Abstract
Objective
Increased gingival elasticity has been implicated as the cause of relapse following orthodontic rotational tooth movement and approaches to reduce relapse are limited. This study aimed to investigate the effects of sulforaphane (SFN), an inhibitor of osteoclastogenesis, on gene expression in gingival fibroblasts and relapse after rotational tooth movement in beagle dogs.
Methods
The lower lateral incisors of five beagle dogs were rotated. SFN or dimethylsulfoxide (DMSO) were injected into the supra-alveolar gingiva of the experimental and control group, respectively, and the effect of SFN on relapse tendency was evaluated. Changes in mRNA expression of extracellular matrix components associated with gingival elasticity in beagles were investigated by real-time polymerase chain reaction. Morphology and arrangement of collagen fibers were observed on Masson’s trichrome staining of buccal gingival tissues of experimental and control teeth.
Results
SFN reduced the amount and percentage of relapse of orthodontic rotation. It also decreased the gene expression of lysyl oxidase and increased the gene expression of matrix metalloproteinase (MMP) 1 and MMP 12, compared with DMSO control subjects. Histologically, collagen fiber bundles were arranged irregularly and were not well connected in the SFN-treated group, whereas the fibers extended in parallel and perpendicular directions toward the gingiva and alveolar bone in a more regular and well-ordered arrangement in the DMSO-treated group.
The relapse rate of rotated teeth after orthodontic treatment is higher than in other forms of tooth movement. Studies on the biological mechanisms of orthodontic relapse and on various methods to reduce relapse rates are ongoing. Based on early studies, during the course of orthodontic rotation, the supra-alveolar gingival fibers retain their attachment to the tooth, displacing the gingiva toward the tooth, and the resulting stretching force of the gingival fibers pulls the rotated tooth toward its pretreatment position, causing relapse.1,2 Thus, gingival fiberotomy, in which the supracrestal fibers and transseptal fibers are severed from the tooth, are performed to remove the tension placed on the tooth, and this procedure reportedly improves orthodontic rotational relapse.3,4 However, following a circumferential supracrestal fiberotomy (CSF), Edwards5 observed a significant and unpredictable variation in individual teeth long-term (15 years), even if CSF was effective in reducing relapse in the early retention period.
In a study by Redlich et al.,6 after orthodontic rotation movement in beagle dogs, scanning and transmission electron microscopy of the gingival fibers around the rotated tooth showed torn, ripped, disorganized, and laterally spaced collagen fibers, with larger collagen fibrils and an increased number of elastic fibers in other regions of the gingiva. As these patterns were inconsistent with the patterns of stretching fibers reported in previous studies, the authors argued that the increased elastic property of the compressed gingiva, rather than stretched collagen fiber, was the cause of rotational relapse. This was similar to the results of previous studies in which orthodontic force placed on a tooth caused a marked increase in glycosaminoglycan, elastic fibers, and collagen fibers in the gingiva and periodontal ligament (PDL) and increased gingival elasticity.7-9 Supporting this argument, additional cytomolecular studies conducted by Redlich et al.10,11 demonstrated that the application of centrifugal force, similar to orthodontic force on gingival and PDL fibroblasts in dogs, increased the gene expression of collagen type I, reduced the gene expression of tissue collagenase, and increased tropoelastin, a precursor of elastic protein.
Pharmacological methods to inhibit relapse and promote gingival tissue remodeling in soft tissues such as the PDL and gingiva, rather than suppressing osteoclastic bone resorption or stimulating osteoblastic bone formation activity in the alveolar bone, have been reported. Relaxin decreased collagen synthesis and increases matrix metalloproteinase (MMP) 1 synthesis in the PDL, which stimulated connective tissue remodeling in this tissue and reduced relapse.12,13 Moreover, Kanzaki et al.14 reported that when sulforaphane (SFN), a compound extracted from broccoli that activates nuclear factor E2-related factor (Nrf2), was injected into the gingiva of mice with observed tooth movement and relapse patterns, the rate of tooth movement decreased and relapse was reduced. Interestingly, this finding revealed that the effect of SFN on inhibiting relapse was larger than its inhibitory effect on tooth movement, which was presumed to be attributed to its ability to promote gingival tissue remodeling.
