Abstract
27-Hydroxycholesterol (27OHChol) exhibits agonistic activity for liver X receptors (LXRs). To determine roles of the LXR agonistic activity in macrophage gene expression, we investigated the effects of LXR inhibition on the 27OHChol-induced genes. Treatment of human THP-1 cells with GSK 2033, a potent cell-active LXR antagonist, results in complete inhibition in the transcription of LXR target genes (such as LXRα and ABCA1) induced by 27OHChol or a synthetic LXR ligand TO 901317. Whereas expression of CCL2 and CCL4 remains unaffected by GSK 2033, TNF-α expression is further induced and 27OHChol-induced CCL3 and CXCL8 genes are suppressed at both the transcriptional and protein translation levels in the presence of GSK 2033. This LXR antagonist downregulates transcript levels and surface expression of CD163 and CD206 and suppresses the transcription of CD14, CD80, and CD86 genes without downregulating their surface levels. GSK 2033 alone had no effect on the basal expression levels of the aforementioned genes. Collectively, these results indicate that LXR inhibition leads to differential regulation of 27-hydroxycholesterol-induced genes in macrophages. We propose that 27OHChol induces gene expression and modulates macrophage functions via LXR-dependent and -independent mechanisms.
Oxysterols are oxygenated derivatives of cholesterol. As compared to cholesterol, they contain an additional hydroxy, epoxide or ketone group in the sterol nucleus, and/or a hydroxyl group in the side chain [1]. 27-Hydroxycholesterol (27OHChol) is a side-chain oxysterol oxygenated at the 27th carbon atom of cholesterol. This oxysterol is produced via oxidation by sterol 27-hydroxylase (CYP27A1), and metabolized via 7α-hydroxylation for bile acid synthesis in the liver [1,2]. 27OHChol can also be formed in peripheral tissues either via enzymatic or non-enzymatic mechanisms [2,3]. The production of 27OHChol is enhanced in atherosclerotic lesions of the artery, and increasing amounts detected in lesions are associated with increasing macrophages [4,5]. The biological function of 27OHChol in peripheral tissues differs from that in the liver. 27OHChol enhances inflammation and triggers an immune response by activating monocytes/macrophages. Following activation, they express chemokines, including chemokine (C-C motif) ligand (CCL) 2, CCL3 and chemokine (C-X-C motif) ligand (CXCL) 8, and pattern recognition receptors that respond to the bacterial pathogen-associated molecular patterns [6-8]. 27OHChol also induces molecules involved in T cell activation on the surface of monocytic cells [9]. Vascular smooth muscle cells and T cells, however, do not express such molecules in response to 27OHChol [10,11]. These findings indicate that macrophages are the major cell type whose gene expression is influenced by 27OHChol.
27OHChol is a functional liver X receptors (LXR) agonist [12]. LXRα (NR1H3) and LXRβ (NR1H2) are two isoforms of LXRs expressed with overlapping, but a distinctive pattern. LXRα is dominant in the liver and expressed primarily in the intestine, adipose tissue, and macrophages, whereas LXRβ is widely expressed [13-15]. Since LXRs are members of the nuclear hormone receptor superfamily of ligand-activated transcription factors, they exert their biological effects by controlling the expression of target genes [15]. Activation of LXRs with 27OHChol increases the transcription of LXR-responsive genes involved in cholesterol efflux, such as ABCA1 and ABCG1 in macrophages [12,14]. These findings indicate that 27OHChol induces gene expression via LXRs. However, it is unknown whether the LXR agonistic activity of 27OHChol is involved in the induction of genes other than for cholesterol metabolism.
This study was therefore undertaken to determine the effects of GSK 2033, a potent cell-active LXR antagonist, on the transcription of 27OHChol-induced genes, including inflammatory and cell surface molecules as well as LXR target genes. Our results demonstrate that GSK 2033 differentially regulates the 27OHChol-induced genes in macrophages.
