Abstract
Background
Patients with coronavirus disease 2019 (COVID-19) can unknowingly spread the virus to several people during the early subclinical period.
Methods
We evaluated the viral dynamics in various body fluid specimens, such as nasopharyngeal swab, oropharyngeal swab, saliva, sputum, and urine specimens, of two patients with COVID-19 from hospital day 1 to 9. Additional samples of the saliva were taken at 1 hour, 2 hours, and 4 hours after using a chlorhexidine mouthwash. The severe acute respiratory syndrome coronavirus 2 (SARS-CoV-2) viral load was determined by real-time reverse transcriptase polymerase chain reaction (rRT-PCR).
Results
SARS-CoV-2 was detected from all the five specimens of both patients by rRT-PCR. The viral load was the highest in the nasopharynx (patient 1 = 8.41 log10 copies/mL; patient 2 = 7.49 log10 copies/mL), but it was also remarkably high in the saliva (patient 1 = 6.63 log10 copies/mL; patient 2 = 7.10 log10 copies/mL). SARS-CoV-2 was detected up to hospital day 6 (illness day 9 for patient 2) from the saliva of both patients. The viral load in the saliva decreased transiently for 2 hours after using the chlorhexidine mouthwash.
Graphical Abstract
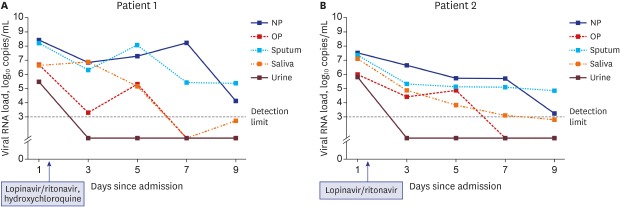
In Korea, since the first report of coronavirus disease 2019 (COVID-19) in January 20, 2020, 11,065 cases of COVID-19 have been confirmed as of May 18, 2020.1 The government of Korea declared the highest alert level (Level IV) in February 23, 2020 because the confirmed number of COVID-19 cases were increasing. Both subclinical manifestations and early high viral loads have been suggested as important factors contributing to the sudden outbreak in Korea.23
Patients with COVID-19 can spread the virus to several people during the early subclinical period before being diagnosed.2 Usually, the symptoms are mild in the earlier stages of the disease, but the condition of the patient rapidly deteriorate 7–10 days after the infection, leading to death in 1.4%–2.3% of patients.456 To understand the transmission dynamics and reinforce infection control practices, assessment of viral shedding is required in a diverse range of body fluids.
From hospital days 1–9, serial clinical specimens were collected every 2 days from two patients diagnosed with COVID-19. Nasopharyngeal and oropharyngeal samples were collected using polyester flocked swabs. Saliva and sputum specimens were sampled by the patients themselves after teaching them the collection method. Each collected sample was inserted into separated tubes containing 2 mL of the virus transport medium. The urine was sampled in a covered collection cup and sealed for safe transportation to the laboratory.
On hospital days 3 and 6, the patients used the chlorhexidine gluconate solution mouthwash (0.12%, 15 mL) for 30 seconds after baseline specimen sampling. Patients were educated on the entire process of gargling and saliva sampling and were directly supervised by the investigator. Additional samples of the saliva were taken at 0 hour (before gargle), 1 hour, 2 hours, and 4 hours after using the chlorhexidine mouthwash.
The presence of SARS-CoV-2 was confirmed by real-time reverse transcriptase polymerase chain reaction (rRT-PCR). RNA was extracted from the clinical samples using NucliSENS easyMAG platform (bioMérieux, Marcy-l'Étoile, France). rRT-PCR was performed using CFX96 real-time PCR detection system (Bio-rad, Hercules, CA, USA) and PowerChek 2019-nCoV real-time PCR kit (Kogenebiotech, Seoul, Korea), which targeted the E and RdRP genes of SARS-CoV-2. The primer and probe sequences were designed according to the previous study.7 The sample was defined as negative if the cycle threshold (Ct) value exceeded 35 cycles. Details regarding the rRT-PCR protocol are provided in the Supplementary Appendix. The RNA copy number was calculated from the Ct values using the standard curve generated by dilution of the plasmid DNA.
