risk (LNT) hypothesis, which states that any radiation dose greater than zero will result in a proportionate increase in the risk of cancer. Although there are controversies regarding radiation risk estimation, the LNT model is currently the most widely accepted model for explaining the radiation dose–response relationship based on current epidemiological data.30
Thus, the cancer risk from diagnostic CBCT imaging is affected by age, sex, machine parameters, and the number of exposures. Our results indicate an increased risk of cancer due to CBCT performed for orthodontic purposes, with the risk being higher for children and females. Therefore, strict adherence to the as low as reasonably achievable (ALARA) principle must be maintained. The International Commission on Radiological Protection addresses the three principles of medical radiation exposure as follows: (1) medical radiation exposure should be justified and have a defined purpose for an individual patient, (2) the patient dose should be carefully managed according to the ALARA principle, and (3) protective measures should be used to avoid unnecessary exposure. Therefore, routine CBCT imaging for the purpose of orthodontic treatment cannot be justified, and the smallest possible FOV to observe the region of interest should be selected. Furthermore, performing CBCT instead of MSCT would decrease the effective dose by approximately 1/7 to 1/10.3
This study has some limitations. First, the estimated cancer risks were based on mean organ doses estimated according to the exposure settings and the age and sex of patients, not on individual exposure settings. Second, confounding factors related to lifestyle (smoking and alcohol intake), environment, and genetics were not considered. For personalized assessment of cancer risk, a study incorporating the effects of these factors is warranted. Third, our organ doses were calculated from machine specifications in South Korea, and there could be other CBCT machines using higher radiation doses.
Supplementary data is available at https://doi.org/10.4041/kjod.2021.51.3.189.
In conclusion, the projected cancer risk attributable to dental CBCT is estimated to span over a wide range depending on demographic factors, machine parameters, and image acquisition settings. Females and children show a higher risk of developing cancer due to radiation from CBCT. Clinicians should be aware of the increased cancer risk due to medical exposure to ionizing radiation, and efforts should be made to minimize the dose from CBCT through optimization of machine parameters such as the FOV and voxel size for the region of interest.
ACKNOWLEDGEMENTS
We would like to thank the members of the Korean Society of Digital Orthodontists for their support with the questionnaires for this study.
REFERENCES
1. Adams GL, Gansky SA, Miller AJ, Harrell WE Jr, Hatcher DC. 2004; Comparison between traditional 2-dimensional cephalometry and a 3-dimensional approach on human dry skulls. Am J Orthod Dentofacial Orthop. 126:397–409. DOI: 10.1016/j.ajodo.2004.03.023. PMID: 15470343.


2. Tronje G, Welander U, McDavid WD, Morris CR. 1981; Image distortion in rotational panoramic radiography. I. General considerations. Acta Radiol Diagn (Stockh). 22(3A):295–9. DOI: 10.1177/028418518102203A14. PMID: 7315508.
3. Loubele M, Bogaerts R, Van Dijck E, Pauwels R, Vanheusden S, Suetens P, et al. 2009; Comparison between effective radiation dose of CBCT and MSCT scanners for dentomaxillofacial applications. Eur J Radiol. 71:461–8. DOI: 10.1016/j.ejrad.2008.06.002. PMID: 18639404.


4. Suomalainen A, Vehmas T, Kortesniemi M, Robinson S, Peltola J. 2008; Accuracy of linear measurements using dental cone beam and conventional multislice computed tomography. Dentomaxillofac Radiol. 37:10–7. DOI: 10.1259/dmfr/14140281. PMID: 18195249.


5. Kapila SD, Nervina JM. 2015; CBCT in orthodontics: assessment of treatment outcomes and indications for its use. Dentomaxillofac Radiol. 44:20140282. DOI: 10.1259/dmfr.20140282. PMID: 25358833. PMCID: PMC4277443.


6. Wrzesień M, Olszewski J. 2017; Absorbed doses for patients undergoing panoramic radiography, cephalometric radiography and CBCT. Int J Occup Med Environ Health. 30:705–13. DOI: 10.13075/ijomeh.1896.00960. PMID: 28584324.


7. Ludlow JB, Timothy R, Walker C, Hunter R, Benavides E, Samuelson DB, et al. 2015; Effective dose of dental CBCT-a meta analysis of published data and additional data for nine CBCT units. Dentomaxillofac Radiol. 44:20140197. DOI: 10.1259/dmfr.20140197. PMID: 25224586. PMCID: PMC4277438.


8. Ludlow JB, Ivanovic M. 2008; Comparative dosimetry of dental CBCT devices and 64-slice CT for oral and maxillofacial radiology. Oral Surg Oral Med Oral Pathol Oral Radiol Endod. 106:106–14. DOI: 10.1016/j.tripleo.2008.03.018. PMID: 18504152.


9. Sezgin ÖS, Kayipmaz S, Yasar D, Yilmaz AB, Ozturk MH. 2012; Comparative dosimetry of dental cone beam computed tomography, panoramic radiography, and multislice computed tomography. Oral Radiol. 28:32–7. DOI: 10.1007/s11282-011-0078-5.


10. United Nations. 2013. UNSCEAR 2013 Report to the general assembly, with scientific annexes. Effect of ionizing radiations. United Nations Publications;New York:
11. Pearce MS, Salotti JA, Little MP, McHugh K, Lee C, Kim KP, et al. 2012; Radiation exposure from CT scans in childhood and subsequent risk of leukaemia and brain tumours: a retrospective cohort study. Lancet. 380:499–505. DOI: 10.1016/S0140-6736(12)60815-0. PMID: 22681860. PMCID: PMC3418594.



12. Hong JY, Han K, Jung JH, Kim JS. 2019; Association of exposure to diagnostic low-dose ionizing radiation with risk of cancer among youths in South Korea. JAMA Netw Open. 2:e1910584. DOI: 10.1001/jamanetworkopen.2019.10584. PMID: 31483470. PMCID: PMC6727680.