The human THP-1 monocyte/macrophage cell line (ATCC, #TIB-202) was purchased from the American Type Culture Collection (ATCC, Manassas, VA, USA). THP-1 cells were cultured in RPMI 1640 medium supplemented with 10% fetal bovine serum (FBS) at 37°C in a humidified atmosphere of 5% CO2. Penicillin (50 U/ml) and streptomycin (50 μg/ml) were added to prevent bacterial contamination. 27OHChol and antibodies against LXRα/β, CD14, CD80, and CD86 were purchased from Santa Cruz Biotechnology (Santa Cruz, CA, USA). GSK 2033 and TO 901317 were purchased from Sigma-Aldrich (St. Louis, MO, USA). Anti-ABCA1 antibody was purchased from Invitrogen (Eugene, OR, USA). Anti-CD163 antibody conjugated with FITC and anti-CD206 antibody conjugated with PE were purchased from BioLegend (San Diego, CA, USA).
Total RNA isolated were reverse-transcribed for 1 h at 42°C with 100 U Moloney murine leukemia virus reverse transcriptase in a 10 μl reaction volume, containing 50 mM Tris-HCl (pH 8.3 at 25°C), 55 mM KCl, 3 mM MgCl2, 10 mM DTT, 1 μg oligo (dT) 15 primers, 0.125 mM each dNTP, and 40 U RNase inhibitor. Subsequent qPCR was performed in triplicate using a LightCycler 96 Real-Time PCR System (Roche, Mannheim, Germany), as previously described [8]. Each 20 μl reaction mixture consisted of 10 μl SYBR Green Master Mix, 2 μl forward and reverse primers (10 pM each) of the gene to be quantified, and cDNA template. The thermal cycling conditions consisted of 95°C for 10 min, followed by 45 cycles of 95°C for 10 sec, 50°C for 10 sec, and 72°C for 10 sec. The relative expression of each gene was calculated as the ratio to the GAPDH gene using the LightCycler 96 software (Version 1.1.0.1320; Roche). The primers used were as follows in Table 1.
Cell lysates were separated by 10% SDS-PAGE, and resolved proteins were transferred to nitrocellulose membranes. After blocking for 1 h in 1% skim milk in TBS (pH 7.4) containing 0.05% Tween-20, membranes were incubated with antibodies against LXRα/β, ABCA1 or β-actin at 4°C overnight. Membranes were washed three times with 0.05% Tween 20/TBS for 10 min each and incubated for 1 h with HRP conjugated secondary Abs (1:5,000 dilution) at room temperature. After washing with 0.05% Tween 20/TBS, membranes were exposed to chemiluminescent detection reagents (Pierce ECL Western Blotting Substrate; Thermo Scientific, Rockford, IL, USA). Chemiluminescence images were captured by using an Amersham Imager 680 (GE Healthcare Life Scicences, Pittsburgh, PA, USA).
THP-1 cells were harvested by centrifugation at 200 × g for 5 min at room temperature and incubated for 2 h with antibodies against CD14, CD80 or CD86 (1:100 dilution) in the FACS buffer containing 2 mM EDTA and 0.2% BSA in PBS. After washing with cold-PBS, cells were incubated for 1 h with Alexa Fluor 488-conjugated secondary antibodies (1:200 dilution) at 4°C. Or the harvested cells were incubated with the fluorescent dye-conjugated antibodies (1:100 dilution) against CD163 or CD206 for 1 h at 4°C. Cells were washed with cold-PBS, resuspended in 1% paraformaldehyde, and analyzed by flow cytometry.
The amounts of CCL2, CCL3, CCL4, CXCL8, and TNF-α secreted in culture media were quantified using commercially available ELISA kits (R&D Systems, Minneapolis, MN, USA), following the manufacturer's instructions.
Statistical analysis was performed via one-way analysis of variance, followed by Dunnett's multiple comparison test, using PRISM (version 5.0) (GraphPad Software Inc., San Diego, CA, USA). A p-value less than 0.05 (p < 0.05) is considered to indicate a statistically significant difference.
