Abstract
Background
C-reactive protein (CRP) test can be used at coronavirus disease 2019 (COVID-19) screening clinics as a point of care test. The aim of this study was to determine whether CRP test can be used as an indicator of lung injury.
Methods
In all, 98 patients with COVID-19 were enrolled for this study. We collected medical records and laboratory results of the patients. Chest radiographic findings were classified into five groups. We analyzed the correlation between laboratory tests and the grade of lung injury.
Results
In patients with COVID-19, increased CRP and erythrocyte sedimentation rate (ESR), and lymphocytopenia were noted. CRP, ESR, and segmented neutrophils were found to be positively correlated while albumin and lymphocyte were negatively correlated with lung injury grade. CRP levels showed the highest correlation coefficient of 0.858. Based on the chest radiographs, the sensitivity and specificity of CRP was 100% and 77.8%, respectively. Within the reference range of CRP, abnormal chest radiograph was not recognized. In addition, initial and subsequent increase in CRP levels in patients with unremarkable chest radiograph could be associated with aggravated lung injury.
초록
배경
C-반응단백질(C-reactive protein, CRP) 검사는 COVID-19 선별진료소에서 현장검사로도 사용할 수 있다. 이 연구에서는 CRP 검사가 COVID-19환자의 폐 손상을 예측하는 지표로 사용될 수 있는지 여부를 확인하고자 하였다.
방법
총 98명의 COVID-19 환자의 의무기록과 검사실 및 영상의학적 검사결과를 수집하였다. 흉부 방사선 소견은 5개의 그룹으로 분류하여 검사실 검사와 폐 손상 등급 간의 상관관계를 분석하였다.
결과
COVID-19 환자에서 증가된 CRP와 ESR 및 림프구감소증이 관찰되었다. 폐 손상 등급에 따라 CRP, ESR 및 호중구 수는 양의 상관관계를 보인 반면, 알부민과 림프구 수는 음의 상관관계를 보였다. CRP가 폐 손상 정도와 가장 높은 상관계수(r=0.858)를 보였으며, 흉부 방사선 사진 결과와 비교하여 CRP 검사는 100%의 민감도와 77.8%의 특이도를 보였다. CRP가 참고 범위 내에 있는 환자에서는 비정상적인 흉부 방사선 소견은 확인되지 않았다. 초기 흉부 방사선 상 특이 소견이 없었으나 이후 비정상 소견이 나타난 환자에서 초기 및 연속적으로 측정한 CRP값이 높았다.
On March 11, 2020, WHO characterized the new coronavirus outbreak as a pandemic. Coronavirus disease 2019 (COVID-19) has spread in more than 213 countries worldwide, and the total number of the patients has exceeded 10,000,000 as of July 2, 2020 (https://covid19.who.int/).
The largest number of COVID-19 patients in South Korea (N=6,833) was recorded in Daegu, where the spread of the disease was caused by a specific religious group called Sincheonji (https://coronaboard.kr/). The Daegu Catholic University Medical Center (DCUMC) had 98 confirmed COVID-19 patients as of March 10, 2020.
The lung is the main target organ of severe acute respiratory syndrome coronavirus 2 (SARS-CoV-2) infection [1, 2]; therefore, it is essential to perform radiographical examination for lung injury. To detect lung injury, chest computed tomography (CT) is more accurate than chest radiograph, but chest CT is not available for screening of large number of people. Though the chest radiograph could be utilized for mass screening, it is difficult to obtain in a short time in an emergency clinic.
In this study, we compared the C-reactive protein (CRP) test levels with chest radiograph findings. Many patients visit COVID-19 screening clinics, but many are required to wait in their own home due to lack of hospitalization. To determine admission, doctors refer to patient’s temperature and clinical symptoms, but they are not an objective criterion. CRP test can be used at screening clinics as a point of care test [3]. If CRP test is correlated with the chest radiograph findings, it can be used as a criterion for determining admission of patients with COVID-19. Some studies have identified objective markers for assessing the severity of disease in COVID-19 patients [4-8]. In previously reported meta-analyses [9, 10], CRP, albumin, erythrocyte sedimentation rate (ESR), eosinopenia, interleukin-6, and neutrophil-to-lymphocyte ratio were the most prevalent laboratory findings. Among those markers, CRP was most frequently described [4-6]. Studies on CRP levels and extent of lung injury were conducted by several researchers [4, 8, 11], but the number of cases in their studies were small (12 to 27 cases) and they did not focus on the clinical usefulness of CRP. Here, we intended to clarify the clinical significance of CRP through detailed analysis and follow-up of CRP levels and lung injury.