13. Kim HJ, Lee JY, Lee HK, Kim KP. 2020. Public health weekly report. Computer programs (ALARA) for calculation of diagnostic radiation dose. Korea Disease Control and Prevention Agency;Cheongju: p. 1023–6.
14. Lee C, Kim KP, Long DJ, Bolch WE. 2012; Organ doses for reference pediatric and adolescent patients undergoing computed tomography estimated by Monte Carlo simulation. Med Phys. 39:2129–46. DOI: 10.1118/1.3693052. PMID: 22482634. PMCID: PMC3326072.



15. Servomaa A, Tapiovaara M. 1998; Organ dose calculation in medical X-ray examinations by the program PCXMC. Radiat Protect Dosim. 80:213–9. DOI: 10.1093/oxfordjournals.rpd.a032509.
16. Solberg TD, DeMarco JJ, Chetty IJ, Mesa AV, Cagnon CH, Li AN, et al. 2001; A review of radiation dosimetry applications using the MCNP Monte Carlo code. Radiochim Acta. 89:337–55. DOI: 10.1524/ract.2001.89.4-5.337.


17. Long DJ, Lee C, Tien C, Fisher R, Hoerner MR, Hintenlang D, et al. 2013; Monte Carlo simulations of adult and pediatric computed tomography exams: validation studies of organ doses with physical phantoms. Med Phys. 40:013901. DOI: 10.1118/1.4771934. PMID: 23298124. PMCID: PMC3537704.


18. Berrington de Gonzalez A, Iulian Apostoaei A, Veiga LH, Rajaraman P, Thomas BA, Owen Hoffman F, et al. 2012; RadRAT: a radiation risk assessment tool for lifetime cancer risk projection. J Radiol Prot. 32:205–22. DOI: 10.1088/0952-4746/32/3/205. PMID: 22810503. PMCID: PMC3816370.

19. National Research Council. 2006. Health risks from exposure to low levels of ionizing radiation: BEIR VII phase 2. National Academies Press;Washington, D.C.:
20. Kellerer AM, Nekolla EA, Walsh L. 2001; On the conversion of solid cancer excess relative risk into lifetime attributable risk. Radiat Environ Biophys. 40:249–57. DOI: 10.1007/s004110100106. PMID: 11820733.


21. Journy NM, Lee C, Harbron RW, McHugh K, Pearce MS, Berrington de González A. 2017; Projected cancer risks potentially related to past, current, and future practices in paediatric CT in the United Kingdom, 1990-2020. Br J Cancer. 116:109–16. DOI: 10.1038/bjc.2016.351. PMID: 27824812. PMCID: PMC5220140.


22. Lee WJ, Choi Y, Ko S, Cha ES, Kim J, Kim YM, et al. 2018; Projected lifetime cancer risks from occupational radiation exposure among diagnostic medical radiation workers in South Korea. BMC Cancer. 18:1206. DOI: 10.1186/s12885-018-5107-x. PMID: 30514249. PMCID: PMC6278159.



23. Walsh L, Zhang W, Shore RE, Auvinen A, Laurier D, Wakeford R, et al. 2014; A framework for estimating radiation-related cancer risks in Japan from the 2011 Fukushima nuclear accident. Radiat Res. 182:556–72. DOI: 10.1667/RR13779.1. PMID: 25251702.


24. Douple EB, Mabuchi K, Cullings HM, Preston DL, Kodama K, Shimizu Y, et al. 2011; Long-term radiation-related health effects in a unique human population: lessons learned from the atomic bomb survivors of Hiroshima and Nagasaki. Disaster Med Public Health Prep. 5 Suppl 1:S122–33. DOI: 10.1001/dmp.2011.21. PMID: 21402804. PMCID: PMC3907953.


25. Pauwels R, Cockmartin L, Ivanauskaité D, Urbonienė A, Gavala S, Donta C, et al. 2014; Estimating cancer risk from dental cone-beam CT exposures based on skin dosimetry. Phys Med Biol. 59:3877–91. DOI: 10.1088/0031-9155/59/14/3877. PMID: 24957710.


26. Yeh JK, Chen CH. 2018; Estimated radiation risk of cancer from dental cone-beam computed tomography imaging in orthodontics patients. BMC Oral Health. 18:131. DOI: 10.1186/s12903-018-0592-5. PMID: 30075771. PMCID: PMC6091080.



27. Stratis A, Zhang G, Jacobs R, Bogaerts R, Bosmans H. 2019; The growing concern of radiation dose in paediatric dental and maxillofacial CBCT: an easy guide for daily practice. Eur Radiol. 29:7009–18. DOI: 10.1007/s00330-019-06287-5. PMID: 31264018.


28. Wu TH, Lin WC, Chen WK, Chang YC, Hwang JJ. 2015; Predicting cancer risks from dental computed tomography. J Dent Res. 94:27–35. DOI: 10.1177/0022034514554226. PMID: 25359782.


29. Peterson E, De P, Nuttall R. 2012; BMI, diet and female reproductive factors as risks for thyroid cancer: a systematic review. PLoS One. 7:e29177. DOI: 10.1371/journal.pone.0029177. PMID: 22276106. PMCID: PMC3261873.


30. Shore RE, Beck HL, Boice JD, Caffrey EA, Davis S, Grogan HA, et al. 2018; Implications of recent epidemiologic studies for the linear nonthreshold model and radiation protection. J Radiol Prot. 38:1217–33. DOI: 10.1088/1361-6498/aad348. PMID: 30004025.