The human LXRα and ABCA1/ABCG1 are targets for regulation by LXR, and ligands for the receptor induce their expression in macrophages [12]. We investigated whether 27OHChol affects expression of the genes along with TO 901317, a synthetic LXR ligand. We observed levels of LXRα transcripts were elevated to 2.25- and 3.49-fold following stimulation with 27OHChol and TO 901317, respectively (Fig. 1A). Assessing the transcript levels of ABCA1 revealed increases up to 1.31- and 8.04-fold by stimulation with 27OHChol and TO 901317, respectively (Fig. 1B). ABCG1 transcripts increased 4.33- and 28.5-fold after stimulation with 27OHChol and TO 901317, respectively (Supplementary Fig. 1). Investigating further whether expressions of LXRα and ABCA1/ABCG1 genes were influenced by GSK 2033, a potent cell-active LXR antagonist [16], revealed that TO 901317- or 27OHChol-induced gene transcription of LXRα and ABCA1/ABCG1 was blocked in the presence of GSK 2033. In line with the results of real-time PCR, 27OHChol and TO 901317 elevated protein levels of LXRα or ABCA1, which was completely inhibited by GSK 2033 (Fig. 1C). These results indicate that although less effective than the synthetic LXR agonist, 27OHChol induces expression of the LXR target genes, and the induction is impaired by GSK 2033.
27OHChol induces expression of C-C chemokines, including CCL2, CCL3, and CCL4 [11]. We undertook to determine effects of GSK 2033 on the transcription of chemokines to understand the function of LXR inhibition in the induction of C-C chemokines. 27OHChol exposure elevated the levels of CCL2, CCL3, and CCL4 gene transcripts and their gene products (Fig. 2A and B). Although GSK 2033 did not significantly reduce expression of the CCL2 and CCL4 genes, the levels of CCL3 transcripts and protein were profoundly decreased in the presence of GSK 2033. The antagonist alone did not alter the transcript levels and secretion of the C-C chemokines. These results suggest that of the three C-C chemokines, GSK 2033 suppresses expression of the CCL3 gene alone.
27OHChol as well as LXR agonists induces the CXCL8 and TNF-α genes which are involved in acute-phase inflammatory responses [8,17,18]. We investigated the roles of LXR agonistic activity of 27OHChol in the expression of CXCL8 and TNF-α. Treatment with GSK 2033 resulted in suppressing the transcription of CXCL8 gene, whereas the transcription of TNF-α further enhanced in the presence of GSK 2033 (Fig. 3A). We examined effects of GSK 2033 on CXCL8 and TNF-α secretion. GSK 2033 impaired CXCL8 production, but promoted secretion of TNF-α (Fig. 3B). These results suggest that LXR-agonistic activity of 27OHChol is associated with a repressive effect on TNF-α expression.
27OHChol affect levels of both M1 and M2 markers of macrophages [8]. We used GSK 2033 to estimate involvement of LXR agonistic activity in the induction of M1/M2 markers. 27OHChol increased levels of transcripts and surface expression of M2 markers of CD163 and CD206. Exposure to GSK 2033 resulted in suppressing the transcription of CD163 and CD206 genes and downregulating their surface levels (Fig. 4A and B). We also investigated effects of GSK 2033 on M1 markers of CD80 and CD86, along with CD14. The transcription of three genes elevated by 27OHChol was suppressed in the presence of GSK 2033. The LXR antagonist, however, did not downregulate surface expression of CD14, CD80, and CD86 (Fig. 5A and B). These results suggest that LXR activation is required for 27OHChol to induce transcription of the M1/M2 markers and that they are differentially regulated at protein level after LXR inhibition.
The LXRs are members of the nuclear receptor superfamily that modulate metabolism, development, proliferation and inflammation through positive and negative regulation of gene expression [13]. LXRs have crucial functions in the regulation of immune responses. Activation of LXR with agonists downregulates the expression of inflammatory genes through a process known as transrepression, and several studies have demonstrated the anti-inflammatory activities of synthetic LXR agonists using different mouse models of inflammatory diseases [19-23]. Endogenous oxysterols, like 27OHChol, are known to activate LXRs [12]. It is evident that this oxysterol is a pro-inflammatory molecule activating macrophages. Activated cells increase cytokine production and upregulate cell surface molecules involved in the immune response [7,9,10]. The current study investigated whether LXR-agonistic activity of 27OHChol affects the cytokine production and upregulates surface molecules after exposure to cell-active GSK 2033 [16], and thereby explored the biological functions of LXRs in macrophage activation.
