Abstract
Bladder cancer is one of the most common types of cancer. Most gene mutations related to bladder cancer are dominantly acquired gene mutations and are not inherited. Previous comparative transcriptome analysis of urinary bladder cancer and control samples has revealed a set of genes that may play a role in tumor progression. Here we set out to investigate further the expression of two candidate genes, centromere protein U (CENPU) and mitochondrial ribosomal protein s28 (MRPS28) to better understand their role in bladder cancer pathogenesis. Our results confirmed that CENPU is up-regulated in human bladder cancer tissues at mRNA and protein levels. Gain-of-function and loss-of-function studies in T24 human urinary bladder cancer cell line revealed a hierarchical relationship between CENPU and MRPS28 in the regulation of cell viability, migration and invasion activity. CENPU expression was also up-regulated in in vivo nude mice xenograft model of bladder cancer and mice overexpressing CENPU had significantly higher tumor volume. In summary, our findings identify CENPU and MRPS28 in the molecular pathogenesis of bladder cancer and suggest that CENPU enhances the progression of bladder cancer by promoting MRPS28 expression.
Globally, bladder cancer is an extremely common urological malignancy. In 2012, bladder cancer was diagnosed in approximately 429,800 patients worldwide and there were 165,100 bladder cancer-related deaths [1]. In 2015, in the United States, there were 74,000 new bladder cancer patients were diagnosed and 16,000 cases of bladder cancer-related death were occurred [2]. In the same year, approximately 80,500 patients were diagnosed with bladder cancer in China and about 32,900 patients died [3]. Although the clinical treatment of bladder cancer has progressed recently, the 5-year survival rate is still less than 60% [4]. Therefore, understanding the molecular mechanisms that underpin tumorigenesis is important to identify molecular targets for gene therapies of bladder cancer.
Analyzing transcriptome data proven to be beneficial assessing overall gene function, thereby elucidating the potential molecular mechanisms underlying pathological conditions, such as cancer. Recent transcriptome studies of bladder cancer have begun to elucidated the molecular mechanism of the disease and uncovered possible new candidates in the bladder cancer pathomechanisms, however follow-up studies on identified candidates is still largely missing [5,6].
Previous transcriptomic analysis on bladder tumors showed overexpression of mitochondrial ribosomal protein s28 (MRPS28) [5] and centromere protein U (CENPU, also known as MLF1IP and KLIP1) [7]. While direct link in bladder cancer is currently not available, we found evidence in other forms of cancer, such as adenocarcinoma [8] and gastric cancer [9] that two candidate genes, CENPU and MRPS28 form a functional synergistic network.
CENPU plays crucial role in orchestrating kinetochore-microtubule attachment via interacting with Hec1 [10]. CENPU is also a transcriptional repressor, which can inhibit herpes simplex virus thymidine kinase promoteractivity [11]. Simultaneously, CENPU is considered as a constitutive component of the centromere, which plays an important function in the progression of cell cycle [12]. Currently, CENPU has been found to possess a key regulatory role in tumorigenesis and cancer progression. Approximately 25% of mice overexpressing the CENPU gene developed breast cancer. Furthermore, the CENPU protein is highly expressed in human breast cancer tissues, and siRNA-mediated knockdown of CENPU significantly reduces the colony formation and migration rate of breast cancer cells [13]. It has also been reported that overexpression of CENPU is associated with poor prognosis in luminal breast cancer patients. Individuals with elevated CENPU expression have a shorter survival period, which is an independent prognostic factor for distant metastasis [14]. Furthermore, CENPU is rarely expressed in brain tissue; however, increased CENPU expression has been reported in various glioblastoma cell lines of human and rat. Therefore, CENPU might have a role in the pathogenesis of glioma [15]. Although elevated CENPU has been implicated in several human malignancies, few studies have investigated the expression patterns of CENPU in bladder cancer. Furthermore, the molecular mechanism by which CENPU affects bladder cancer progression has not been clearly defined.
Mitochondrial ribosomal proteins (MRP), which are encoded by nuclear gene, are consisted of a small 28S subunit and a large 39S subunit. MRPS28 is located on 8q21.1-q21.2, encoding 28S subunit protein, which is unique to mammals [16]. MRPS28 is synthesized as a precursor with an amino-terminal extension that serves as a mitochondrial targeting signal. Cells with disrupted copy of MRPS28 are unable to respire and spontaneously lost portions of their mitochondrial genomes with a high frequency [17]. At present, the involvement of MRPS28 in tumor development has not been well studied. Gopal and Rajkumar [16] reported that a variant of MRPS28 was differentially expressed in response to radiation in a cervical carcinoma-derived cell line. Currently, no studies have confirmed the role of MRPS28 in the development of bladder cancer.
In this research, we investigated the function of CENPU in bladder cancer. More importantly, the molecular mechanism of CENPU and MRPS28 in regulating bladder cancer development was further explored. As far as we know, this was the first time that the mechanism by which CENPU and MRPS28 expression affect bladder cancer progression was elaborated. Our findings will provide novel molecular target and theoretical basis for the target treatment of bladder cancer.