Figure 1
ALARA-Dental software (Kyung Hee University, Seoul, Korea, and Korea Centers for Disease Control and Prevention, Cheongju, Korea)13 for estimating organ doses delivered for the acquisition of dental radiographs, including intraoral radiographs, panoramic radiographs, and cone-beam computed tomography images.
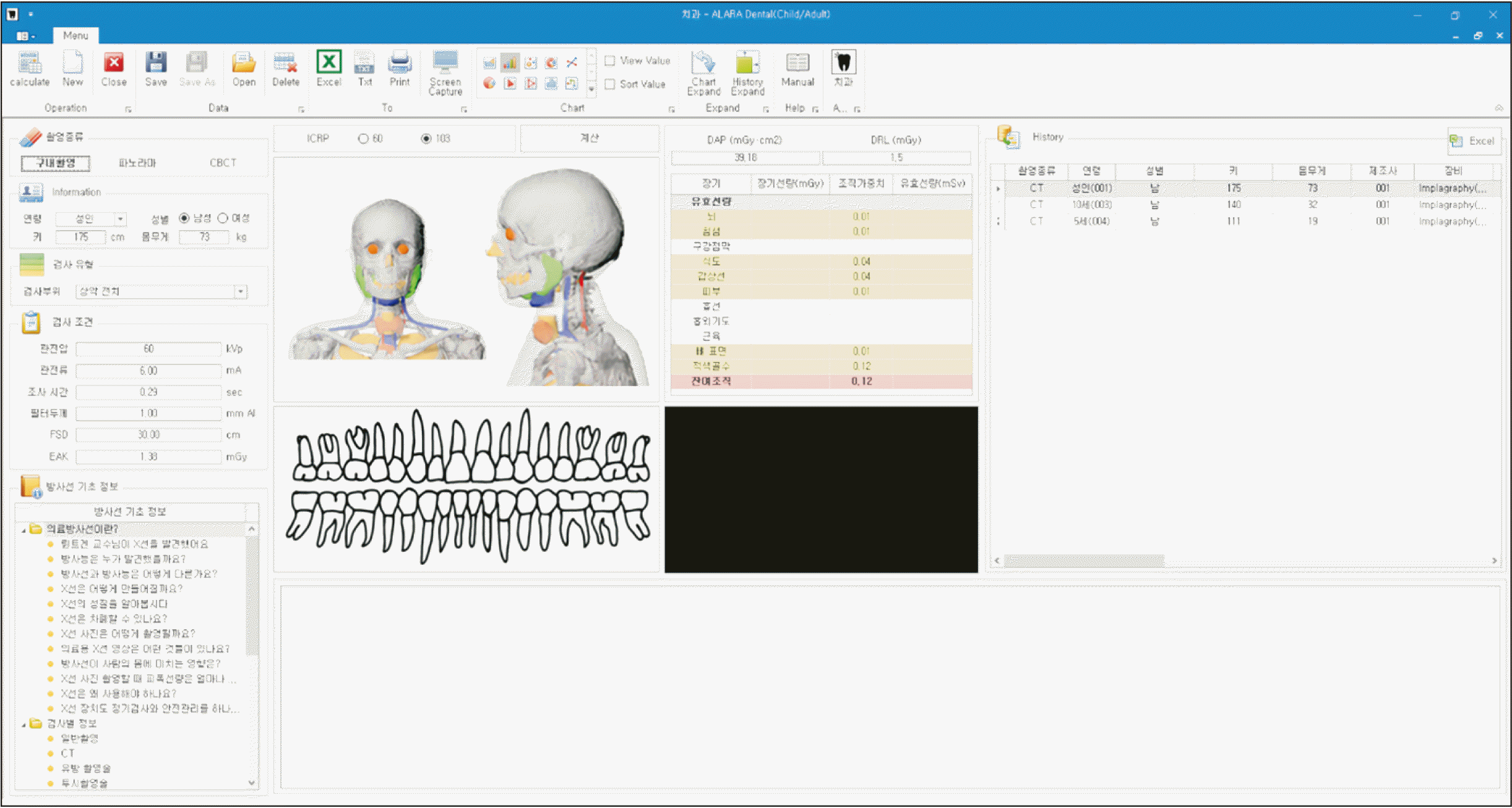
Figure 2
Estimation of cancer risk from cone-beam computed tomography for orthodontic purposes using the radiation risk assessment tool (RadRAT version 4.1.1; National Institutes of Health, Bethesda, MD, USA).18
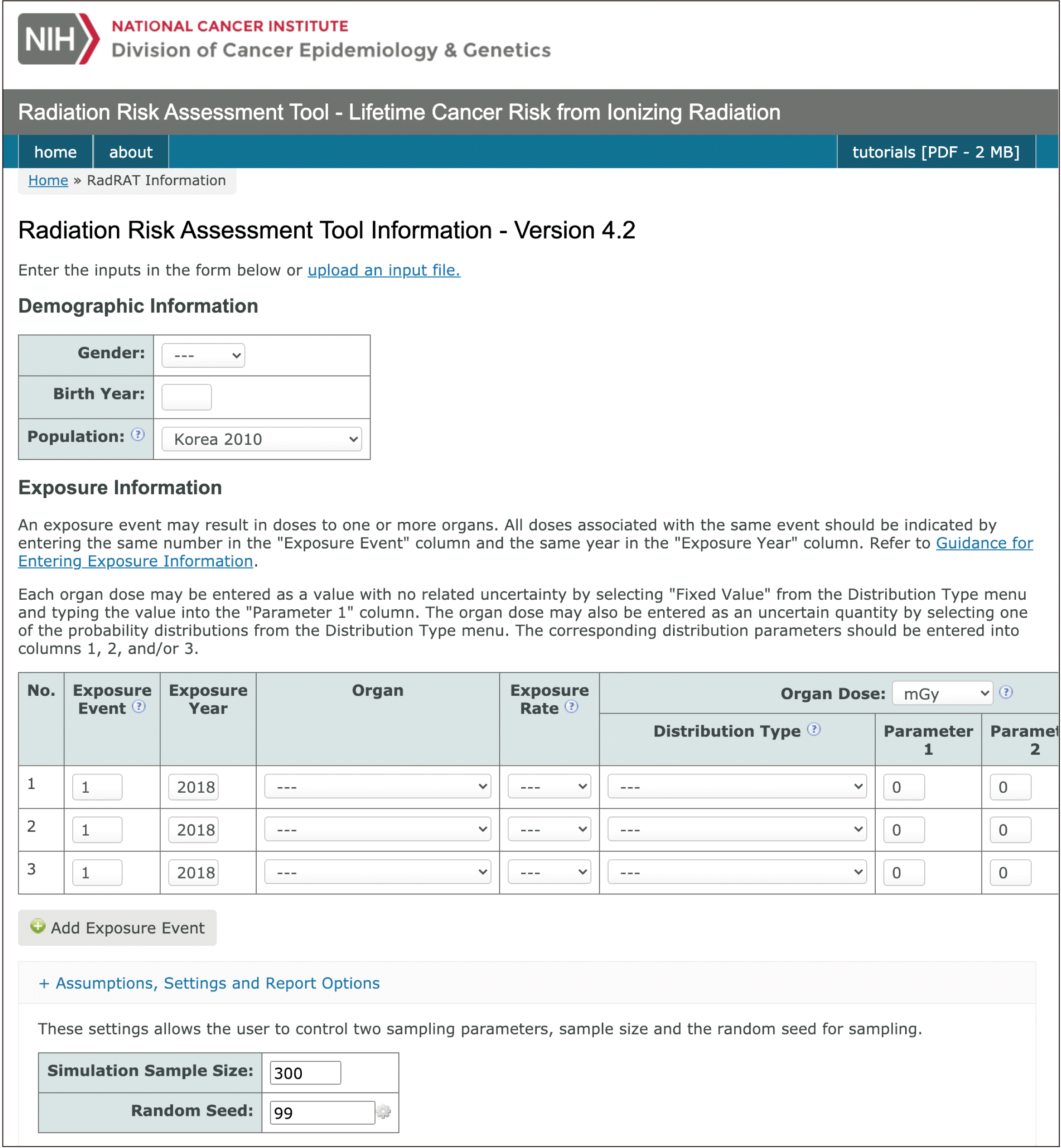
Figure 3
Distribution of mean organ doses (mGy) delivered by cone-beam computed tomography performed for orthodontic purposes in children and adults.
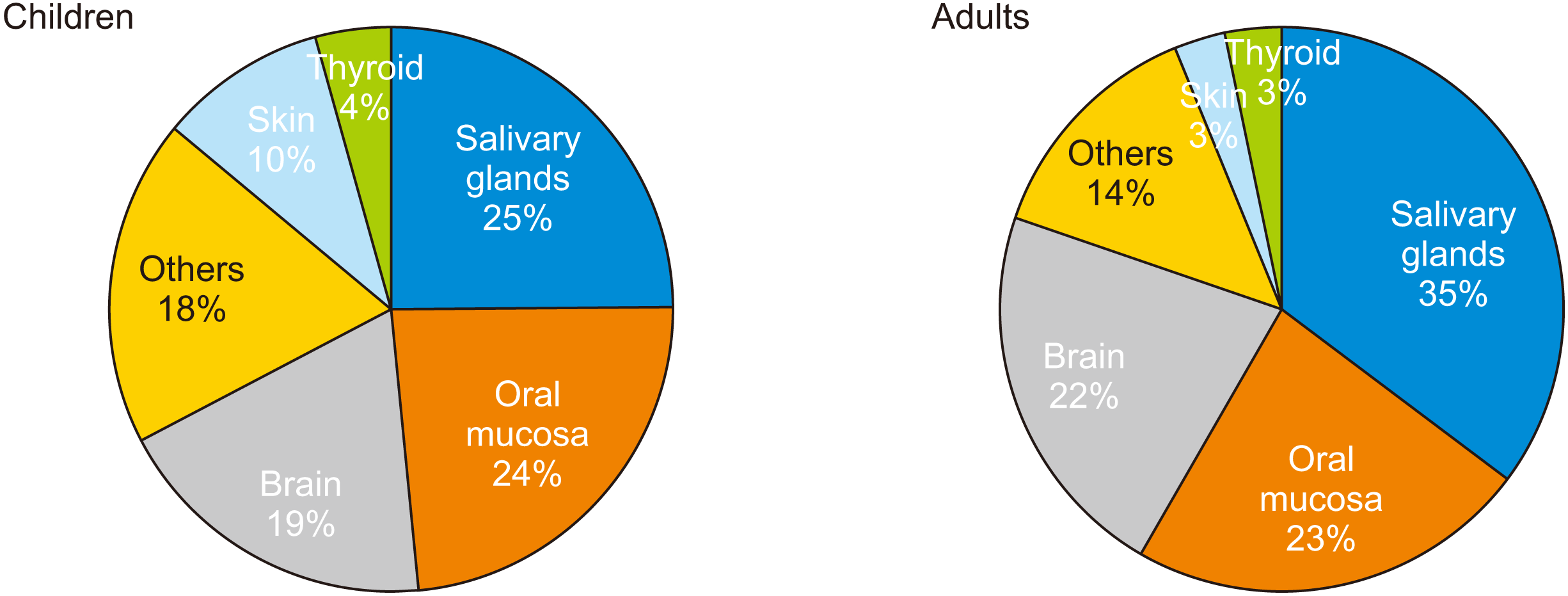
Figure 4
Mean lifetime fractional ratio (LFR) after a single exposure under maximum and median exposure settings, according to age.
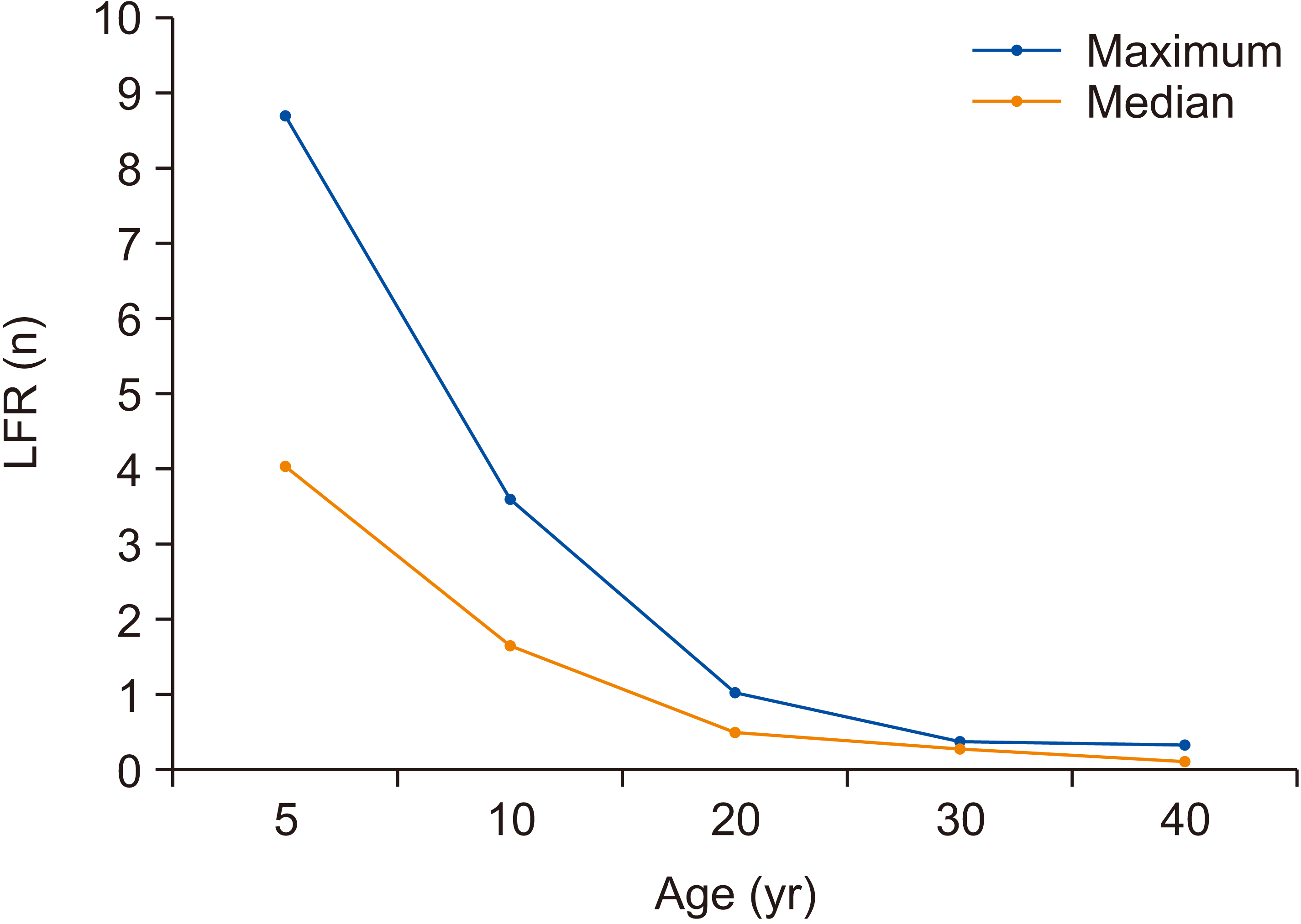
Figure 5
Comparison of the mean lifetime fractional ratio (LFR) after a single exposure under maximum exposure settings between male and female patients in different age groups.
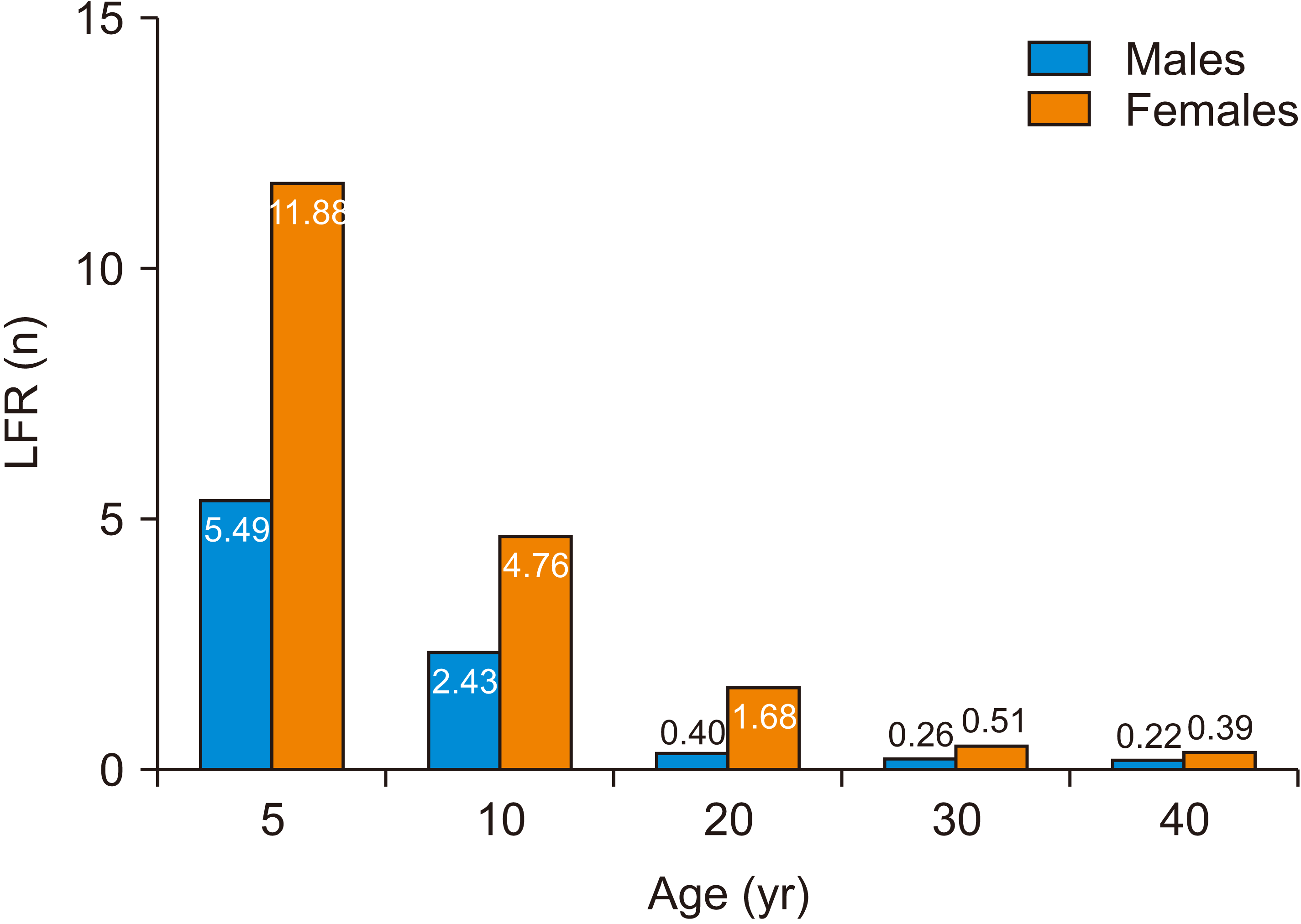
Figure 6
Comparison of mean lifetime attributable risk (LAR) values after a single exposure under maximum exposure parameters between adults and children, according to the cancer site.