27OHChol induces expression of diverse genes involved in lipid metabolism, inflammation, and cell differentiation [9,10,12]. Consistent with previous reports [12,14], we found that 27OHChol induces the LXR target genes of LXRα and ABCA1/ABCG1 via LXR-dependent mechanisms. Besides, we have demonstrated that the LXR agonistic activity is involved in expression of CCL3, CXCL8, CD14, CD80, CD86, CD163 and CD206, since their gene transcription is inhibited by GSK 2033. The significantly reduced expression of pattern recognition receptors, such as CD14 and CD206, following LXR inhibition, agrees with previous study that has demonstrated that LXR activation impacts the functioning of immune cells to establish an immune response against pathogens [24-26]. The downregulation of CD80 and CD86 is in line with a previous study by Torocsik et al. that reported increased expression of these molecules in monocyte-derived dendritic cells after administration of LXR agonists [27]. Taken together, our results suggest that the LXR-agonistic activity of 27OHChol is involved in the complex effects of this oxysterol on immune cells.
27OHChol elevates transcripts of the LXRα gene without a noticeable increase in its gene product. TO 901317, however, enhances its expression at both mRNA and protein levels, as shown in Fig. 1. The differences can be caused by the nature of LXR agonistic activity. 27OHChol is a natural LXR agonist, whereas TO 901317 is a synthetic one. TO 901317 displays a strong LXR agonistic activity and fully activates LXR signaling pathways [23]. In contrast, 27OHChol has a weak partial LXR agonistic activity [12]. Even though 27OHChol increases the level of LXRα transcripts, it seems that the activation of additional factors is required for translation of the mRNA into protein.
Cell surface molecules such as CD14, CD80, CD86, CD163, and CD206 are specific markers whose levels are enhanced by 27OHChol on monocytic cells [8]. Among them, CD163 and CD206 belong to M2 polarization markers, and CD80, and CD86 to M1 markers [28]. The results of Figs. 4B and 5B imply that expression of CD163 and CD206 protein is positively regulated by the LXR pathway while CD14, CD80, and CD86 are negatively regulated at protein level in the presence of 27OHChol. Collectively, these results suggest that LXR activity of 27OHChol is also involved in M2 polarization, which agrees with a previous study that reported that 27OHChol drives M2 polarization of human macrophages [29].
In summary, LXR inhibition with GSK 2033 leads to inhibited expression of CCL3 and CXCL8, but not of CCL2 and CCL4, enhanced TNF-α expression, and differential expression of M1/M2 markers on cell surface. We believe that the diverse effects of GSK 2033 are likely to be specific. GSK 2033 alone did not affect cell viability (Supplementary Fig. 2) and had no effect on the basal expression of the aforementioned genes. These results indicate that 27OHChol activates macrophages and affects immune cells via LXR-dependent and -independent mechanisms. Since 27OHChol induces inflammation and modifies immune responses by activating primarily monocytic cells, the molecular mechanisms underlying the cell type-specific effects of this oxysterol are still subject to further studies.
Supplementary data including two figures can be found with this article online at https://doi.org/10.4196/kjpp.2021.25.2.111.
ACKNOWLEDGEMENTS
This research was supported by the Basic Science Research Program through the National Research Foundation of Korea (NRF) funded by the Ministry of Education (NRF-2019R1I1A3A01055344).
Notes
REFERENCES
1. Schroepfer GJ Jr. 2000; Oxysterols: modulators of cholesterol metabolism and other processes. Physiol Rev. 80:361–554. DOI: 10.1152/physrev.2000.80.1.361. PMID: 10617772.