In all, 98 patients were enrolled for this study, from which, 79 patients were diagnosed at other hospitals and were transferred to the DCUMC (Daegu, Korea). The remaining 19 patients were diagnosed and admitted at the DCUMC. Reverse-transcription polymerase chain reaction (RT-PCR) for COVID-19 was conducted using upper and/or lower respiratory specimens at DCUMC using Powerchek™ 2019-nCoV Real-time PCR kit (Kogene Biotech, Seoul, Korea). PCR was performed with a CFX96 real-time PCR detection system (Bio-Rad, Hercules, CA, USA). We collected medical records of the patients from February to March 2020 using electric medical records of DCUMC. The chest radiograph findings were read by two radiologists. They classified the chest radiographic findings into five groups according to arbitrary criteria based on the extent of infiltration as follows: unremarkable finding (grade 0), less than 25% of total lung volume (grade 1), 25 to 50% of total lung volume (grade 2), 50 to 75% of total lung volume (grade 3), and greater than 75% of total lung volume (grade 4) (Table 1 and Fig. 1). As only one case belonged to grade 4, it was reclassified as grade 3 for statistical analysis. We used the UniCel DxH 800 (Beckman Coulter, Brea, CA, USA) for hematology tests, cobas c 702 (Roche Diagnostics, Rotkreuz, Switzerland) for biochemical tests, and Test1 (AliFax, Padova, Italy) for ESR tests. This study was approved by the Institutional Review Board (IRB) of DCUMC (CR-20-051-PRO-001-R).
Statistical analysis was performed using IBM SPSS 19.0. Spearman rank correlation analysis was used for determining the association between laboratory results and lung injury grade. Kruskal Wallis test was used for determining the significance of CRP levels according to the lung injury grade. Repeated measurement ANOVA was performed to compare the difference in serial CRP values between patients with unchanged (grade 0) and aggravated (grade 1-4) chest radiographic findings monitored for 14 days.
The average age and M:F ratio among the 98 patients were 56.8±18.9 years and 0.53:1, respectively. The average number of days between COVID-19 diagnosis and initial blood sample collection following hospitalization was 4.3±1.9 days. Cough appeared most frequently (59.2%), followed by sputum (44.9%), fever (42.9%), and dyspnea (41.8%). In the laboratory results, increased CRP and ESR, and lymphocytopenia were noted. Fifty-four patients (55.1%) showed no active pulmonary lesions on chest radiograph (Table 1). Fig. 1 provides typical chest radiographs by the lung injury grade. There was no evidence of bacterial superinfection in any patient.
We calculated correlation coefficients between chest radiograph findings and laboratory tests (Table 1). The blood collection and laboratory tests were performed within 0-1 day of chest imaging for all patients. CRP, ESR and segmented neutrophils showed a positive correlation while albumin and lymphocyte showed a negative correlation with lung injury grade. The results revealed that CRP levels had the highest correlation coefficient of 0.858.
Based on the chest radiographs, CRP test showed 100% sensitivity and 77.8% specificity (Table 2). In the group with normal CRP (≤5 mg/L), all chest radiographs were unremarkable, and in the group with increased CRP (>5 mg/L), abnormalities were observed in 78.6% of chest radiographs. In groups with CRP in the range of 5 to 20 and 20 to 50 mg/dL, abnormalities were observed in 47.4% and 89.5% of chest radiographs, respectively, while in the group with over 50 mg/dL CRP, all patients had abnormal chest radiograph (Table 2). The distribution of CRP concentration by the lung injury grade is shown in Fig. 2. CRP levels were significantly different according to the lung injury grade. In each group of injury grade, CRP showed high odds ratio and odds ratio increased in proportion to the CRP level (Table 3).
We analyzed serial CRP values on days 1, 4, 7, 10, and 14 in the 54 patients with unremarkable chest radiograph on admission (grade 0). Forty-three patients had no change in chest radiograph and 11 had aggravated chest findings for 14 days. The serial CRP values between the unchanged and aggravated groups were significantly different (P<0.001, Fig. 3). Among 12 patients who showed increased CRP concentration with unremarkable chest radiograph, 11 patients had abnormal chest radiograph or chest CT findings after 4 to 12 days (Table 4).
In this study, increased CRP and ESR, and lymphopenia were noted in COVID-19 patients. CRP, ESR and segmented neutrophils showed a positive correlation while albumin and lymphocyte showed a negative correlation with lung injury grade. CRP test showed the highest correlation (r=0.858), 100% sensitivity, and 77.8% specificity compared to the chest radiograph findings. Abnormal chest radiograph was not detected in the reference range of CRP. In addition, initial and subsequent increase in CRP levels in patients with unremarkable chest radiograph could be associated with aggravated lung injury. CRP is one of the strongest acute phase reactants and is increased in various infections and inflammatory diseases [12]. CRP aids in non-specific host defense against infectious organisms by activating the classical complement pathway [13]. Concentration of CRP is generally higher in bacterial infection than in viral infection [14]. CRP levels increase within 6 to 12 hours after the onset of inflammation or injury and peak at about 48 hours and they are generally proportional to the extent of tissue damage [12].