Two patients with COVID-19 were admitted to our hospital. Patient 1, a 46-year-old female, presented with mild dizziness and fatigue, while patient 2, a 65-year-old female, complained of mild sore throat followed by general weakness before COVID-19 confirmation. Both patients did not have fever, and the laboratory test results were unremarkable (Supplementary Table 1). On hospital day 1 (illness day 4), the chest radiograph of patient 2 showed pneumonia in the left lower lung field (Supplementary Fig. 1). During the study period from hospital days 1–9, patients were administered an antiviral agent for 10 days after the first sampling of the diverse body fluids; patient 1 received lopinavir/ritonavir 400 mg/100 mg twice a day and hydroxychloroquine 400 mg once daily, while patient 2 received only lopinavir/ritonavir 400 mg/100 mg twice a day.
On hospital day 1, SARS-CoV-2 was detected from all five specimens (nasopharynx, oropharynx, saliva, sputum, and urine) of both patients using rRT-PCR (Fig. 1 and Supplementary Table 2). The viral load was the highest in the nasopharynx (patient 1 = 8.41 log10 copies/mL; patient 2 = 7.49 log10 copies/mL), but it was also remarkably high in the oropharynx (patient 1 = 6.65 log10 copies/mL; patient 2 = 5.99 log10 copies/mL), saliva (patient 1 = 6.63 log10 copies/mL; patient 2 = 7.10 log10 copies/mL), and sputum (patient 1 = 8.22 log10 copies/mL; patient 2 = 7.33 log10 copies/mL). SARS-CoV-2 was detected initially in the urine samples (patient 1 = 5.48 log10 copies/mL; patient 2 = 5.79 log10 copies/mL), but it was not detected from hospital day 3. The viral load in each specimen decreased gradually in the serial samples. SARS-CoV-2 was detected up to hospital day 6 (illness day 9 for patient 2) from the saliva in both patients (Figs. 1, 2 and Supplementary Table 2).
The viral load in the saliva decreased transiently for 2 hours after using the chlorhexidine mouthwash, but it increased again at 2–4 hours post-mouthwash (Fig. 2 and Supplementary Table 3).
In this study, we have shown that the SARS-CoV-2 viral load is consistently high in the saliva; it was relatively higher than that in the oropharynx during the early stage of COVID-19. This finding suggests that SARS-CoV-2 might be secreted from the salivary gland rather than the nasopharynx. Chlorhexidine mouthwash was effective in reducing the SARS-CoV-2 viral load in the saliva for a short-term period.
Transmission of coronaviruses occurs primarily by close contact via respiratory droplets, which are generated by sneezing, coughing, breathing, and talking. According to a study measuring the respiratory droplets, the size was approximately the same among sneezing, coughing, and talking, but the amount was the largest when sneezing.8 Contrary to seasonal coronavirus infections, nasal symptoms are infrequent and less prominent in the cases of novel coronavirus infections, including Middle Eastern respiratory syndrome and COVID-19.910 The Middle Eastern respiratory syndrome coronavirus (MERS-CoV) transmission was relatively limited in the community, but a large number of community-acquired SARS-CoV-2 infections have been reported in many countries worldwide, such as China, Korea, Italy and Spain. Similar to the two cases in this study, several cases of SARS-CoV-2 infection have occurred in Korea even after a short conversation with patients with subclinical respiratory symptoms.1 Owing to its high viral load in the saliva, SARS-CoV-2 might be transmitted to other people during a conversation. This difference in transmission might be related to the receptor binding tropism; MERS-CoV binds to the dipeptidyl peptidase 4 (DPP4) receptor, while SARS-CoV-2 binds to the angiotensin converting enzyme 2 (ACE2) receptor, similar to seasonal coronaviruses.11 In humans, ACE2 is expressed both in the respiratory tract and the salivary gland duct epithelium, whereas DPP4 is exclusively expressed in the lower respiratory tract epithelium.1213