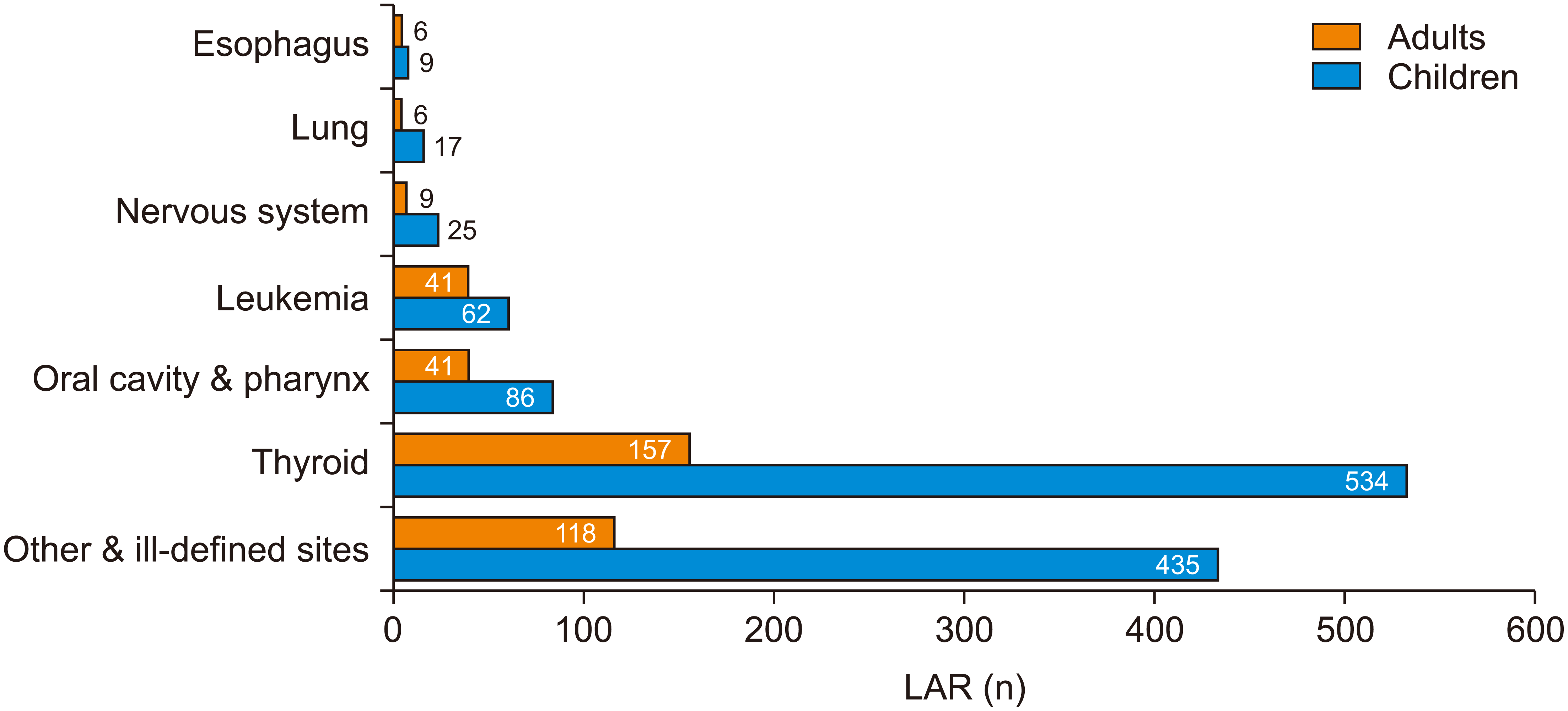
Table 1
Descriptive statistics for the exposure parameters of cone-beam computed tomography machines analyzed in the study
Statistics | kVp | mA | Exposure time (s) |
---|---|---|---|
Mean ± standard deviation | 94.0 ± 18.3 | 7.7 ± 2.6 | 20.2 ± 5.3 |
Range | 80–130 | 5–16 | 9–24 |
Median | 105 | 9.5 | 16.5 |
Table 2
Estimated mean organ doses (mGy) under median and maximum exposure settings for cone-beam computed tomography performed for orthodontic purposes in children aged 5 and 10 years and adults
Exposed organ | Median exposure* | Maximum exposure† | |||||||
---|---|---|---|---|---|---|---|---|---|
|
|
||||||||
Children‡ |
Adults (M) |
Adults (F) |
Children‡ |
Adults (M) |
Adults (F) |
||||
|
|
||||||||
5 years | 10 years | 5 years | 10 years | ||||||
Brain | 33.3 | 28.4 | 18.7 | 26.5 | 69.4 | 59.5 | 23.0 | 32.2 | |
Salivary glands | 40.9 | 42.2 | 39.6 | 39.0 | 82.1 | 84.0 | 41.3 | 40.2 | |
Oral mucosa | 43.4 | 33.3 | 23.3 | 25.0 | 89.9 | 70.7 | 28.2 | 29.5 | |
Esophagus | 4.5 | 1.7 | 0.4 | 0.6 | 9.3 | 3.6 | 0.5 | 0.7 | |
Thyroid | 9.2 | 5.2 | 3.1 | 3.2 | 18.5 | 10.8 | 4.2 | 3.4 | |
Skin | 21.1 | 12.1 | 3.1 | 3.8 | 40.3 | 23.1 | 3.0 | 3.7 | |