Zhao et al. [15] found that angiotensin-converting enzyme 2 (ACE2) is the receptor for SARS-CoV-2. In the human lung, ACE2 is expressed on type I and II alveolar epithelial cells. Among them, 83% of the type II alveolar cells have express ACE2 [15]. The binding of SARS-CoV-2 on ACE2 causes an elevated expression of ACE2, which can lead to damage to alveolar cells. Damage to alveolar cells can trigger a series of systemic reactions and even death. About a third of patients presented with acute respiratory distress syndrome, showing desquamation of pneumocytes and hyaline membrane formation [16, 17]. Abnormalities in chest CT images were found in nearly 100% of the patients [1, 18]. Therefore, it is important to find an objective marker that can predict the extent of lung injury.
In this study, the positive rates of initial symptoms (fever 42.9% and cough 59.2%) were not high as in other studies [1, 19]. Patient’s symptoms are important in the diagnosis of COVID-19 but are difficult to use to predict lung injury. In the correlation analysis, CRP showed the highest correlation (r=0.858) with the lung injury grade followed by ESR (r=0.796). Lymphocytopenia was commonly detected [17, 20], suggesting that COVID-19 might act on lymphocytes, especially T lymphocytes [19]. The mechanism of lymphopenia includes direct infection and destruction of lymphocytes [21] and cytokine mediated lymphocyte destruction [22]. In other studies, the correlation coefficient between CRP levels and extent of lung injury was 0.59 [11], 0.62 [4], and 0.87 [8]. CRP may be the best indicator of lung injury compared to other laboratory parameters. In a study by Liu et al. [11], albumin and lymphocytes were more correlated with lung injury compared to CRP. Generally, serum albumin concentration is influenced by age (r=-0.504, this study, data not shown); hence, the direct association between albumin and lung injury will not be high. All those who showed abnormal chest radiograph had increased CRP levels. In the group of patients with normal CRP levels, none of the patients had active pulmonary lesions on the chest radiograph. As chest radiograph is less sensitive compared to chest CT, normal CRP levels cannot be used to confirm no lung injury. In the groups of patients with increased CRP levels, CRP levels were proportional to the positive rate and degree of lung injury. Compared to the other studies that analyzed the association between lung injury and CRP [4, 8, 11], this study showed the highest correlation and odds ratio. We also obtained high sensitivity and specificity with the lowest cut-off value.
Among 54 patients of grade 0, the group with aggravated chest radiographic findings showed higher initial and subsequent CRP levels than the group with unchanged chest radiographic findings. Initial and subsequent increase in CRP levels was associated with aggravated lung injury. For the 12 patients with unremarkable chest radiographs and increased CRP levels, we followed up with CRP test and chest radiograph. Except one patient (Case 1), all patients had lung injury findings while two of them (Case 7 & 10) showed normal CRP levels with multiple ground glass opacity lesion by chest CT on follow up test. This discrepancy might be explained by short half-life of CRP (4 to 7 hours) [12] and long resolution time of ground glass opacity (5 to 10 days) [18, 23]. CRP levels have been routinely used in clinical laboratories to detect or monitor inflammatory status of the patients. Though CRP is not lung specific, its application as lung injury marker would be especially useful for initial classification and follow-up of COVID-19 patients.
Limitations of this study were limited number of enrolled cases and less sensitivity of chest radiograph compared to chest CT. Though the number of patients was not high, the results of this study were statistically significant. Chest CT examination is considered superior to chest radiograph for determining the degree of lung injury, but chest CT examination is expensive and not readily available in many hospitals. In the future, we will study the correlation between chest CT findings and CRP levels.
In conclusion, CRP test showed a sensitivity of 100% in detecting lung injury, and the level was proportional to the extent of lung lesion. Therefore, CRP test will be useful as a predictor of lung injury in patients with COVID-19.
Acknowledgements
This study was supported by a research grant from Daegu Medical Association COVID19 Scientific Committee.
REFERENCES
1. Huang C, Wang Y, Li X, Ren L, Zhao J, Hu Y, et al. 2020; Clinical features of patients infected with 2019 novel coronavirus in Wuhan, China. Lancet. 395:497–506. DOI: 10.1016/S0140-6736(20)30183-5. PMID: 31986264. PMCID: PMC7159299.