Coronaviruses are a group of enveloped positive-sense single-stranded RNA viruses. Considering that chlorhexidine compounds are effective against lipid-enveloped viruses, we prescribed a chlorhexidine (0.12%, 15 mL) mouthwash and found viral suppression for 2 hours after using the mouthwash once. However, in patient 1, there was no decrease in viral load in the saliva 1 hour after using the mouthwash (hospital day 6), which might be due to mixing of the saliva specimen with the postnasal drip or incomplete gargling. Therefore, chlorhexidine mouthwash might be beneficial for the control of SARS-CoV-2 transmission in both the community and hospital setting.
Saliva can be easily collected from patients without performing any invasive procedures. The time and cost associated with the collection of saliva were reported to be 2.26-fold and 2.59-fold lower than that of nasopharyngeal sampling.14 In addition, collecting the saliva specimen is safe and efficient during a novel infectious disease epidemic because patients are able to provide samples of their saliva themselves. Recent studies have shown that the saliva might be a valuable specimen for the diagnosis of respiratory virus infections compared to nasopharyngeal swabs.1415 Similarly, the results in this study also suggest that saliva specimens can be used for the diagnosis of SARS-CoV-2 infection. However, the viral load in the saliva might decrease during the late stage of the infection. Further research is needed to determine whether saliva specimens can help diagnose SARS-CoV-2 infection in patients with advanced pneumonia.
Lopinavir/ritonavir appears to suppress viremia, but it might be ineffective in controlling respiratory tract infections. In a mouse study for MERS-CoV, lopinavir/ritonavir did not reduce virus replication or severe pathology in the lung tissue, contrary to remdesivir.16 Lopinavir/ritonavir is an anti-HIV drug, developed to achieve a high concentration in the plasma and lymphatic tissue, but not in the lung tissue. Pharmacokinetic data on the respiratory tract concentration of lopinavir/ritonavir are lacking, and further research is, therefore, warranted.
There are several limitations to this study. The study was only conducted in a small number of patients and did not include controls (gargling with saline) for comparison. Large-scale studies, including control subjects, are required to minimize the confounding effects of disease severity, sampling errors, and other factors. In addition, environmental surveillance should be included in the study design of future studies. Although rRT-PCR results were not positive for the 15 environmental specimens at day 4 and day 14 (data not shown), the possibility of environmental contamination should be considered. Second, a viral culture test was not conducted in this study. Therefore, it was not possible to determine the survival of the virus over time. Finally, the standardized plasmid DNA was not use. Ct values were converted to RNA copy number using plasmid DNA for an intuitive comparison of changes in the viral concentration. Since standardized plasmid DNA was not available, a self-constructed plasmid DNA was used.
In summary, we analyzed the serial viral load in the nasopharynx, oropharynx, saliva, sputum, and urine samples of two patients with COVID-19. During the early stage of infection, the viral load of SARS-CoV-2 was remarkably high in the nasopharynx and the saliva. Chlorhexidine mouthwash could be beneficial in controlling droplet transmission in addition to mask wearing and hand washing.
Notes
Author Contributions:
Conceptualization: Yoon JG, Song JY.
Data curation: Yoon JG, Yoon J, Yoon SY, Seong H, Noh JY.
Formal analysis: Yoon JG, Yoon J, Song JY.
Investigation: Yoon JG, Seong H, Noh JY, Song JY, Cheong HJ, Kim WJ
Methodology: Yoon JG, Yoon J, Yoon SY, Lim CS.
Validation: Yoon JG, Yoon J, Yoon SY, Lim CS.
Writing - original draft: Yoon JG, Yoon J, Song JY.
Writing - review & editing: Yoon JG, Yoon J, Song JY, Yoon SY, Lim CS, Seong H, Noh JY, Cheong HJ, Kim WJ.
References
1. Ministry of Health and Welfare, Korea Centers for Disease Control and Prevention. Updates on 2019 Novel Coronavirus: for Press Release. Cheongju: Korea Centers for Disease Control and Prevention;2020.
2. Song JY, Yun JG, Noh JY, Cheong HJ, Kim WJ. Covid-19 in South Korea - Challenges of subclinical manifestations. N Engl J Med. 2020; 382(19):1858–1859. PMID: 32251568.