Tumor tissues and the corresponding cancer-adjacent normal tissues of 100 bladder cancer patients were collected during surgery and stored in liquid nitrogen. All of these patients were diagnosed with bladder cancer for the first time in our hospital from April 2017 to January 2020. All patients had no previous history of cancer-related treatment. The range of patients' age was 45–75 years old with an average age of (58.3 ± 11.7) years old. Among the 100 bladder cancer cases, 43 cases were female and 57 cases were male. Tumor tissues were obtained from the center of the lesion, while the corresponding cancer-adjacent normal tissues were collected from tissues about 10 mm away from the lesion tissues.
This study received written informed consent by all patients and was approved by the Ethics Committee of Bengbu Medical College (approval No. 2017-008).
Immortalized bladder epithelium cells (SV-HUC-1, Cat. No.C1241) and bladder cancer cells (T24, Cat. No. HZ-H024) were obtained from the Shanghai Institute of Cell Biology, Chinese Academy of Sciences. Both cells lines were cultured in Dulbecco’s Modified Eagle’s Medium (DMEM) containing 10% fetal bovine serum (FBS) with penicillin/streptomycin and incubated in 5% CO2 at 37°C.
T24 cells were collected after reaching 80% confluency, dispersed in FBS-free DMEM and seeded in 6-well plates (5 × 104 cells per well). The siRNAs targeting CENPU (si-CENPU) and MRPS28 (si-MRPS28), siRNA negative control targeting CENPU and MRPS28 (si-NC), pcDNA-CENPU and pcDNA-MRPS28 overexpression vector, and the pcDNA-CENPU and pcDNA-MRPS28 empty vectors, were obtained from GenePharma (Shanghai, China). The full maps of the pcDNA-CENPU and pcDNA-MRPS28 overexpression vectors were shown in Supplementary Fig. 1. After the cells reached 50% confluency, they were transfected with the aforementioned molecular transfectants using Lipofectamine 2000 (Thermo Fisher, Waltham, MA, USA). Co-transfections with the CENPU siRNA and pcDNA-MRPS28 overexpression vector and the MRPS28 siRNA and pcDNA-CENPU overexpression vector were also performed using Lipofectamine 2000 according to the manufacturer’s instructions. After 6 h, the liquid in each well was exchanged for fresh normal culture media. Cells were harvested after 48 h incubation for the subsequent studies.
Cell viability was determined using the cell counting kit-8 (CCK-8) assay. Cells dispersed in DMEM (10% FBS) were added to 96-well plates and incubated for 72 h. Each well contained 1 × 103 cells and 100 µl DMEM (10% FBS). CCK-8 volume of 10 µl was subsequently added into each well and plates were incubated for 2 h at 37°C. The absorbance (450 nm) of each well was measured with a microplate reader (BioTek Instruments Inc., Winooski, VT, USA), using the absorbance value of 10 µl of CCK-8 without cells as a blank. Cell viability was defined as follows: (experimental group absorbance – blank absorbance) / blank absorbance value × 100%.
Cells in the logarithmic growth phase were seeded into 6-well plates by adding 1 ml of DMEM (10% FBS) cell suspension (1 × 105 cells/ml). When cells reached a confluency of 80%, a scratch was made at the bottom of the wells with a sterile 200 µl pipette. The liquid in each well was removed and the remaining cells were washed twice with phosphate-buffered saline (PBS). The original scratch width was measured and marked as the scratch width at 0 h. Fresh DMEM (10% FBS) at a volume of 1 ml was subsequently added and plates were incubated at 37°C. Plates were removed from the incubator after 48 and 72 h to measure the width of the scratch using a light microscope (Olympus, Tokyo, Japan).
The Transwell migration assay was used to study the migration and invasion of cells using Transwell chambers (with or without Matrigel) (BD Biosciences, San Jose, CA, USA). Serum-free media cell suspension (1 × 105 cells/ml) was added to the upper chambers (200 µl) and the lower chambers contained DMEM with 10% FBS (600 µl). After 24 h incubation, the upper layer cells were removed with a cotton swab. Lower layer cells were fixed for 20 min in 95% ethanol and stained for 10 min in crystal violet (0.1%). After washing with PBS, cells were observed by light microscopy (Olympus) and counted using 5 random, unique fields of view.
Animal experiments in this study have been approved by the Animal Ethics Committee of Bengbu Medical College (approval No. 2017-021). Seventy-two nude mice (4–5 weeks old, 20–25 g) with body masses ranging from 11–13 g were purchased from the Beijing Vital River Laboratory Animal Technology Co., Ltd., China. T24 cells for the pcDNA-NC group, pcDNA-CENPU group, and pcDNA-CENPU + si-MRPS28 group were harvested and resuspended in PBS (3 × 107 cells/ml). Mice were given free access to food and water. After 2 weeks, mice were injected subcutaneously with 100 µl of cell suspension in the back. Notably, every three days, mice of each group were injected subcutaneously with 30 µg of Lipofectamine 2000-encapsulated pcDNA-CENPU empty vector, or pcDNA-CENPU overexpression vector, or pcDNA-CENPU overexpression vector and MRPS28 siRNA [18]. At 1, 2, 3, and 4 weeks after injection, 6 nude mice per group were randomly selected and sacrificed to collect tumors. The tumor volume was then measured. It should be noted that, after injection, the health and behavior of mice were monitored every 24 h. The health and behavior of mice were monitored by observing the daily diet weight, behavior, mental state, stool and urine weight and body weight.