2. Rothan HA, Byrareddy SN. 2020; The epidemiology and pathogenesis of coronavirus disease (COVID-19) outbreak. J Autoimmun. 109:102433. DOI: 10.1016/j.jaut.2020.102433. PMID: 32113704. PMCID: PMC7127067.


3. Matheeussen V, Van Hoof V, Loens K, Lammens C, Vanderstraeten A, Coenen S, et al. 2018; Analytical performance of a platform for point-of-care CRP testing in adults consulting for lower respiratory tract infection in primary care. Eur J Clin Microbiol Infect Dis. 37:1319–23. DOI: 10.1007/s10096-018-3253-3. PMID: 29744764.


4. Tan C, Huang Y, Shi F, Tan K, Ma Q, Chen Y, et al. 2020; C-reactive protein correlates with computed tomographic findings and predicts severe COVID-19 early. J Med Virol. 92:856–62. DOI: 10.1002/jmv.25871. PMID: 32281668. PMCID: PMC7262341.


5. Chen W, Zheng KI, Liu S, Yan Z, Xu C, Qiao Z. 2020; Plasma CRP level is positively associated with the severity of COVID-19. Ann Clin Microbiol Antimicrob. 19:18. DOI: 10.1186/s12941-020-00362-2. PMID: 32414383. PMCID: PMC7227180.



6. Bhargava A, Fukushima EA, Levine M, Zhao W, Tanveer F, Szpunar SM, et al. 2020; Predictors for severe COVID-19 infection. Clin Infect Dis. 71:1962–8. DOI: 10.1093/cid/ciaa674. PMID: 32472676. PMCID: PMC7314166.


7. Shang W, Dong J, Ren Y, Tian M, Li W, Hu J, et al. 2020; The value of clinical parameters in predicting the severity of COVID-19. J Med Virol. 10:2188–92. DOI: 10.1002/jmv.26031. PMID: 32436996. PMCID: PMC7280691.


8. Wang L. 2020; C-reactive protein levels in the early stage of COVID-19. Med Mal Infect. 50:332–4. DOI: 10.1016/j.medmal.2020.03.007. PMID: 32243911. PMCID: PMC7146693.


9. Zeng F, Huang Y, Guo Y, Yin M, Chen X, Xiao L, et al. 2020; Association of inflammatory markers with the severity of COVID-19: A meta-analysis. Int J Infect Dis. 96:467–74. DOI: 10.1016/j.ijid.2020.05.055. PMID: 32425643. PMCID: PMC7233226.



10. Zhang ZL, Hou YL, Li DT, Li FZ. 2020; Laboratory findings of COVID-19: a systematic review and meta-analysis. Scand J Clin Lab Invest. 80:441–7. DOI: 10.1080/00365513.2020.1768587. PMID: 32449374. PMCID: PMC7256350.


11. Liu Y, Yang Y, Zhang C, Huang F, Wang F, Yuan J, et al. 2020; Clinical and biochemical indexes from 2019-nCoV infected patients linked to viral loads and lung injury. Sci China Life Sci. 63:364–74. DOI: 10.1007/s11427-020-1643-8. PMID: 32048163. PMCID: PMC7088566.



12. Litao MK, Kamat D. 2014; Erythrocyte sedimentation rate and C-reactive protein: how best to use them in clinical practice. Pediatr Ann. 43:417–20. DOI: 10.3928/00904481-20140924-10. PMID: 25290132.


13. Sproston NR, Ashworth JJ. 2018; Role of C-reactive protein at sites of inflammation and infection. Front Immunol. 9:754. DOI: 10.3389/fimmu.2018.00754. PMID: 29706967. PMCID: PMC5908901.



14. Korppi M, Kröger L. 1993; C-reactive protein in viral and bacterial respiratory infection in children. Scand J Infect Dis. 25:207–13. DOI: 10.3109/00365549309008486. PMID: 8511515.