2. Brown AJ, Jessup W. 1999; Oxysterols and atherosclerosis. Atherosclerosis. 142:1–28. DOI: 10.1016/S0021-9150(98)00196-8. PMID: 9920502.


3. Iuliano L. 2011; Pathways of cholesterol oxidation via non-enzymatic mechanisms. Chem Phys Lipids. 164:457–468. DOI: 10.1016/j.chemphyslip.2011.06.006. PMID: 21703250.


4. Carpenter KL, Taylor SE, van der Veen C, Williamson BK, Ballantine JA, Mitchinson MJ. 1995; Lipids and oxidised lipids in human atherosclerotic lesions at different stages of development. Biochim Biophys Acta. 1256:141–150. DOI: 10.1016/0005-2760(94)00247-V. PMID: 7766691.


5. Garcia-Cruset S, Carpenter KL, Guardiola F, Stein BK, Mitchinson MJ. 2001; Oxysterol profiles of normal human arteries, fatty streaks and advanced lesions. Free Radic Res. 35:31–41. DOI: 10.1080/10715760100300571. PMID: 11697115.


6. Heo W, Kim SM, Eo SK, Rhim BY, Kim K. 2014; FSL-1, a Toll-like receptor 2/6 agonist, induces expression of interleukin-1α in the presence of 27-hydroxycholesterol. Korean J Physiol Pharmacol. 18:475–480. DOI: 10.4196/kjpp.2014.18.6.475. PMID: 25598661. PMCID: PMC4296036.



7. Kim SM, Lee SA, Kim BY, Bae SS, Eo SK, Kim K. 2013; 27-Hydroxycholesterol induces recruitment of monocytic cells by enhancing CCL2 production. Biochem Biophys Res Commun. 442:159–164. DOI: 10.1016/j.bbrc.2013.11.052. PMID: 24269812.


8. Lee J, Kim BY, Son Y, Giang DH, Lee D, Eo SK, Kim K. 2019; 4'-O-Methylalpinumisoflavone inhibits the activation of monocytes/macrophages to an immunostimulatory phenotype induced by 27-hydroxycholesterol. Int J Mol Med. 43:2177–2186. DOI: 10.3892/ijmm.2019.4135.

9. Son Y, Kim SM, Lee SA, Eo SK, Kim K. 2013; Oxysterols induce transition of monocytic cells to phenotypically mature dendritic cell-like cells. Biochem Biophys Res Commun. 438:161–168. DOI: 10.1016/j.bbrc.2013.07.046. PMID: 23876312.


10. Kim SM, Kim BY, Eo SK, Kim CD, Kim K. 2015; 27-Hydroxycholesterol up-regulates CD14 and predisposes monocytic cells to superproduction of CCL2 in response to lipopolysaccharide. Biochim Biophys Acta. 1852:442–450. DOI: 10.1016/j.bbadis.2014.12.003. PMID: 25497142.


11. Kim SM, Kim BY, Lee SA, Eo SK, Yun Y, Kim CD, Kim K. 2014; 27-Hydroxycholesterol and 7alpha-hydroxycholesterol trigger a sequence of events leading to migration of CCR5-expressing Th1 lymphocytes. Toxicol Appl Pharmacol. 274:462–470. DOI: 10.1016/j.taap.2013.12.007. PMID: 24370436.


12. Fu X, Menke JG, Chen Y, Zhou G, MacNaul KL, Wright SD, Sparrow CP, Lund EG. 2001; 27-hydroxycholesterol is an endogenous ligand for liver X receptor in cholesterol-loaded cells. J Biol Chem. 276:38378–38387. DOI: 10.1074/jbc.M105805200. PMID: 11504730.


13. Bookout AL, Jeong Y, Downes M, Yu RT, Evans RM, Mangelsdorf DJ. 2006; Anatomical profiling of nuclear receptor expression reveals a hierarchical transcriptional network. Cell. 126:789–799. DOI: 10.1016/j.cell.2006.06.049. PMID: 16923397. PMCID: PMC6211849.


14. Ignatova ID, Angdisen J, Moran E, Schulman IG. 2013; Differential regulation of gene expression by LXRs in response to macrophage cholesterol loading. Mol Endocrinol. 27:1036–1047. DOI: 10.1210/me.2013-1051. PMID: 23686114. PMCID: PMC3706843.



15. Willy PJ, Umesono K, Ong ES, Evans RM, Heyman RA, Mangelsdorf DJ. 1995; LXR, a nuclear receptor that defines a distinct retinoid response pathway. Genes Dev. 9:1033–1045. DOI: 10.1101/gad.9.9.1033. PMID: 7744246.


16. Zuercher WJ, Buckholz RG, Campobasso N, Collins JL, Galardi CM, Gampe RT, Hyatt SM, Merrihew SL, Moore JT, Oplinger JA, Reid PR, Spearing PK, Stanley TB, Stewart EL, Willson TM. 2010; Discovery of tertiary sulfonamides as potent liver X receptor antagonists. J Med Chem. 53:3412–3416. DOI: 10.1021/jm901797p. PMID: 20345102.