The anesthesia and euthanasia of mice were as follows: Briefly, a total of 72 nude mice were used in this research. Before being sacrificed by cervical dislocation, mice were deeply anesthetized with an intraperitoneal injection of 60 mg/kg pentobarbital. The standard for deep anesthesia was that mice had no response to limb and head stimulation. Then mice were euthanized in about 10 s by cervical dislocation. Mice were judged to be dead if they met the following criteria: 10 s after cervical dislocation, mice were stopped breathing and did not respond to systemic stimuli. The duration of the experiment was about 10 min. The health and behavior of nude mice was observed every 3 min. During the research, no accidental death of nude mice was occurred.
Immunohistochemical staining for CENPU and MRPS28 was performed on paraffin-embedded tumors that were fixed in a 10% formaldehyde solution. After dewaxing, hydration, and antigen retrieval, the endogenous peroxidase activity was quenched using H2O2 (3%) and goat serum was used to block tumor tissue sections for 30 min at 4°C. After three PBS washes, Triton X-100 (0.5%) was added onto the tissue sections, followed by a 2 min incubation on ice. Sections were washed three times in PBS. A rabbit anti-mouse CENPU antibody or MRPS28 antibody (1:100 dilution, Wuhan Boster Bio-Engineering Co., Ltd., Wuhan, China) was added and the sections were incubated for 12 h incubation at 4°C. After incubation with the primary antibodies, sections were probed using biotinylated secondary antibodies for 2 h followed by three times washing with PBS. Sections were stained using 3,3’-diaminobenzidine color reaction and counterstained with hematoxylin. Following ethanol dehydration, sections were sealed with neutral resin and the integrated optical density (IOD) was analyzed using the Gel-Pro Analyzer V4.0 image analysis software system (Media Cybernetics, Rockville, MD, USA). Large IOD/Area value indicated more protein expression level.
Total RNA was extracted from cells or tissues using Trizol (Thermo Fisher Scientific). cDNA was synthesized by reverse transcription of isolated RNA using the PrimeScript miRNA cDNA Synthesis Kit (Applied Biosystems, Carlsbad, CA, USA). Expression of the target genes was quantified using the ABI 7500 Fast Real-Time PCR instrument (Applied Biosystems) with the following cycling conditions: pre-denaturation at 95°C, 5 min, then 40 cycles of 95°C, 15 s and 60°C, 1 min. Gene expression was calculated according to the 2–ΔΔCt method with glyceraldehyde-3-phosphate dehydrogenase (GAPDH) as an internal reference. The following primer sequences were used: CENPU, F, 5'-ATGAACTGCTTCGGTTAGAGC-3', R, 5'-TATTTCGCAGATGGCTTTCGG-3'. GAPDH, F, GTCGATGGCTAGTCGTAGCATCGAT, R, TGCTAGCTGGCATGCCCGATCGATC.
Total protein was extracted from tissues and cells in a radioimmunoprecipitation assay (RIPA) buffer (Sigma-Aldrich, St. Louis, MO, USA) containing aprotease inhibitor. A BCA protein assay kit (Pierce, Rockford, IL, USA) was used to measure protein concentration. Quantified proteins were separated by sodium dodecyl sulfate-polyacrylamide gel electrophoresis (SDS-PAGE) on a 10% gel and transferred onto a polyvinylidenefluoride (PVDF) membrane. Membranes were blocked in 5% skimmed milk for 1 h and incubated in a primary antibody (mouse anti-rabbit CENPU, MRPS28, N-cadherin, Slug, and Snail antibodies, 1:1,000 dilution; Cell Signaling Technology, Beverly, MA, USA) for 12 h at 4°C. After three times washing with TBST, membranes were incubated for 1 h with a goat anti-mouse IgG secondary antibody (Wuhan Boster Bio-Engineering Co., Ltd.). Membranes were washed three times with TBST and viewed on a LI-COR imager (LI-COR Biosciences, Lincoln, NE, USA) using β-actin as a loading control.
Statistical analyses were performed using SPSS version 20.0 (IBM Co., Armonk, NY, USA). Data were obtained from ≥ 3 independent experiments and values were represented as the mean ± standard deviation. A Student’s t-test was used to identify significant differences between two groups. The comparison among more than two groups was performed using one-way analysis of variance (ANOVA). Tukey's post hoc test was used to validate ANOVA for pairwise comparisons. All graphs were made using GraphPad Prism v5 (GraphPad Software, Inc., La Jolla, CA, USA). p < 0.05 was considered statistically significant.
The expression of CENPU was determined in tumor tissues and cancer-adjacent normal tissues from 100 bladder cancer cases by qRT-PCR and Western Blot. Compared to normal tissues (1.13 ± 0.11), CENPU mRNA transcripts were 2-fold higher in tumor tissues (2.45 ± 0.26) (p < 0.01). Similarly, at the protein level, CENPU was more than 3-fold higher in tumor tissues (0.34 ± 0.09) than in normal tissues (0.10 ± 0.03) (p < 0.001) (Fig. 1A, B). CENPU expression was also investigated in bladder epithelium cell line (SV-HUC-1) and bladder cancer cell line (T24). The relative CENPU mRNA and protein expression levels were significantly higher in T24 cells (mRNA: 2.28 ± 0.17) compared to SV-HUC-1 cells (mRNA: 1.01 ± 0.05) (p < 0.01) (Fig. 1C, D).
To further characterize the role of CENPU in bladder cancer pathogenesis, siRNA was used to knockdown CENPU expression in T24 cells. At the mRNA and protein levels, the expression of CENPU was significantly lower in cells transfected with si-CENPU compared to the control group (si-NCgroup). We also overexpressed CENPU by transfecting T24 cells with a pcDNA-CENPU overexpression vector. CENPU mRNA and protein was significantly higher in pcDNA-CENPU transfected cells (pcDNA-CENPU group) than in the control (pcDNA-NC group) (p < 0.01) (Fig. 2A, B). The effect of CENPU down-regulation or up-regulation on cell viability, migration, and invasion was subsequently examined. Cells with down-regulated CENPU expression (si-CENPU group) had reduced viability compared to the control group (si-NC group) (p < 0.01), while cells overexpressing CENPU (pcDNA-CENPU group) had increased viability relative to the control (pcDNA-NC group) (p < 0.01) (Fig. 2C). Cell