Thymus | 1.8 | 1.0 | 0.4 | 0.9 | 4.1 | 2.2 | 0.7 | 1.0 | |
Thoracic airway | 3.8 | 2.0 | 0.9 | 1.1 | 7.7 | 4.1 | 1.2 | 1.4 | |
Skeletal muscle | 4.1 | 2.0 | 1.1 | 2.2 | 8.1 | 4.1 | 1.2 | 2.3 | |
Bone surface | 11.6 | 5.1 | 1.6 | 1.6 | 24.6 | 10.9 | 4.2 | 9.1 | |
Red bone marrow | 10.5 | 5.4 | 3.3 | 4.3 | 22.3 | 11.6 | 2.0 | 10.1 | |
Remainder organ & tissues | 2.2 | 2.9 | 2.0 | 2.2 | 8.5 | 6.2 | 2.4 | 2.6 | |
Total | 186.6 | 141.5 | 97.3 | 110.5 | 384.8 | 290.9 | 111.9 | 136.4 |
Table 3
Age (yr) |
No. of exp |
Sex | LAR (n) |
LBR (n) |
LFR (LAR‡/LBR, %) |
|||||||
---|---|---|---|---|---|---|---|---|---|---|---|---|
|
||||||||||||
Oral cavity & pharynx | Esophagus | Lung | Nervous system | Thyroid | Other & ill-defined sites | Leukemia | All cancers (total) | |||||
5 | 1 | F | 44 | 1 | 13 | 7 | 690 | 260 | 15 | 1,030 | 18,430 | 5.59 |
5 | 1 | M | 50 | 4 | 7 | 24 | 72 | 307 | 20 | 482 | 19,313 | 2.50 |
5 | 2 | F | 86 | 1 | 26 | 14 | 1,330 | 512 | 29 | 1,990 | 18,430 | 10.80 |
5 | 2 | M | 97 | 97 | 8 | 14 | 48 | 138 | 605 | 946 | 19,313 | 4.90 |
5 | 3 | F | 130 | 2 | 39 | 21 | 2,020 | 772 | 44 | 3,020 | 18,430 | 16.39 |
5 | 3 | M | 147 | 147 | 12 | 20 | 72 | 210 | 912 | 1,430 | 19,310 | 7.41 |
10 | 1 | F | 32 | 0 | 6 | 4 | 257 | 106 | 5 | 408 | 18,394 | 2.22 |