17. Kim SM, Jang H, Son Y, Lee SA, Bae SS, Park YC, Eo SK, Kim K. 2013; 27-hydroxycholesterol induces production of tumor necrosis factor-alpha from macrophages. Biochem Biophys Res Commun. 430:454–459. DOI: 10.1016/j.bbrc.2012.12.021. PMID: 23246833.


18. Hong C, Walczak R, Dhamko H, Bradley MN, Marathe C, Boyadjian R, Salazar JV, Tontonoz P. 2011; Constitutive activation of LXR in macrophages regulates metabolic and inflammatory gene expression: identification of ARL7 as a direct target. J Lipid Res. 52:531–539. DOI: 10.1194/jlr.M010686. PMID: 21187453. PMCID: PMC3035689.


19. Joseph SB, Castrillo A, Laffitte BA, Mangelsdorf DJ, Tontonoz P. 2003; Reciprocal regulation of inflammation and lipid metabolism by liver X receptors. Nat Med. 9:213–219. DOI: 10.1038/nm820. PMID: 12524534.


20. Fowler AJ, Sheu MY, Schmuth M, Kao J, Fluhr JW, Rhein L, Collins JL, Willson TM, Mangelsdorf DJ, Elias PM, Feingold KR. 2003; Liver X receptor activators display anti-inflammatory activity in irritant and allergic contact dermatitis models: liver-X-receptor-specific inhibition of inflammation and primary cytokine production. J Invest Dermatol. 120:246–255. DOI: 10.1046/j.1523-1747.2003.12033.x. PMID: 12542530.


21. Heine G, Dahten A, Hilt K, Ernst D, Milovanovic M, Hartmann B, Worm M. 2009; Liver X receptors control IgE expression in B cells. J Immunol. 182:5276–5282. DOI: 10.4049/jimmunol.0801804. PMID: 19380774.


22. Park MC, Kwon YJ, Chung SJ, Park YB, Lee SK. 2010; Liver X receptor agonist prevents the evolution of collagen-induced arthritis in mice. Rheumatology (Oxford). 49:882–890. DOI: 10.1093/rheumatology/keq007. PMID: 20159908.


23. Terasaka N, Hiroshima A, Koieyama T, Ubukata N, Morikawa Y, Nakai D, Inaba T. 2003; T-0901317, a synthetic liver X receptor ligand, inhibits development of atherosclerosis in LDL receptor-deficient mice. FEBS Lett. 536:6–11. DOI: 10.1016/S0014-5793(02)03578-0. PMID: 12586329.


24. Bruhn KW, Marathe C, Maretti-Mira AC, Nguyen H, Haskell J, Tran TA, Vanchinathan V, Gaur U, Wilson ME, Tontonoz P, Craft N. 2010; LXR deficiency confers increased protection against visceral Leishmania infection in mice. PLoS Negl Trop Dis. 4:e886. DOI: 10.1371/journal.pntd.0000886. PMID: 21103366. PMCID: PMC2982826.


25. Korf H, Vander Beken S, Romano M, Steffensen KR, Stijlemans B, Gustafsson JA, Grooten J, Huygen K. 2009; Liver X receptors contribute to the protective immune response against Mycobacterium tuberculosis in mice. J Clin Invest. 119:1626–1637. DOI: 10.1172/JCI35288. PMID: 19436111. PMCID: PMC2689129.



26. Joseph SB, Bradley MN, Castrillo A, Bruhn KW, Mak PA, Pei L, Hogenesch J, O'connell RM, Cheng G, Saez E, Miller JF, Tontonoz P. 2004; LXR-dependent gene expression is important for macrophage survival and the innate immune response. Cell. 119:299–309. DOI: 10.1016/j.cell.2004.09.032. PMID: 15479645.


27. Töröcsik D, Baráth M, Benko S, Széles L, Dezso B, Póliska S, Hegyi Z, Homolya L, Szatmári I, Lányi A, Nagy L. 2010; Activation of liver X receptor sensitizes human dendritic cells to inflammatory stimuli. J Immunol. 184:5456–5465. DOI: 10.4049/jimmunol.0902399. PMID: 20410489.