invasion was measured in the four groups using a Transwell assay. As shown in Fig. 2D, less invasive cells were observed in the si-CENPU group compared to the si-NC group. However, the number of invasive cells in the pcDNA-CENPU group was remarkably higher than in the pcDNA-NC group. A cell scratch test was used to examine the migration ability of the cells. After 48 and 72 h, significantly wider scratch widths were found in the si-CENPU group compared to the si-NC group, while significantly narrower scratch widths were found in the pcDNA-CENPU group relative to pcDNA-NC group (Fig. 2E). The protein expression of N-cadherin, Slug, and Snail proteins was also examined by Western blot analysis. Lower amounts of all three proteins were detected in si-CENPU cells compared to si-NC group cells (p < 0.05 or p < 0.01). In contrast, the pcDNA-CENPU had more N-cadherin, Slug, and Snail proteins relative to the pcDNA-NC group (p < 0.05 or p < 0.01) (Fig. 2F).
We investigated the role of MRPS28 by down-regulating (si-MRPS28 group) (Fig. 3A) and up-regulating (pcDNA-MRPS28 group) (Fig. 3B) MRPS28 expression in T24 cells. Down-regulation of MRPS28 decreased the viability of cells compared to the si-NC group (p < 0.05), while up-regulation of MRPS28 increased cell viability relative to the pcDNA-NC group (p < 0.05) (Fig. 3C). Similarly, cell invasion was lower in MRPS28 down-regulated cells and higher in MRPS28 up-regulated cells compared to the respective control groups (Fig. 3D). After incubation for 48 and 72 h, wider scratch widths were observed in the si-MRPS28 group compared to the si-NC group. However, at both time points, the scratch widths of the pcDNA-MRPS28 group were significantly narrower than that of the pcDNA-NC group (Fig. 3E).
In order to explore the link between CENPU and MRPS28, we simultaneously down-regulated CENPU and up-regulated MRPS28 in T24 cells (si-CENPU + pcDNA-MRPS28 group). Down-regulation of CENPU alone (si-CENPU group) significantly decreased the viability of cells relative to the control group (si-NC group) (p < 0.01). However, simultaneous up-regulation of MRPS28 (si-CENPU + pcDNA-MRPS28 group) increased cell viability relative to the si-CENPU group (p < 0.01) (Fig. 4A). Similarly, more invasive cells (Fig. 4B) and higher migration (Fig. 4C) abilities were detected in the si-CENPU + pcDNA-MRPS28 group compared to the si-CENPU group. In addition, we simultaneously up-regulated CENPU and down-regulated MRPS28 in T24 cells (pcDNA-CENPU + si-MRPS28 group). Cells with up-regulation of CENPU (pcDNA-CENPU group) had higher cell viability (p < 0.01) and more invasive and migrated cells relative to the control group (pcDNA-NC group). Simultaneous down-regulation of MRPS28 (pcDNA-CENPU + si-MRPS28 group) significantly decreased the viability (p < 0.01), invasion, and migration of T24 cells compared to the pcDNA-CENPU group (Fig. 4D–F).
Mice with up-regulated CENPU expression (pcDNA-CENPU group) and simultaneously down-regulated MRPS28 expression (pcDNA-CENPU + si-MRPS28 group) were used to examine the role of CENPU/MRPS28 in bladder cancer progression in vivo. In this study, a total of 3 × 106 cells were injected subcutaneously into nude mice. At week 1, tumor volume were comparable between the experimental and control (pcDNA-NC) groups. However, within 2–4 weeks, CENPU-overexpressing mice (pcDNA-CENPU) had significantly higher tumor volume compared to the pcDNA-NC group (p < 0.05). In contrast, from 1–4 weeks, CENPU-overexpressing mice with down-regulated MRPS28 expression (pcDNA-CENPU + si-MRPS28 group) had significantly lower tumor volume compared to the pcDNA-CENPU group (p < 0.05 or p < 0.01 or p < 0.001). After 4 weeks of injection, the maximum tumor volume was about 1.3 cm3, which was in line with the recommendations of the University of Pennsylvania Institutional Animal Care and Use Committee guidelines (Fig. 5A). CENPU and MRPS28 expression at the protein level was established in tumor tissues by immunohistochemistry and Western blot analysis. In comparison to the pcDNA-NC group, more CENPU protein was detected in the tumor tissues of CENPU-overexpressing mice (pcDNA-CENPU group) (p < 0.001). As expected, more CENPU protein and less MRPS38 protein was detected in CENPU-overexpressing mice with down-regulated MRPS28 expression (pcDNA-CENPU + si-MRPS28 group) compared to the pcDNA-NC group (p < 0.001) (Fig. 5B, C). The levels of N-cadherin, Slug, and Snail proteins in tumor tissues were examined by Western blot analysis. Tumor tissues of CENPU-overexpressing mice (pcDNA-CENPU group) had a higher amount of N-cadherin, Slug, and Snail proteins compared to the pcDNA-NC group (p < 0.001). Furthermore, the amount of all three proteins was lower in tumor tissues of CENPU-overexpressing mice with down-regulated MRPS28 expression (pcDNA-CENPU + si-MRPS28 group) compared to the pcDNA-CENPU group (p < 0.001) (Fig. 5D).
Despite recent progress, bladder cancer remains a frequent diagnosis that is associated with high mortality rates. Chemotherapy is one of the most common approaches to bladder cancer treatment. However, drug resistance caused by long-term chemotherapy poses a significant challenge for successful treatment strategies. Understanding the pathogenesis of bladder cancer is essential for developing alternative treatment strategies. In this study, we investigated for the first time that CENPU enhanced bladder cancer progression by promoting MRPS28 expression.