15. Zhao Y, Zhao Z, Wang Y, Zhou Y, Ma Y, Zuo W. 2020; Single-cell RNA expression profiling of ACE2, the receptor of SARS-CoV-2. Am J Respir Crit Care Med. 202:756–9. DOI: 10.1164/rccm.202001-0179LE. PMID: 32663409. PMCID: PMC7462411.



16. Xu Z, Shi L, Wang Y, Zhang J, Huang L, Zhang C, et al. 2020; Pathological findings of COVID-19 associated with acute respiratory distress syndrome. Lancet Respir Med. 8:420–2. DOI: 10.1016/S2213-2600(20)30076-X. PMID: 32085846. PMCID: PMC7164771.



17. Rodriguez-Morales AJ, Cardona-Ospina JA, Gutiérrez-Ocampo E, Villamizar-Peña R, Holguin-Rivera Y, Escalera-Antezana JP, et al. 2020; Clinical, laboratory and imaging features of COVID-19: A systematic review and meta-analysis. Travel Med Infect Dis. 34:101623. DOI: 10.1016/j.tmaid.2020.101623. PMID: 32179124. PMCID: PMC7102608.


18. Song F, Shi N, Shan F, Zhang Z, Shen J, Lu H, et al. 2020; Emerging 2019 novel coronavirus (2019-nCoV) pneumonia. Radiology. 295:210–7. DOI: 10.1148/radiol.2020200274. PMID: 32027573. PMCID: PMC7233366.


19. Li LQ, Huang T, Wang YQ, Wang ZP, Liang Y, Huang TB, et al. 2020; COVID-19 patients' clinical characteristics, discharge rate, and fatality rate of meta-analysis. J Med Virol. 92:577–83. DOI: 10.1002/jmv.25757. PMID: 32162702. PMCID: PMC7228329.


20. Tian S, Hu N, Lou J, Chen K, Kang X, Xiang Z, et al. 2020; Characteristics of COVID-19 infection in Beijing. J Infect. 80:401–6. DOI: 10.1016/j.jinf.2020.02.018. PMID: 32112886. PMCID: PMC7102527.



21. Zheng HY, Zhang M, Yang CX, Zhang N, Wang XC, Yang XP, et al. 2020; Elevated exhaustion levels and reduced functional diversity of T cells in peripheral blood may predict severe progression in COVID-19 patients. Cell Mol Immunol. 17:541–3. DOI: 10.1038/s41423-020-0401-3. PMID: 32203186. PMCID: PMC7091621.



22. Zheng M, Gao Y, Wang G, Song G, Liu S, Sun D, et al. 2020; Functional exhaustion of antiviral lymphocytes in COVID-19 patients. Cell Mol Immunol. 17:533–5. DOI: 10.1038/s41423-020-0402-2. PMID: 32203188. PMCID: PMC7091858.


23. Chen N, Zhou M, Dong X, Qu J, Gong F, Han Y, et al. 2020; Epidemiological and clinical characteristics of 99 cases of 2019 novel coronavirus pneumonia in Wuhan, China: a descriptive study. Lancet. 395:507–13. DOI: 10.1016/S0140-6736(20)30211-7. PMID: 32007143. PMCID: PMC7135076.