10 | 1 | M | 36 | 1 | 3 | 16 | 27 | 124 | 7 | 210 | 19,270 | 1.09 |
10 | 2 | F | 62 | 0 | 12 | 8 | 493 | 208 | 10 | 791 | 18,394 | 4.30 |
10 | 2 | M | 70 | 2 | 6 | 30 | 51 | 243 | 14 | 411 | 19,270 | 2.13 |
10 | 3 | F | 94 | 1 | 17 | 13 | 750 | 314 | 15 | 1,200 | 18,390 | 6.53 |
10 | 3 | M | 106 | 4 | 9 | 45 | 78 | 368 | 21 | 621 | 19,270 | 3.22 |
20 | 1 | F | 18 | 0 | 4 | 2 | 68 | 30 | 3 | 123 | 18,273 | 0.67 |
20 | 1 | M | 19 | 0 | 1 | 5 | 7 | 26 | 3 | 60 | 19,173 | 0.31 |
20 | 2 | F | 34 | 0 | 7 | 4 | 130 | 59 | 5 | 239 | 18,273 | 1.31 |
20 | 2 | M | 38 | 0 | 2 | 9 | 13 | 52 | 5 | 118 | 19,173 | 0.62 |
20 | 3 | F | 52 | 0 | 11 | 7 | 198 | 88 | 8 | 362 | 18,273 | 1.98 |
20 | 3 | M | 57 | 1 | 3 | 14 | 20 | 78 | 8 | 178 | 19,173 | 0.93 |
30 | 1 | F | 12 | 0 | 3 | 1 | 27 | 21 | 2 | 65 | 17,619 | 0.37 |
30 | 1 | M | 13 | 0 | 1 | 3 | 3 | 18 | 2 | 39 | 19,035 | 0.21 |
30 | 2 | F | 23 | 0 | 5 | 3 | 51 | 40 | 4 | 126 | 17,619 | 0.72 |
30 | 2 | M | 26 | 0 | 1 | 6 | 5 | 35 | 5 | 77 | 19,035 | 0.41 |
30 | 3 | F | 34 | 0 | 8 | 4 | 78 | 61 | 7 | 191 | 17,619 | 1.08 |
30 | 3 | M | 39 | 0 | 2 | 9 | 8 | 54 | 7 | 117 | 19,035 | 0.61 |
40 | 1 | F | 3 | < 0 | 1 | 0 | 2 | 3 | 1 | 18 | 15,977 | 0.11 |
40 | 1 | M | 1 | 0 | 0 | 1 | 0 | 5 | 1 | 16 | 18,670 | 0.09 |
40 | 2 | F | 5 | < 0 | 2 | 1 | 4 | 6 | 2 | 35 | 15,977 | 0.22 |