SFN is known to be particularly effective in activating Nrf2 and inhibiting osteoclast activity, among other activators of Nrf2. Nrf2 is a transcription factor that regulates the induction of cytoprotective enzymes, and is secreted by the body as a defense against reactive oxygen species (ROS) that damage cells.15 ROS act as signaling molecules of receptor activator of nuclear factor kappa-B ligand (RANKL), a key cytokine that mediates the dissolution and absorption of bone components during osteoclastogenesis and activates osteoclasts; whereas, Nrf2 inhibits RANKL-mediated osteoclastogenesis induced by ROS and has been shown to reduce the rate of tooth movement.16 In addition, Nrf2 effectively stimulated epithelial wound healing by inducing cell migration17,18 and inhibited scar formation through its anti-fibrotic effect19,20 in the skin and soft tissues of other organs.
Given these previous results, we hypothesized that SFN affects soft tissues by providing support for tissue remodeling and alleviating fibrous tissue distortion, which might inhibit orthodontic relapse of rotated teeth. Thus, this study aimed to investigate the effects of SFN treatment on changes in the extracellular matrix (ECM) components of fibroblasts associated with gingival elasticity, which are thought to be the cause of relapse, as well as its effect on orthodontic relapse after actual rotational tooth movement in beagle dogs.
The animal experimental protocol used in this study was approved by the Institutional Animal Care and Use Committee, Yonsei University Health System (IACUC approval No. 2018-0226). Five male beagle dogs (age, 12–15 months; weight, 10–13 kg; OrientBio, Seongnam, Korea) were used in this study. Only beagles with good tooth arrangements and a healthy periodontium were selected. All beagles were housed individually in cages and bred according to the guideline for the Care and Use of Laboratory Animals of medium and large size of the Department of Laboratory Animal Resources, Yonsei Biomedical Research Institute, Yonsei University College of Medicine.
For all experimental procedures, dogs were anesthetized intravenously with 1% alfaxan (5 mg/kg, Jurox, Rutherford NSW, Australia) or via the inhalation of 2% isoflurane (Sigma-Aldrich, St. Louis, MO, USA) depending on the time required for the experiment. The dogs were continuously monitored during and after the procedure to check for any side effects of anesthesia, and no notable adverse reactions were observed.
Lingual buttons (Ormco Co., Orange, CA, USA) were bonded to the labial and lingual surfaces of the mandibular lateral incisors (I2 teeth) and on the labial surface of the mandibular canine (C1 teeth) of five beagle dogs using Superbond C&B (Sun Medical Co., Shiga, Japan). The teeth were rotated by applying rotational couple force with a power chain (Clear Generation II; Ormco Co.) for 6 weeks (Figure 1A). Each of the elastic chains engaged between buttons exerted initial forces of around 50 g.21 The power chain was replaced every 2 weeks and dogs were inspected every day for any debonding of bonded appliances. After 6 weeks of rotational movement, we generated a steel connection between lingual buttons by passively ligating them with a 0.2-mm steel ligature to retain the teeth in their current form.
A total of 10 lateral incisors for five beagles was examined, of which the right and left incisors were assigned to the control and experimental groups, respectively. During a retention period of 4 weeks, SFN (Sigma-Aldrich, 10 mM, 0.06 mL, the concentration and dosage were referred to in Kanzaki’s study14) for the experimental group, and dimethylsulfoxide (DMSO, solvent of SFN, 0.06 mL) for control groups, were injected into the supra-alveolar gingiva of the buccal side of the rotated teeth once per week, four times total, using a 31 G-needle insulin syringe (Ultra-Fine II 6 mm, 0.3 mL; Becton Dickinson Co., Franklin Lakes, NJ, USA). The needle was inserted at right angles to the attached gingiva area located directly below the boundary between free gingiva and attached gingiva, vertically, and at both centers of half the buccal gingiva of the experimental teeth, horizontally. After this, SFN and DMSO were injected into each side of 3 units (Figure 1B and 1C). One week after the last injection, the appliances were removed to allow relapse of the rotated teeth. An intraoral impression was obtained immediately (T1) after the orthodontic appliances were removed and 2–4 weeks later (T2, T3) to create dental models, which were compared to the initial model (T0), before the application of the orthodontic force, to measure the extent of tooth rotation. The degree of relapse of the rotated teeth in the experimental and control groups was also compared and analyzed. The experimental schedule for the beagle dogs is briefly illustrated in Figure 2.