Fig. 1
(A) Patchy consolidations in both upper lung fields (Grade 1). The lesion was improved, and the patient was discharged without sequelae 17 days later (CRP: 19.5 mg/L). (B) Patchy consolidations in the right lower lung and left middle and lower lung fields (Grade 2). The lesion was improved, and the patient was discharged without sequelae 1 month later (CRP: 44.7 mg/L). (C) Peripherally located consolidations in both lung fields (Grade 3). The patient was discharged without sequelae 1 month later (CRP: 120 mg/L). (D) Diffuse consolidations in both lung fields (Grade 4). The patient died of acute respiratory distress syndrome 10 days later despite aggressive antiviral therapy (CRP: 79.5 mg/L).
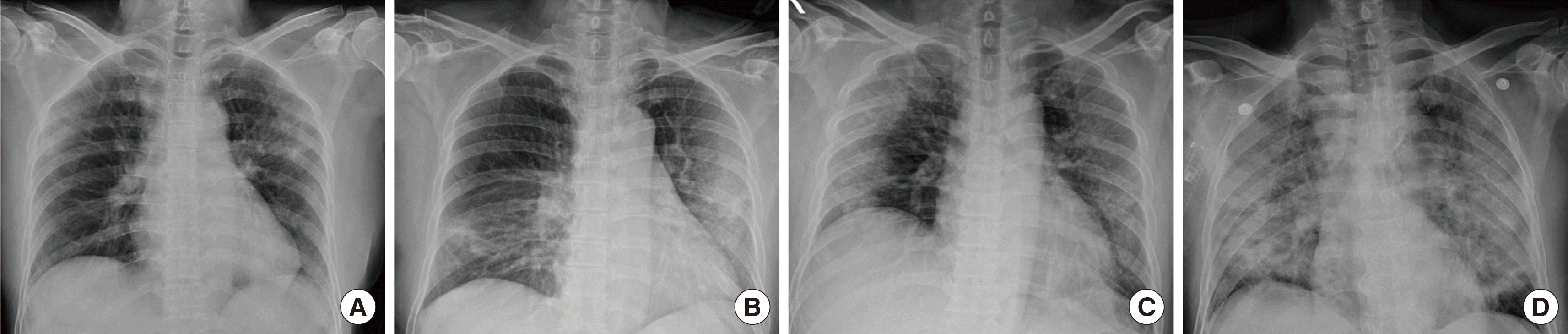
Fig. 2
Distribution of CRP levels according to the lung injury grade. Kruskal Wallis test was used for determining the significance of CRP levels according to the lung injury grade.
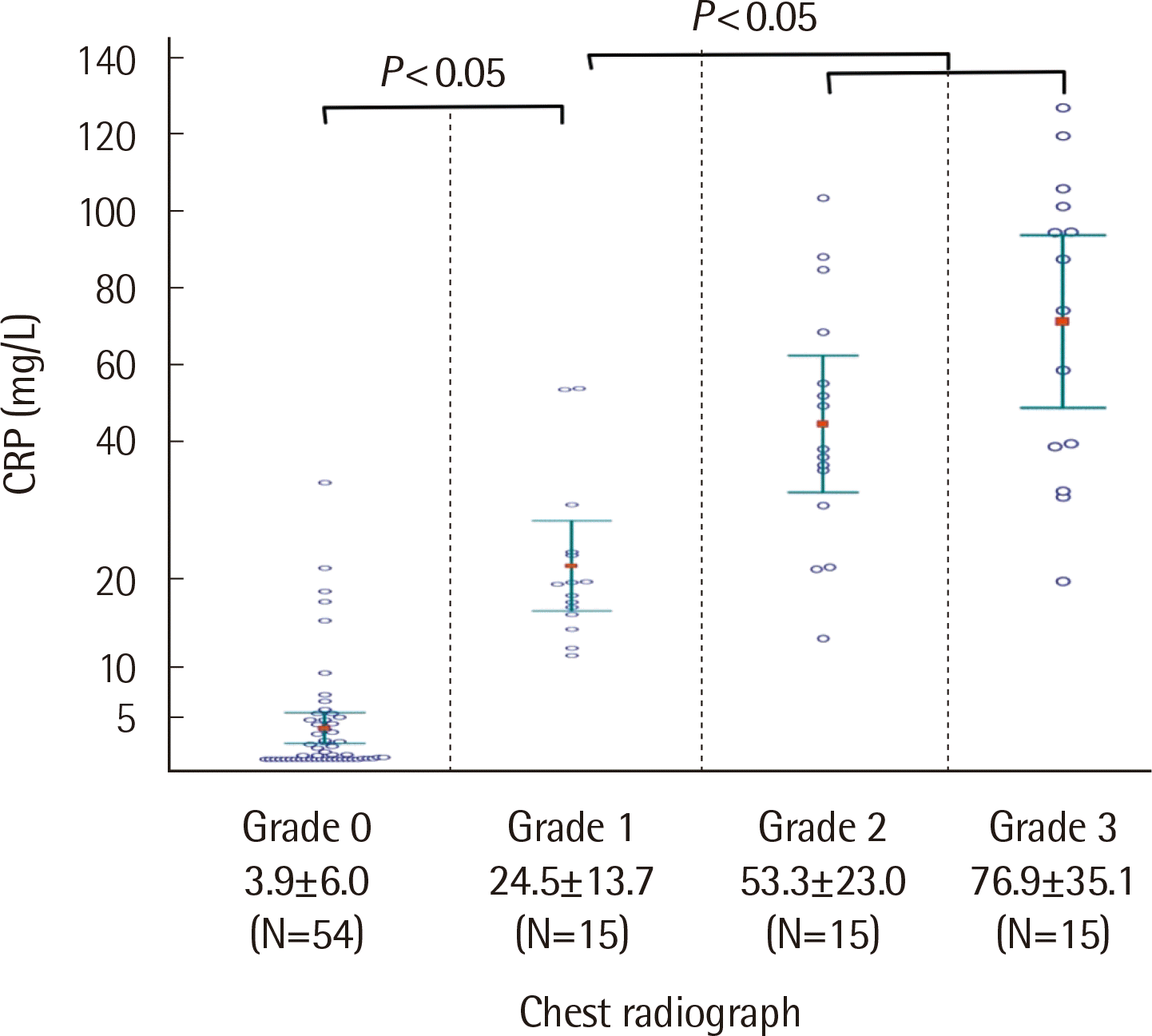
Fig. 3
Comparison of the serial CRP values between patients with unchanged (grade 0) and aggravated (grade 1-4) chest radiographic (CXR) findings monitored for 14 days.
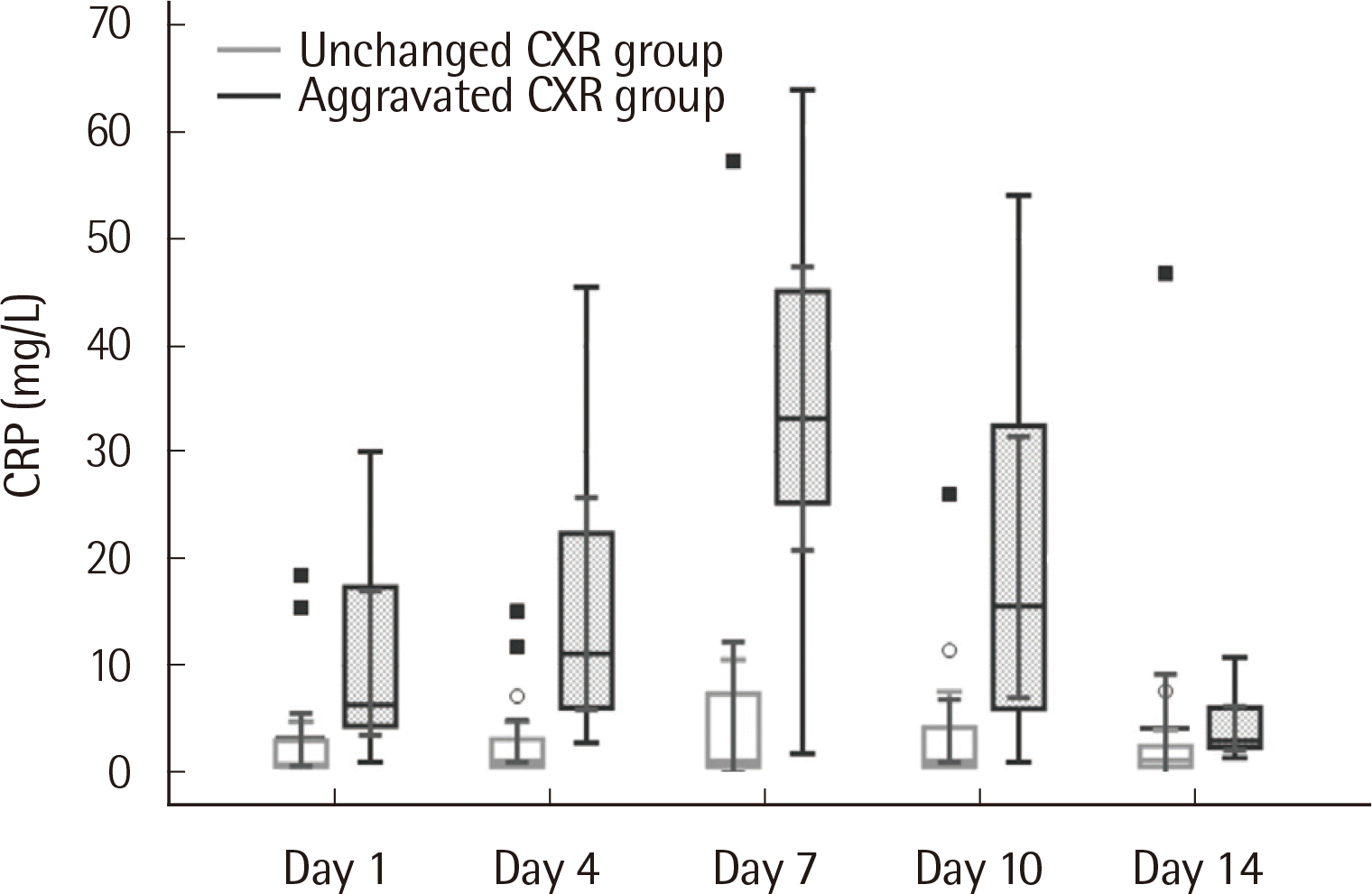
Table 1