28. Zhou Y, Yoshida S, Kubo Y, Yoshimura T, Kobayashi Y, Nakama T, Yamaguchi M, Ishikawa K, Oshima Y, Ishibashi T. 2017; Different distributions of M1 and M2 macrophages in a mouse model of laser-induced choroidal neovascularization. Mol Med Rep. 15:3949–3956. DOI: 10.3892/mmr.2017.6491. PMID: 28440413. PMCID: PMC5436148.



29. Marengo B, Bellora F, Ricciarelli R, De Ciucis C, Furfaro A, Leardi R, Colla R, Pacini D, Traverso N, Moretta A, Pronzato MA, Bottino C, Domenicotti C. 2016; Oxysterol mixture and, in particular, 27-hydroxycholesterol drive M2 polarization of human macrophages. Biofactors. 42:80–92. DOI: 10.1002/biof.1243. PMID: 26669587.

Fig. 1
Inhibitory effects of GSK 2033 on the transcription and protein translation of the LXRα and ABCA1 genes.
THP-1 cells were serum-starved overnight and treated with GSK 2033 (1 μM) for 2 h. Cells were further stimulated with 27OHChol (2.5 μg/ml) or TO 901317 (1 μM) for 48 h in RPMI media supplemented with 10% FBS. The levels of LXRα (A) or ABCA1 (B) gene transcripts were assessed by real-time PCR. Data are expressed as the means ± standard deviation (n = 3 replicates for each group). LXRs, liver X receptors; FBS, fetal bovine serum. ***p < 0.001 vs. control; ###p < 0.001 vs. 27OHChol or TO 901317; #p < 0.05 vs. 27OHChol or TO 901317. (C) LXRα and ABCA1 proteins were detected by Western blot analysis. Data represent a representative experiment (from 3 independent experiments).

Fig. 2
Differential effects of GSK 2033 on 27OHChol-induced expression of C-C chemokines.
After serum-starvation overnight, THP-1 cells were treated with GSK 2033 (1 μM) for 2 h and stimulated with 27OHChol (2.5 μg/ml) for 48 h in RPMI media supplemented with 10% FBS. (A) Levels of CCL2, CCL3, and CCL4 gene transcripts were assessed by real-time PCR. (B) The amounts of CCL2, CCL3, and CC4 proteins secreted in culture media were quantified by ELISA. Data are expressed as the means ± standard deviation (n = 3 replicates for each group). The results are representative of 3 independent experiments. FBS, fetal bovine serum. ***p < 0.001 vs. control; *p < 0.05 vs. control; ###p < 0.001 vs. 27OHChol.

Fig. 3
Opposite effects of GSK 2033 on CXCL8 and TNF-α expression.
Serum-starved THP-1 cells were treated with GSK 2033 (1 μM) for 2 h and stimulated for 48 h with 27OHChol (2.5 μg/ml). (A) Transcript levels of the CXCL8 and TNF-α genes were assessed by real-time PCR. (B) The amounts of CXCL8 and TNF-α proteins secreted in culture media were quantified by ELISA. Data are expressed as the means ± standard deviation (n = 3 replicates for each group). The results are representative of 3 independent experiments. The results are representative of 3 independent experiments. ***p < 0.001 vs. control; ###p < 0.001 vs. 27OHChol.

Fig. 4
Suppressive effects of GSK 2033 on M2 surface makers.
Following serum-starvation, THP-1 cells were treated with GSK 2033 (1 μM) for 2 h and stimulated with 27OHChol (2.5 μg/ml) for 48 h. (A) Transcript levels of the DC163 and CD206 genes were assessed by real-time PCR. Data are expressed as the means ± standard deviation (n = 3 replicates for each group). (B) After immunostaining of CD163 and CD206 with fluorophore-conjugated Abs, cells were analyzed by flow cytometry. The results are representative of 3 independent experiments. ***p < 0.001 vs. control; ### p < 0.001 vs. 27OHChol.