Dental models of the beagles (T0–T3) were scanned with a 3Shape Lab Scanner (Lab scanner E3; 3Shape Co., Copenhagen K, Denmark), and the amount of tooth rotation was measured using the Geomagic Control XTM (3D SYSTEMS, Seoul, Korea) software. The angle between the line connecting the cusp tip of the second premolars and the extension line through the incisal edges of the left and right experimental teeth (I2, n = 10) was measured to determine original, rotational, and relapse angles (Figure 3). With these measurements, the degree of rotation (T1–T0), amount of relapse after 2 and 4 weeks (T1–T2, T1–T3), and percentage of total relapse after 2 and 4 weeks (T1–T2 / T1–T0 × 100%, T1–T3 / T1–T0 × 100%) were calculated to determine whether SFN could reduce orthodontic relapse of the rotated teeth.
The relapse angle was measured after arranging the scanned images such that the occlusal plane of the mandibular molars was parallel to the ground. The angle was measured three times and the correlation coefficient of each measurement was obtained. The three measurements were highly correlated, with a correlation coefficient ≥ 0.99, in both the control and experimental groups, and thus, the average of the three values, rounded to three decimal places, was used for analysis.
The mesio-buccal supra-alveolar gingiva of the experimental teeth (n = 10) was dissected for real-time quantitative polymerase chain reaction (PCR) before sacrificing the beagles. We chose collagen type I alpha 1 (COL1A1), lysyl oxidase (LOX), elastin (ELN), MMP 1, MMP 12, and tissue inhibitor of metalloproteinases-1 (TIMP 1) as ECM components associated with the elasticity of the gingiva. To identify changes in the expression level of genes after SFN injection, total RNA was isolated from each mesio-buccal supra-alveolar gingiva of the experimental teeth injected with either DMSO or SFN using TRIzol reagent (Gibco BRL, New York, NY, USA). Three biologic replicates were used. cDNA was synthesized from total RNA with Maxime RT PreMix (iNtRON, Seongnam, Korea). Quantitative PCR was performed using the StepOnePlus Real-Time PCR System (Applied Biosystems, Thermo Fisher Scientific, Beverly, MA, USA) and Power SYBR green master mix (Thermo Fisher Scientific), according to the manufacturer’s instructions. Quantitative PCR products were normalized using β-2-microglobulin (B2M) levels as internal controls. Primer sequences used for real-time quantitative PCR are shown in Table 1. Data were analyzed using the StepOne (Applied Biosystems, Thermo Fisher Scientific) analysis software and the 2−∆∆Ct method. All PCRs were performed in triplicate.
After the beagles were sacrificed via an intravenous injection of KCl, distobuccal half segments of the experimental teeth were dissected (n = 10), fixed in 4% paraformaldehyde solution at 4°C for 2 days, and decalcified in ethylenediaminetetraacetic acid (EDTA) (10%, pH 7.4) solution for 4 months at 4°C. This EDTA solution was replaced twice per week. Fully decalcified samples were dehydrated in a graded ethanol series (70–100%), cleared in xylene, and then infiltrated and embedded in paraffin. Buccolingual serial sections, 3-µm thick, were cut parallel to the longitudinal axis of the experimental teeth. The qualified ones among the sections cut near the center of the tooth were selected and mounted.
Masson’s trichrome staining was performed to detect collagen fibers in gingival tissues. The distal images of Masson’s trichrome staining were observed using a Pannoramic 250 Flash III (3DHISTECH, Budapest, Hungary) and Caseviewer (ver. 2.0; 3DHISTECH).
All data are represented as means and standard deviations. Intergroup comparisons of mean values comprising the amount and percentage of relapse between the two groups followed a normal distribution and were analyzed with a paired t-test. Intergroup comparisons of the mean values of real-time quantitative PCR data were analyzed with a Wilcoxon signed rank test to compare DMSO and SFN groups. p-values less than 0.05 were considered statistically significant. IBM SPSS Statistics (ver. 23.0; IBM Corp., Armonk, NY, USA) was used for statistical analysis.
Table 2 shows intergroup and intragroup comparisons of the mean amount and percentage of rotation in the SFN and DMSO groups. There were no significant differences in the mean degree of rotation after 6 weeks between the groups, whereas the mean amount and percentage of relapse at 2 and 4 weeks after injection were significantly reduced in the experimental group compared to those in the control group (p < 0.05).
In the control group, no significant differences were found between the mean amount and percentage of rotational relapse at 2 and 4 weeks after the retention period, indicating that most relapse occurred within 2 weeks after completion of the retention period. In contrast, in the experimental group, significant differences were found between the mean amount and percentage of rotational relapse between 2 and 4 weeks after the retention period (p < 0.05), indicating that relapse occurred at a relatively slower rate.