3. Pan Y, Zhang D, Yang P, Poon LL, Wang Q. Viral load of SARS-CoV-2 in clinical samples. Lancet Infect Dis. 2020; 20(4):411–412. PMID: 32105638.


4. Huang C, Wang Y, Li X, Ren L, Zhao J, Hu Y, et al. Clinical features of patients infected with 2019 novel coronavirus in Wuhan, China. Lancet. 2020; 395(10223):497–506. PMID: 31986264.


5. To KK, Tsang OT, Chik-Yan Yip C, Chan KH, Wu TC, Chan JM, et al. Consistent detection of 2019 novel coronavirus in saliva. Clin Infect Dis. 2020; ciaa149. PMID: 32047895.


6. Guan WJ, Ni ZY, Hu Y, Liang WH, Ou CQ, He JX, et al. Clinical characteristics of coronavirus disease 2019 in China. N Engl J Med. 2020; 382(18):1708–1720. PMID: 32109013.
7. Corman VM, Landt O, Kaiser M, Molenkamp R, Meijer A, Chu DK, et al. Detection of 2019 novel coronavirus (2019-nCoV) by real-time RT-PCR. Euro Surveill. 2020; 25(3):2000045.


8. Duguid JP. The size and the duration of air-carriage of respiratory droplets and droplet-nuclei. J Hyg (Lond). 1946; 44(6):471–479. PMID: 20475760.


9. Choi WS, Kang CI, Kim Y, Choi JP, Joh JS, Shin HS, et al. Clinical presentation and outcomes of Middle East respiratory syndrome in the Republic of Korea. Infect Chemother. 2016; 48(2):118–126. PMID: 27433382.
10. Lee N, Hui D, Wu A, Chan P, Cameron P, Joynt GM, et al. A major outbreak of severe acute respiratory syndrome in Hong Kong. N Engl J Med. 2003; 348(20):1986–1994. PMID: 12682352.


11. Letko M, Marzi A, Munster V. Functional assessment of cell entry and receptor usage for SARS-CoV-2 and other lineage B betacoronaviruses. Nat Microbiol. 2020; 5(4):562–569. PMID: 32094589.


12. Widagdo W, Begeman L, Schipper D, Run PR, Cunningham AA, Kley N, et al. Tissue distribution of the MERS-Coronavirus receptor in bats. Sci Rep. 2017; 7(1):1193. PMID: 28446791.


13. Liu L, Wei Q, Alvarez X, Wang H, Du Y, Zhu H, et al. Epithelial cells lining salivary gland ducts are early target cells of severe acute respiratory syndrome coronavirus infection in the upper respiratory tracts of rhesus macaques. J Virol. 2011; 85(8):4025–4030. PMID: 21289121.


14. To KK, Yip CC, Lai CY, Wong CK, Ho DT, Pang PK, et al. Saliva as a diagnostic specimen for testing respiratory virus by a point-of-care molecular assay: a diagnostic validity study. Clin Microbiol Infect. 2019; 25(3):372–378. PMID: 29906597.


15. Kim YG, Yun SG, Kim MY, Park K, Cho CH, Yoon SY, et al. Comparison between saliva and nasopharyngeal swab specimens for detection of respiratory viruses by multiplex reverse transcription-PCR. J Clin Microbiol. 2016; 55(1):226–233. PMID: 27807150.


16. Sheahan TP, Sims AC, Leist SR, Schäfer A, Won J, Brown AJ, et al. Comparative therapeutic efficacy of remdesivir and combination lopinavir, ritonavir, and interferon beta against MERS-CoV. Nat Commun. 2020; 11(1):222. PMID: 31924756.


SUPPLEMENTARY MATERIALS
Supplementary Data 1
Real-time reverse transcriptase polymerase chain reaction (rRT-PCR) laboratory procedure
Supplementary Table 3
Serial viral loads from saliva after chlorhexidine mouthwash