Demographic and clinical characteristics of and correlation with lung injury grade in patients with COVID-19 (N=98)
Characteristics | Data | Correlation coefficient |
---|---|---|
Age (yr) | 56.8±18.9 | |
M:F ratio | 0.53:1 | |
Clinical symptoms | ||
Fever | 42 (42.9) | NA |
Chill | 25 (25.5) | NA |
Cough | 58 (59.2) | NA |
Sputum | 44 (44.9) | NA |
Sore throat | 18 (18.4) | NA |
Dyspnea | 41 (41.8) | NA |
Cheat pain | 12 (12.2) | NA |
Headache | 29 (29.6) | NA |
Laboratory results (Reference range) | ||
CRP (≤5 mg/L) | 25.0±32.4 | 0.858* |
Albumin (3.5–5.1 g/dL) | 3.9±4.4 | -0.631* |
Glucose (74–100 mg/dL) | 119.8±57.2 | 0.448* |
ALT (<40 U/L) | 28.2±27.7 | 0.325* |
eGFR (>90 mL/min/1.7 m2)† | 90.2±24.5 | -0.366* |
Hemoglobin (M12.9–16.9, F10.7–14.6 g/dL) | 128±18.0 | -0.130 |
WBC (3.6–9.6×109/L) | 5.52±2.67 | 0.245 |
Segmented WBC (×109/L) | 3.58±2.52 | 0.456* |
Lymphocyte (×109/L) | 1.33±0.56 | -0.572* |
Monocyte (×109/L) | 0.50±0.27 | 0.060 |
ESR (M≤10, F≤20 mm/hr) | 45.5±30.8 | 0.796* |
Chest radiograph grades‡ | ||
Grade 0 (No) | 54 (55.1) | NA |
Grade 1 (<25%) | 15 (15.3) | NA |
Grade 2 (25–50%) | 15 (15.3) | NA |
Grade 3 (50–75%) | 13 (13.3) | NA |
Grade 4 (>75%) | 1 (1.0) | NA |
Table 2
