Abstract
Background
Methods
Results
ACKNOWLEDGEMENTS
Notes
AUTHOR CONTRIBUTIONS
Kim HK collected and analyzed the data and drafted the manuscript. Lee SG, Chae H, Song SH, Ahn S, Kim S, and Lee SM analyzed the data and interpreted the results. Park HD, Lee YH, Yun YM, and Lee SY contributed to the conception. SC designed and supervised the study. All authors acquired data from each institution, reviewed the manuscript, and provided critical feedback.
REFERENCES


































FIGURE AND TABLES
Fig. 1
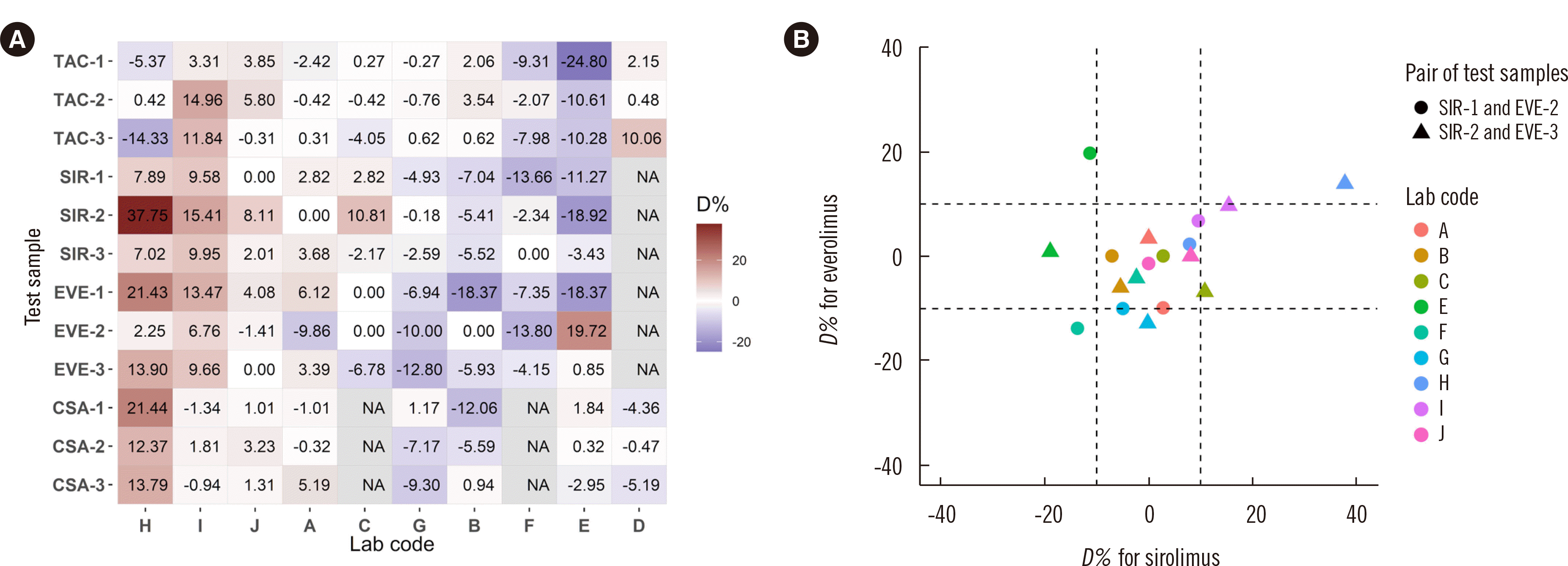
Table 1
Laboratory code | Test drugs | Internal standard | LC manufacturer* | MS instrument manufacturer* | MS instrument model |
---|---|---|---|---|---|
A | TAC+SIR+EVE | Ascomycin+SIR-d3+EVE-d4 | Shimadzu | SCIEX | Triple Quad 4500 |
CSA | CSD | Agilent | Agilent | 6460 Triple Quadrupole | |
MPA | Indomethacin | Waters | Waters | Quattro Micro | |
B | TAC+SIR+EVE+CSA | Ascomycin | Waters | Waters | Quattro Premier |
C | TAC+SIR+EVE | Ascomycin+SIR-d3+EVE-d4 | Waters | Waters | Xevo TQ-S |
D | TAC+CSA | Ascomycin+CSD | Waters | Waters | Xevo TQD |
MPA | Indomethacin | Waters | Waters | Xevo TQD | |
E | TAC+SIR+EVE | Ascomycin | Waters | Waters | Xevo TQD |
CSA | CSD | Waters | Waters | Xevo TQD | |
F | TAC+SIR+EVE+CSA | Ascomycin+EVE-d4+CSD | Agilent | Agilent | 6490 Triple Quadrupole |
G | TAC+SIR+EVE+CSA | Ascomycin+SIR-d3+EVE-d4+CSD | Agilent | SCIEX | API 4000 |
H | TAC+SIR+EVE+CSA | TAC-13C,d2+SIR-d3+EVE-d4+CSA-d4 | Agilent | SCIEX | API 3200 |
I | TAC+SIR+EVE+CSA | Ascomycin | Agilent | SCIEX | QTRAP 5500 |
MPA | MPA-d3 | Agilent | SCIEX | QTRAP 5500 | |
J | TAC+SIR+EVE+CSA | Ascomycin+CSD | Agilent | SCIEX | Triple Quad 3500 |
Most laboratories used precipitation with organic solvent mixture followed by centrifugation for extraction, except Lab I, which used liquid/liquid extraction. All the participating laboratories used the commercial calibrator from Chromsystems Instruments & Chemicals GmbH (Gräfelfing, Germany).
Table 2
Table 3
Table 4
Experiment | Drug | Condition | Coefficient β2 (95% CI) | P* | Expected concentration at 6 ng/mL (%diff) | |
---|---|---|---|---|---|---|
Reconstitution | TAC | A0 | Baseline | 6.000 | (Baseline) | |
B0 | 0.00012 (-0.00034–0.00058) | 0.612 | 5.997 | (-0.049) | ||
SIR | A0 | Baseline | 6.000 | (Baseline) | ||
B0 | -0.00005 (-0.00043–0.00032) | 0.772 | 6.002 | (0.029) | ||
EVE | A0 | Baseline | 6.000 | (Baseline) | ||
B0 | -0.00015 (-0.00036–0.00005) | 0.141 | 6.007 | (0.112) | ||
Storage | TAC | A1 | Baseline | 6.000 | (Baseline) | |
A2 | -0.00037 (-0.00102–0.00028) | 0.257 | 6.009 | (0.143) | ||
A3 | -0.00028 (-0.00093–0.00037) | 0.386 | 6.007 | (0.109) | ||
A4 | -0.00022 (-0.00087–0.00043) | 0.496 | 6.005 | (0.086) | ||
SIR | A1 | Baseline | 6.000 | (Baseline) | ||
A2 | -0.00157 (-0.00239–-0.00076) | < 0.001 | 6.033 | (0.555) | ||
A3 | -0.00091 (-0.00173–-0.00009) | 0.030 | 6.019 | (0.321) | ||
A4 | -0.00061 (-0.00143–0.00020) | 0.138 | 6.013 | (0.217) | ||
EVE | A1 | Baseline | 6.000 | (Baseline) | ||
A2 | -0.00043 (-0.00098–0.00012) | 0.121 | 6.013 | (0.222) | ||
A3 | -0.00117 (-0.00172–-0.00062) | < 0.001 | 6.036 | (0.598) | ||
A4 | -0.00075 (-0.00130–-0.00020) | 0.009 | 6.023 | (0.384) |