Previous studies have found that CENPU proteins are associated with multiple human malignancies [19]. The up-regulation of CENPU is involved in the progression of glioblastoma, breast cancer and familial colorectal cancer [14,15,20]. However, few studies have examined the role of CENPU in bladder cancer progression. Recently, Wang et al. [7] proposed CENPU as a potential therapeutic target for bladder cancer treatment. Increased expression of CENPU in bladder cancer tissues was found to be associated with larger tumor size, advanced TNM stage, and lower survival rates. CENPU-knockdown in human bladder cancer cell lines inhibited cell proliferation and arrested cell cycle progression in the G1 phase. Similarly, our data showed that CENPU is significantly up-regulated in bladder cancer. Furthermore, overexpression of CENPU enhanced in vitro malignant phenotype and in vivo growth of bladder cancer cells. These findings suggested that CENPU promoted the progression of bladder cancer.
Few researches have investigated the mechanisms underpinning CENPU up-regulation in bladder cancer development. Knockdown of CENPU in vitro could affect the regulation of several proteins that are involved in the HMGB1 signaling pathway, such as IL6, IL1B, TNF, and HMGB1 [7]. HMGB1 up-regulation is associated with the progression of bladder cancer and a poor prognosis, while decreased HMGB1 expression suppresses the bioactivity of bladder cancer cells [21-23]. It has been reported that CENPU facilitates ovarian cancer metastasis by enhancing HMGB2 expression [10]. Although this study did not investigate the HMGB family, we have demonstrated for the first time that CENPU enhances the expression of MRPS28. Immunohistochemistry and Western blot on xenograft tumors shows that CENPU overexpression elevates the expression of MRPS28 protein. Both in vitro and in vivo studies illustrated that MRPS28 silencing reverses the promoting effect of CENPU on bladder cancer progression. This represents an important finding for understanding the mechanism of bladder cancer progression and potential for targeted treatments.
Other members of the MRP family have been previously implicated in the development and progression of some human malignancies. It has been demonstrated that decreased MRPS23 expression suppresses metastasis in rats with breast cancer [24]. Furthermore, enhanced MRPS18-2 expression has been associated with prostate cancer, and promotes the migration of prostate cancer cells migration by inducing epithelial to mesenchymal cell transition (EMT) [25]. Another MRPS protein, MRPS18a, is rarely expressed in normal human breast cells, but aberrantly overexpressed in breast cancer cells [26]. However, no studies had previously investigated MRP family expression in bladder cancer. We have provided the first evidence of the positive regulation of MRPS28 by CENPU in bladder cancer. MRPS28 expression was elevated via CENPU overexpression, which further enhanced bladder cancer cells in vitro malignant phenotype and in vivo growth. Based on our findings, we will continue to evaluate the impact of MRPS28 on bladder cancer progression in our future research.
It has been reported that CENPU up-regulation enhances EMT in human cancers, including breast cancer [13]. EMT is important for the occurrence, development, invasion and metastasis of malignant tumors [27]. EMT occurs by decreased adhesion between cells and the loss of cell polarity, resulting in the transformation of the originally polar epithelial cells to mesenchymal cells with strong migration and invasion capacities [28]. We found that the levels of three EMT-associated proteins, N-cadherin, Slug, and Snail, are increased by overexpressing CENPU. N-cadherin is a calcium-dependent transmembrane glycoprotein that is involved in the regulation of calcium-mediated cell-to-cell adhesion, including homogenous adhesion, cell polarity, and morphology maintenance, as well as cell aggregation and migration [29]. N-cadherin is an interstitial marker of EMT, which is positively correlated with tumor invasion. Increased expression of N-cadherin is associated with the enhanced transformation of tumor cells from epithelial to mesenchymal, resulting in enhanced vascular proliferation and invasive ability of tumors [30]. Both Slug and Snail are zinc-finger transcription factors belonging to the Snail family, which are key regulators of EMT [31]. Slug and Snail inhibit the expression of E-cadherin (a cell adhesion molecule), therefore decreasing cell-cell adhesion and enhancing infiltration and metastasis [32,33]. Based on our data and previous studies, we speculated that CENPU might promote EMT by increasing the expression of N-cadherin, Slug, and Snail proteins, thereby enhancing the migration and invasion of bladder cancer cells.
Of course, this study has limitation. The signaling relationship between CENPU and MRPS28 in bladder cancer should be researched. However, due to the limitations of the laboratory, we are currently unable to conduct experiments to study this point. This will be the focus in our future research. In conclusion, we have provided evidence that CENPU up-regulation might enhance the progression of bladder cancer by promoting MRPS28 expression and stimulating EMT. Therefore, CENPU could function as a useful biomarker for diagnosis and potential therapeutic target for the treatment of bladder cancer.
Supplementary data including one figure can be found with this article online at https://doi.org/10.4196/kjpp.2021.25.2.119.
ACKNOWLEDGEMENTS
The study was supported by National Natural Science Foundation of China (No. 81702495); Natural Science Research Projects In Bengbu Medical College (No. BYKY1738ZD, BYKY1723ZD); AnHui Natural Science Foundation (No. 1808085QH279); Anhui natural science foundation (No. 1808085MH293) and University Natural Science Research Project in Anhui Province (No. KJ2019A0308).
Notes
REFERENCES
1. Torre LA, Bray F, Siegel RL, Ferlay J, Lortet-Tieulent J, Jemal A. 2015; Global cancer statistics, 2012. CA Cancer J Clin. 65:87–108. DOI: 10.3322/caac.21262. PMID: 25651787.