40 | 2 | M | 3 | 0 | 0 | 1 | 0 | 10 | 2 | 33 | 18,670 | 0.18 |
40 | 3 | F | 8 | < 0 | 4 | 1 | 6 | 8 | 2 | 52 | 15,977 | 0.33 |
40 | 3 | M | 4 | 0 | 1 | 2 | 1 | 15 | 2 | 49 | 18,670 | 0.26 |
Table 4
Age (yr) |
No. of exp | Sex | LAR (n) |
LBR (n) |
LFR (LAR‡/LBR, %) |
|||||||
---|---|---|---|---|---|---|---|---|---|---|---|---|
|
||||||||||||
Oral cavity & pharynx | Esophagus | Lung | Nervous system | Thyroid | Other & ill-defined sites | Leukemia | All cancers (total) | |||||
5 | 1 | F | 92 | 2 | 29 | 16 | 1,450 | 576 | 33 | 2,190 | 18,430 | 11.88 |
5 | 1 | M | 104 | 8 | 15 | 58 | 154 | 687 | 43 | 1,060 | 19,310 | 5.49 |
5 | 2 | F | 181 | 3 | 58 | 32 | 2,780 | 1,130 | 62 | 4,250 | 18,430 | 23.06 |
5 | 2 | M | 204 | 16 | 30 | 113 | 295 | 1,350 | 83 | 2,090 | 19,310 | 10.82 |
5 | 3 | F | 270 | 5 | 86 | 48 | 4,120 | 1,690 | 92 | 6,300 | 18,430 | 34.18 |
5 | 3 | M | 304 | 24 | 44 | 169 | 436 | 2,020 | 123 | 3,110 | 19,310 | 16.11 |
10 | 1 | F | 68 | 1 | 13 | 10 | 535 | 243 | 11 | 876 | 18,394 | 4.76 |
10 | 1 | M | 77 | 3 | 7 | 37 | 56 | 284 | 16 | 469 | 19,270 | 2.43 |
10 | 2 | F | 151 | 3 | 48 | 24 | 1,840 | 958 | 42 | 3,050 | 18,390 | 16.59 |
10 | 2 | M | 170 | 14 | 25 | 86 | 195 | 1,130 | 58 | 1,650 | 19,270 | 8.56 |
10 | 3 | F | 225 | 4 | 71 | 35 | 2,720 | 1,430 | 62 | 4,530 | 18,390 | 24.63 |