Fig. 5
Differential effects of GSK 2033 on expression of M1 surface markers.
Serum-starved THP-1 cells were treated with GSK 2033 (1 μM) for 2 h, followed by stimulation with 27OHChol (2.5 μg/ml) for 48 h. (A) Transcript levels of the CD14, CD80, and CD86 genes were assessed by real-time PCR. Data are expressed as the means ± standard deviation (n = 3 replicates for each group). (B) After immunostaining of CD14, CD80, and CD86 with fluorophore-conjugated Abs, cells were analyzed by flow cytometry. The results are representative of 3 independent experiments. ***p < 0.001 vs. control; **p < 0.01 vs. control; *p < 0.05 vs. control; ###p < 0.001 vs. 27OHChol; #p < 0.05 vs. 27OHChol.
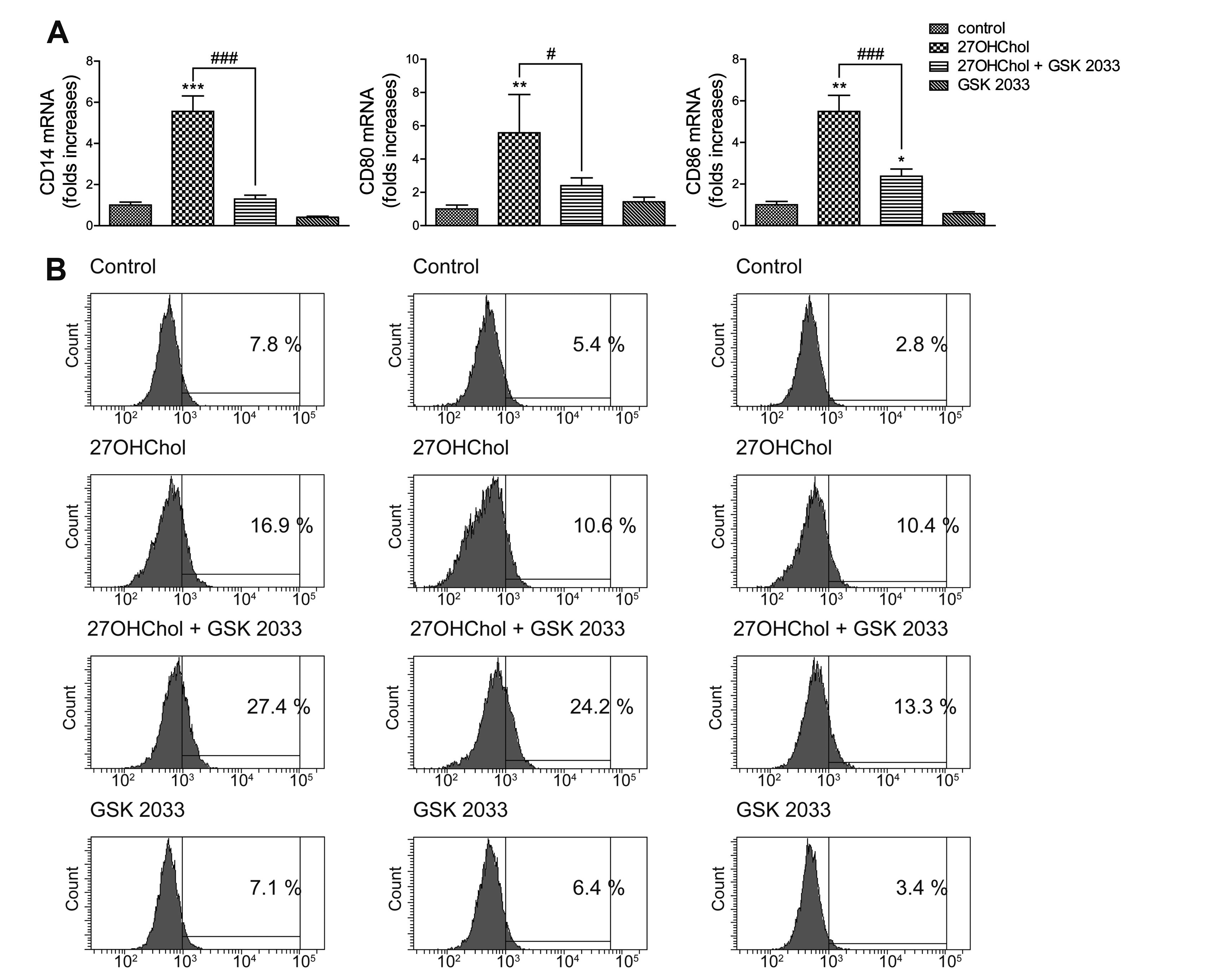
Table 1
List of primers used in this study