2. Siegel RL, Miller KD, Jemal A. 2015; Cancer statistics, 2015. CA Cancer J Clin. 65:5–29. DOI: 10.3322/caac.21254. PMID: 25559415.


3. Chen W, Zheng R, Baade PD, Zhang S, Zeng H, Bray F, Jemal A, Yu XQ, He J. 2016; Cancer statistics in China, 2015. CA Cancer J Clin. 66:115–132. DOI: 10.3322/caac.21338. PMID: 26808342.


4. Huang B, Zhang J, Zhang X, Huang C, Hu G, Li S, Xie T, Liu M, Xu Y. 2017; Suppression of LETM1 by siRNA inhibits cell proliferation and invasion of bladder cancer cells. Oncol Rep. 38:2935–2940. DOI: 10.3892/or.2017.5959. PMID: 29048663.


5. Volanis D, Zaravinos A, Kadiyska T, Delakas D, Zoumpourlis V, Spandidos DA. 2011; Expression profile of Rho kinases in urinary bladder cancer. J BUON. 16:511–521. PMID: 22006759.

6. Chen X, Jiang F, Jia C, Liu M, Nan Y, Qu L, Kong Q, Hou F, Luo W, Na W, Jin X, Tan J. 2019; Comprehensive gene expression analysis in NMIBC using RNA-seq reveals new therapy strategies. Front Oncol. 9:523. DOI: 10.3389/fonc.2019.00523. PMID: 31293967. PMCID: PMC6604748.



7. Wang S, Liu B, Zhang J, Sun W, Dai C, Sun W, Li Q. 2017; Centromere protein U is a potential target for gene therapy of human bladder cancer. Oncol Rep. 38:735–744. DOI: 10.3892/or.2017.5769. PMID: 28677729. PMCID: PMC5562008.



8. Zhu X, Wang D, Lin Q, Wu G, Yuan S, Ye F, Fan Q. 2019; Screening key lncRNAs for human rectal adenocarcinoma based on lncRNA-mRNA functional synergistic network. Cancer Med. 8:3875–3891. DOI: 10.1002/cam4.2236. PMID: 31116002. PMCID: PMC6639256.



9. Vecchi M, Nuciforo P, Romagnoli S, Confalonieri S, Pellegrini C, Serio G, Quarto M, Capra M, Roviaro GC, Contessini Avesani E, Corsi C, Coggi G, Di Fiore PP, Bosari S. 2007; Gene expression analysis of early and advanced gastric cancers. Oncogene. 26:4284–4294. DOI: 10.1038/sj.onc.1210208. PMID: 17297478.


10. Li H, Zhang H, Wang Y. 2018; Centromere protein U facilitates metastasis of ovarian cancer cells by targeting high mobility group box 2 expression. Am J Cancer Res. 8:835–851. PMID: 29888106. PMCID: PMC5992511.
11. Pan HY, Zhang YJ, Wang XP, Deng JH, Zhou FC, Gao SJ. 2003; Identification of a novel cellular transcriptional repressor interacting with the latent nuclear antigen of Kaposi's sarcoma-associated herpesvirus. J Virol. 77:9758–9768. DOI: 10.1128/JVI.77.18.9758-9768.2003. PMID: 12941884. PMCID: PMC224565.



12. Lin SY, Lv YB, Mao GX, Chen XJ, Peng F. 2018; The effect of centromere protein U silencing by lentiviral mediated RNA interference on the proliferation and apoptosis of breast cancer. Oncol Lett. 16:6721–6728. DOI: 10.3892/ol.2018.9477. PMID: 30405814. PMCID: PMC6202484.



13. Zhang J, Zhang X, Hu Y, Li J, Liu J, Zhang S, Su W. 2015; Abstract P4-05-11: CENPU acts as a new proto-oncogene to regulate tumorigenesis and cancer metastasis in breast cancer. Cancer Res. 75(9 Suppl):P4-05-11. DOI: 10.1158/1538-7445.SABCS14-P4-05-11.


14. Huang DP, Luo RC. 2016; MLF1IP is correlated with progression and prognosis in luminal breast cancer. Biochem Biophys Res Commun. 477:923–926. DOI: 10.1016/j.bbrc.2016.06.159. PMID: 27378428.


15. Hanissian SH, Teng B, Akbar U, Janjetovic Z, Zhou Q, Duntsch C, Robertson JH. 2005; Regulation of myeloid leukemia factor-1 interacting protein (MLF1IP) expression in glioblastoma. Brain Res. 1047:56–64. DOI: 10.1016/j.brainres.2005.04.017. PMID: 15893739.


16. Gopal G, Rajkumar T. 2005; Variant of mitochondrial ribosomal protein s28 (mrps28) gene is differentially expressed in response to radiation in a cervical carcinoma derived cell line. Indian J Biochem Biophys. 42:81–86. PMID: 23923566.

17. Dang H, Ellis SR. 1990; Structural and functional analyses of a yeast mitochondrial ribosomal protein homologous to ribosomal protein S15 of Escherichia coli. Nucleic Acids Res. 18:6895–6901. DOI: 10.1093/nar/18.23.6895. PMID: 2263452. PMCID: PMC332747.


18. Liang Z, Wang X, Xu X, Xie B, Ji A, Meng S, Li S, Zhu Y, Wu J, Hu Z, Lin Y, Zheng X, Xie L, Liu B. 2017; MicroRNA-608 inhibits proliferation of bladder cancer via AKT/FOXO3a signaling pathway. Mol Cancer. 16:96. DOI: 10.1186/s12943-017-0664-1. PMID: 28549468. PMCID: PMC5446711.


19. Suzuki H, Arakawa Y, Ito M, Saito S, Takeda N, Yamada H, Horiguchi-Yamada J. 2007; MLF1-interacting protein is mainly localized in nucleolus through N-terminal bipartite nuclear localization signal. Anticancer Res. 27(3B):1423–1430. PMID: 17595757.

20. Dominguez-Valentin M, Therkildsen C, Veerla S, Jönsson M, Bernstein I, Borg A, Nilbert M. 2013; Distinct gene expression signatures in lynch syndrome and familial colorectal cancer type x. PLoS One. 8:e71755. DOI: 10.1371/journal.pone.0071755. PMID: 23951239. PMCID: PMC3741139.