In the mesio-buccal supra-gingival tissue of beagles, the expression of genes encoding COL1A1, LOX, ELN, MMP 1, MMP 12, and TIMP 1 were compared after SFN or DMSO treatment by real-time RT-PCR. The gene expression of COL1A1 (Figure 4A) was not significantly different between the two groups. However, the gene expression of LOX (Figure 4B) was decreased in the SFN-treated group compared to that in the DMSO group. The gene expression of ELN (Figure 4C) was not significantly different between the two groups. In addition, the gene expression of MMP 1 (Figure 4D) and MMP 12 (Figure 4E) were increased in the SFN-treated group compared to levels in the DMSO-treated group. On the other hand, the gene expression of TIMP1 (Figure 4F) was not significantly different between the two groups.
We next observed the histological appearance, morphology, and arrangement of collagen fibers in Masson’s trichrome-stained buccal gingival tissue of the experimental and control teeth (Figures 5 and 6). Collagen fibers bundles were longer and more connected in the DMSO-treated group than in the SFN-treated group (Figure 5C and 5D). The morphology and arrangement of the principal gingival fibers are shown in detail in larger images (Figure 6). The collagen fiber bundles that form dentogingival fibers in the SFN-treated group were short and not well connected to one another (Figure 6B). However, in the DMSO-treated group, we observed a more regular and well-ordered arrangement of the collagen fiber bundles connected to form the dentogingival fibers extending from the cervical cementum into the lamina propria of the free and attached gingiva (Figure 6A). Circular fibers are fiber bundles that run their course in the gingiva and encircle the tooth in a cuff- or ring-like fashion. In the SFN-treated group, circular fibers were arranged in a thinner and less compact manner compared to the arrangement in the DMSO-treated group (Figure 6C and 6D). The dentoperiosteal fibers extended from the cementum directly over the alveolar crest and then inclined apically to the periosteum of the alveolar bone. Similarly, collagen fiber bundles connected to form dentoperiosteal fibers were irregularly arranged and not well connected in the SFN-treated group (Figure 6F), whereas collagen fiber bundles were shown more regular and well-ordered arrangement in the DMSO-treated group (Figure 6E).
Our analysis of dental models from beagle dogs showed that the relapse tendency of rotational tooth movement was significantly reduced in the SFN experimental group compared to that in the control group. This result is similar to that of a study by Kanzaki et al.,14 which showed that 1 week after SFN injection, the relapse rate of tooth movement was 93% in the control group and 23% in the experimental group, and that SFN is effective in reducing the relapse of orthodontic tooth movement. However, the initial relapse rate in the present study was lower because the retention period after tooth movement was 4 weeks, which was longer than that in Kanzaki’s study,14 to allow SFN to sufficiently affect gingival elasticity. Moreover, the type of tooth movement in this study was rotational, unlike the tipping movement illustrated in Kanzaki’s study.14 In addition, in Kanzaki’s study,14 SFN was injected subperiosteally, making it difficult to differentiate whether the reduction in relapse rates was due to the inhibitory effect of Nrf2 on RANKL-mediated osteoclastogenesis or the induction of gingival tissue remodeling. In the present study, however, SFN was injected supraperiosteally in the supra-alveolar gingiva region, and its inhibitory effect on osteoclastogenesis could be discounted as much as possible, given our observation of its direct reducing effect on orthodontic relapse by inducing the remodeling of supporting tissues.
Gingival collagen has a relatively high turnover rate, although the turnover rate of PDL collagen is higher. Svoboda et al.22 reported that PDL contains five and 15 times as much phagocytosed collagen as attached gingiva and skin, respectively. The turnover of insoluble elastin under normal conditions is very slow due to extensive cross-linking.23,24 If increased collagen and elastin after orthodontic rotational movement remain in the gingival tissue for a prolonged time, orthodontic relapse of rotational movement increases. Meng et al.25 reported that after orthodontic rotational movement and an 8 week-retention period, the amount of collagen I normalized after 8 weeks of retention, but the increase in elastin and fibrillin 1 expression was maintained after the retention period and even after CSF; thus, collagen fibers are involved in short-term relapse, whereas elastic fibers affect long-term relapse.
Lox is known to be responsible for the enzymatic cross-linking of insoluble collagen and elastin,26 and plays an important role in the regulation of connective tissue accumulation.27,28 In the present study, SFN reduced the gene expression of LOX in the gingiva. We postulated that this inhibitory effect might facilitate collagen and elastin degradation by inhibiting the post-translational modification of collagen and elastin, reducing resistance to proteolysis by collagenases such as matrix metalloproteinases, MMP 1 and MMP 12.