10 | 3 | M | 254 | 20 | 37 | 126 | 288 | 1,690 | 86 | 2,470 | 19,270 | 12.82 |
20 | 1 | F | 19 | 0 | 4 | 3 | 72 | 56 | 7 | 307 | 18,273 | 1.68 |
20 | 1 | M | 22 | 0 | 1 | 6 | 9 | 37 | 2 | 76 | 19,173 | 0.40 |
20 | 2 | F | 38 | 0 | 8 | 5 | 139 | 110 | 13 | 312 | 18,273 | 1.71 |
20 | 2 | M | 43 | 1 | 3 | 12 | 18 | 72 | 3 | 150 | 19,173 | 0.78 |
20 | 3 | F | 57 | 0 | 13 | 8 | 205 | 165 | 19 | 464 | 18,273 | 2.54 |
20 | 3 | M | 64 | 1 | 4 | 17 | 26 | 108 | 5 | 223 | 19,173 | 1.16 |
30 | 1 | F | 13 | 0 | 3 | 2 | 29 | 39 | 5 | 90 | 17,619 | 0.51 |
30 | 1 | M | 15 | 0 | 1 | 4 | 4 | 25 | 1 | 50 | 19,035 | 0.26 |
30 | 2 | F | 25 | 0 | 6 | 4 | 55 | 76 | 11 | 175 | 17,619 | 0.99 |
30 | 2 | M | 29 | 0 | 2 | 7 | 7 | 50 | 3 | 97 | 19,035 | 0.51 |
30 | 3 | F | 37 | 0 | 9 | 5 | 81 | 114 | 16 | 260 | 17,619 | 1.48 |
30 | 3 | M | 43 | 1 | 3 | 11 | 11 | 74 | 4 | 145 | 19,035 | 0.76 |
40 | 1 | F | 11 | 0 | 3 | 1 | 10 | 34 | 5 | 63 | 15,977 | 0.39 |
40 | 1 | M | 13 | 0 | 1 | 3 | 1 | 22 | 1 | 41 | 18,670 | 0.22 |
40 | 2 | F | 21 | 0 | 5 | 3 | 19 | 68 | 10 | 125 | 15,977 | 0.78 |
40 | 2 | M | 25 | 0 | 2 | 6 | 2 | 45 | 3 | 82 | 18,670 | 0.44 |
40 | 3 | F | 32 | 0 | 8 | 4 | 28 | 102 | 15 | 187 | 15,977 | 1.17 |
40 | 3 | M | 38 | 1 | 3 | 9 | 4 | 67 | 4 | 124 | 18,670 | 0.66 |