21. Wang W, Jiang H, Zhu H, Zhang H, Gong J, Zhang L, Ding Q. 2013; Overexpression of high mobility group box 1 and 2 is associated with the progression and angiogenesis of human bladder carcinoma. Oncol Lett. 5:884–888. DOI: 10.3892/ol.2012.1091. PMID: 23426143. PMCID: PMC3576183.


22. Yang GL, Zhang LH, Bo JJ, Huo XJ, Chen HG, Cao M, Liu DM, Huang YR. 2012; Increased expression of HMGB1 is associated with poor prognosis in human bladder cancer. J Surg Oncol. 106:57–61. DOI: 10.1002/jso.23040. PMID: 22237993.


23. Huang Z, Zhong Z, Zhang L, Wang X, Xu R, Zhu L, Wang Z, Hu S, Zhao X. 2015; Down-regulation of HMGB1 expression by shRNA constructs inhibits the bioactivity of urothelial carcinoma cell lines via the NF-κB pathway. Sci Rep. 5:12807. DOI: 10.1038/srep12807. PMID: 26239046. PMCID: PMC4523846.


24. Gao Y, Li F, Zhou H, Yang Y, Wu R, Chen Y, Li W, Li Y, Xu X, Ke C, Pei Z. 2017; Down-regulation of MRPS23 inhibits rat breast cancer proliferation and metastasis. Oncotarget. 8:71772–71781. DOI: 10.18632/oncotarget.17888. PMID: 29069745. PMCID: PMC5641088.


25. Mushtaq M, Jensen L, Davidsson S, Grygoruk OV, Andrén O, Kashuba V, Kashuba E. 2018; The MRPS18-2 protein levels correlate with prostate tumor progression and it induces CXCR4-dependent migration of cancer cells. Sci Rep. 8:2268. DOI: 10.1038/s41598-018-20765-8. PMID: 29396484. PMCID: PMC5797078.



26. Sørensen KM, Meldgaard T, Melchjorsen CJ, Fridriksdottir AJ, Pedersen H, Petersen OW, Kristensen P. 2017; Upregulation of Mrps18a in breast cancer identified by selecting phage antibody libraries on breast tissue sections. BMC Cancer. 17:19. DOI: 10.1186/s12885-016-2987-5. PMID: 28056857. PMCID: PMC5376696.



27. He SJ, Xiang CQ, Zhang Y, Lu XT, Chen HW, Xiong LX. 2017; Recent progress on the effects of microRNAs and natural products on tumor epithelial-mesenchymal transition. Onco Targets Ther. 10:3435–3451. DOI: 10.2147/OTT.S139546. PMID: 28744148. PMCID: PMC5513877.


28. Li Y, Gao A, Yu L. 2016; Monitoring of TGF-β 1-induced human lung adenocarcinoma A549 cells epithelial-mesenchymal transformation process by measuring cell adhesion force with a microfluidic device. Appl Biochem Biotechnol. 178:114–125. DOI: 10.1007/s12010-015-1862-1. PMID: 26394790.


29. Jia S, Wang W, Hu Z, Shan C, Wang L, Wu B, Yang Z, Yang X, Lei D. 2015; BDNF mediated TrkB activation contributes to the EMT progression and the poor prognosis in human salivary adenoid cystic carcinoma. Oral Oncol. 51:64–70. DOI: 10.1016/j.oraloncology.2014.10.008. PMID: 25456007.


30. Sun H, Liu M, Wu X, Yang C, Zhang Y, Xu Z, Gao K, Wang F. 2017; Overexpression of N-cadherin and β-catenin correlates with poor prognosis in patients with nasopharyngeal carcinoma. Oncol Lett. 13:1725–1730. DOI: 10.3892/ol.2017.5645. PMID: 28454316. PMCID: PMC5403301.



31. Tang Y, Weiss SJ. 2017; Snail/Slug-YAP/TAZ complexes cooperatively regulate mesenchymal stem cell function and bone formation. Cell Cycle. 16:399–405. DOI: 10.1080/15384101.2017.1280643. PMID: 28112996. PMCID: PMC5351930.



32. Jung H, Kim B, Moon BI, Oh ES. 2016; Cytokeratin 18 is necessary for initiation of TGF-β1-induced epithelial-mesenchymal transition in breast epithelial cells. Mol Cell Biochem. 423:21–28. DOI: 10.1007/s11010-016-2818-7. PMID: 27734227.


33. Jeon SY, Hwang KA, Kim CW, Jeung EB, Choi KC. 2017; Altered expression of epithelial mesenchymal transition and pluripotent associated markers by sex steroid hormones in human embryonic stem cells. Mol Med Rep. 16:828–836. DOI: 10.3892/mmr.2017.6672. PMID: 28586020.