Diagnostic values of CRP test for lung injury according to CRP level
CRP (mg/L) | Chest PA | |
|
||
Abnormal* | Unremarkable | |
> 5 (N = 56) | 44 | 12 |
≤ 5 (N = 42) | 0 | 42 |
Total (N = 98) | 44 | 54 |
Sensitivity 100% | Specificity 77.8% | |
PPV 78.6% | NPV 100% | |
CRP (mg/L) | Abnormal rates of chest PA in the increased CRP group | |
|
||
Abnormal chest PA | ||
5–20 (N = 19) | 9 (47.4%) | |
20–50 (N = 19) | 17 (89.5%) | |
> 50 (N = 18) | 18 (100%) |
Table 3
Multinomial logistic regression for CRP according to the chest injury grade
Chest injury grade (Reference: Grade 0) | CRP (mg/L) | OR | 95% Confidence Interval for OR | P-value |
---|---|---|---|---|
Grade 1 | < 5 | 1 | - | - |
5–20 | 58.8 | 3.075–1124.407 | 0.007* | |
20–50 | 252 | 10.120–6275.150 | 0.001* | |
> 50 | 336 | 5.198–21717.724 | 0.006* | |
Grade 2 | < 5 | 1 | - | - |
5–20 | 8.4 | 0.263–268.378 | 0.229 | |
20–50 | 294 | 11.976–7217.373 | 0.001* | |
> 50 | 1,176 | 21.521–64260.947 | 0.001* | |
Grade 3 | < 5 | 1 | - | - |
5–20 | 8.4 | 0.263–268.378 | 0.229 | |
20–50 | 168 | 6.421–4395.324 | 0.002* | |
> 50 | 1,512 | 28.096–81369.142 | 0.000* |
Table 4
Follow-up test results for 12 patients with increased CRP (mg/L) and normal chest radiographic findings on admission