Fig. 1
Abnormally high expression of centromere protein U (CENPU) in bladder cancer cells and tissues.
Significantly higher CENPU mRNA (A) and protein (B) expression was found in tumor tissues compared to cancer-adjacent normal tissues. Significantly higher CENPU mRNA (C) and protein (D) expression was found in bladder cancer cells (T24) compared to bladder epithelium cells (SV-HUC-1). Scale bar represents 1 cm. **p < 0.01. ***p < 0.001.
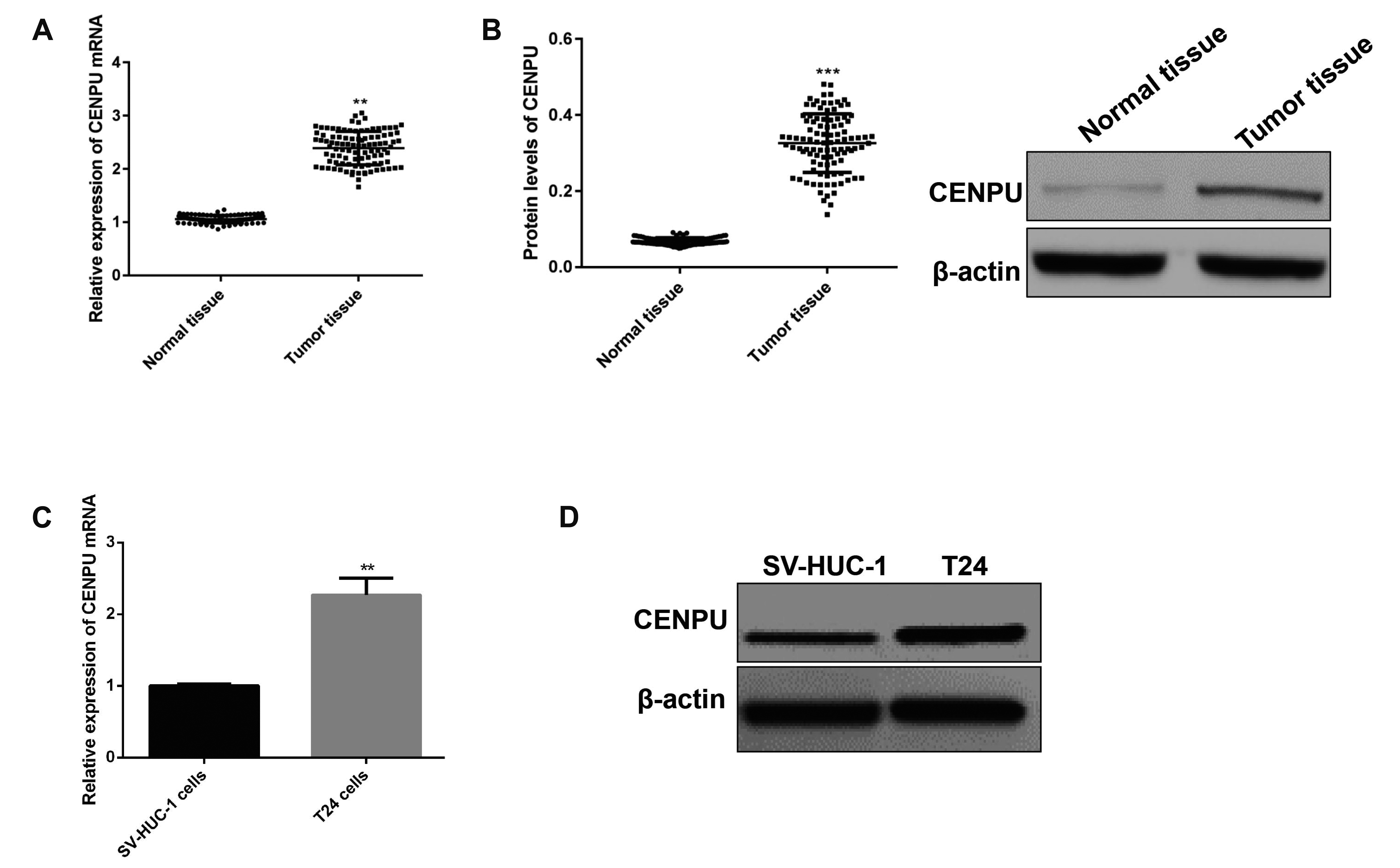
Fig. 2
Overexpression of centromere protein U (CENPU) enhanced the viability, migration, and invasion of T24 cells.
(A, B) CENPU mRNA and protein expression of each group was measured by qRT-PCR and Western blotting. (C) The cell viability of each group was measured using the cell counting kit-8 (CCK-8) assay. (D) The cell invasion ability was assessed using Transwell assay, magnification ×100. (E) Cell scratch test was performed to evaluate the cell migration abilities. (F) N-cadherin, Slug, and Snail protein expression in transfected T24 cells of each group determined by Western analysis. *p < 0.05 or **p < 0.01 vs. si-NC group or pcDNA-NC group.

Fig. 3
Overexpression of mitochondrial ribosomal protein s28 (MRPS28) promoted the viability, migration, and invasion of T24 cells.
(A, B) The protein expression of MRPS28 for each group was determined by Western blot. (C) Cell viability of each group using the cell counting kit-8 (CCK-8) assay. (D) Cell invasion ability using Transwell assay, magnification ×100. (E) Cell scratch test to evaluate the cell migration abilities. *p < 0.05 vs. si-NC group or pcDNA-NC group.

Fig. 4
Centromere protein U (CENPU) enhanced the viability, invasion, and migration of T24 cells by promoting mitochondrial ribosomal protein s28 (MRPS28) expression.
(A, D) Cell viability of each group was detected using the cell counting kit-8 (CCK-8) assay. (B, E) Cells invasion ability was measured using Transwell assay, magnification ×100. (C, F) Transwell assay was used to evaluate the cell migration abilities, magnification ×100. **p < 0.01 vs. si-NC group or pcDNA-NC group. ##p < 0.01 vs. si-CENPU group or pcDNA-CENPU group.

Fig. 5
Centromere protein U (CENPU) promoted bladder cancer development in vivo by elevating mitochondrial ribosomal protein s28 (MRPS28) expression.
(A) Picture of tumor tissues and volume of tumors from nude mice in each group from 1–4 weeks. (B, C) Immunohistochemistry and Western blot analysis was used to measure the expression of CENPU and MRPS28 proteins in tumor tissues of nude mice. (D) Western blot analysis to measure N-cadherin, Slug and Snail protein expression in tumor tissues. In this study, T24 cells were injected subcutaneously into the back of nude mice. *p < 0.05 or ***p < 0.001 vs. pcDNA-NC group. #p < 0.05 or ##p < 0.01 or ###p < 0.001 vs. pcDNA-CENPU group.