The MMPs known to be elastolytic include MMP 2, MMP 7, MMP 9, MMP 12, and MT1-MMP, and of these, MMP 9 (gelatinase B) and MMP 12 (macrophage elastase) play a role as diagnostic markers of the progression of cardiovascular and respiratory diseases associated with elastin degradation.29,30 Here, we observed higher gene expression levels of MMP 1 and MMP 12 in the experimental group than in the control group. We believe that this increase in ECM degradation via proteolysis reduces the elasticity of the gingival tissue after orthodontic treatment and facilitates remodeling of this tissue, thereby helping to reduce relapse after orthodontic rotation. These degradations can also be seen from the results of Masson’s trichrome staining, with SFN causing a reduction in the assembly of collagen fiber bundles and the number of collagen fibers in the gingival tissue, by facilitating its degradation and reducing elasticity of the gingival tissue.
Several studies have reported the effect of Nrf2 on soft tissues based on Nrf2 knockdown with Nrf2 or Keap1 siRNA (specific small interfering RNA) transfection or Nrf2 activation using Nrf2 activators. Nrf2 is known to have antifibrotic effects, and Artaud-Macari et al.19 reported that Nrf2 activation reduced the expression of α-smooth muscle actin and transforming growth factor-β, which are characteristic factors of myofibroblastic differentiation during idiopathic pulmonary fibrosis. Kawarazaki et al.20 also reported that SFN inhibited the cell growth of keloid fibroblasts and reduced collagen deposition. In the present study, the Nrf2 activator SFN also exhibited anti-fibrotic effects by hindering the synthesis and facilitating the degradation of collagen and elastin.
To our best knowledge, this is the first study that investigated how the antifibrotic effects of SFN in gingival soft tissue influence orthodontic relapse of rotated teeth. As such, with only a few relevant studies available, there was no clear standard for the dose and duration of SFN administration. The dose of SFN used in this study was inferred from a study by Kanzaki et al.14 The beagle is approximately 30 times heavier than the mice. Thus, the dose of SFN administered to the beagles were 0.06 mL, which was 30 times the dose administered to the mice. However, additional cellular experiments would be required to determine if the dose of SFN used in this experiment was optimal.
In addition, the split-mouth design of local infiltration of SFN or DMSO without any carrier could have the possibility of interaction. Regardless, SFN is a water-insoluble liquid with a molecular weight of 177.29 (Sigma-Aldrich Chemical Co. #S4441) and the injection site was attached gingiva, which is a dense connective tissue; the injection depth was shallow and the distance between the left and right lateral incisors was more than 1.5 cm. Therefore, the injected SFN was not expected to spread beyond the dense connective tissue of the central incisor to the gingival tissue of the lateral incisor on the opposite side.31,32
Moreover, as this was an animal study with beagle dogs, the number of animals was not sufficient and the relapse pattern could not be observed over a longer retention period. Future investigations should consider the histological observation of elastin, which has been associated with a slower remodeling time.
Nevertheless, the results of the present study suggest that SFN treatment reduces orthodontic relapse of rotated teeth by suppressing gingival elasticity, attained by activating the metabolism of increased collagen and elastin after orthodontic rotational movement.
Increased elasticity of the gingiva after tooth movement has emerged as one of the causes of orthodontic relapse. SFN treatment changed the gene expression of LOX, MMP 1, and MMP 12, which was associated with increased gingival elasticity and reduction in the amount and percentage of orthodontic rotation relapse. SFN treatment based on the biological conversion of the data from beagle results and additional human cellular experiments may be considered as one of the pharmacologic approaches to prevent orthodontic rotational relapse.
REFERENCES
1. Reitan K. 1959; Tissue rearrangement during retention of orthodontically rotated teeth. Angle Orthod. 29:105–13.
2. Edwards JG. 1968; A study of the periodontium during orthodontic rotation of teeth. Am J Orthod. 54:441–61. DOI: 10.1016/0002-9416(68)90199-1. PMID: 5239302.


3. Edwards JG. 1970; A surgical procedure to eliminate rotational relapse. Am J Orthod. 57:35–46. DOI: 10.1016/0002-9416(70)90203-4. PMID: 5262003.


4. Kaplan RG. 1976; Clinical experiences with circumferential supracrestal fiberotomy. Am J Orthod. 70:147–53. DOI: 10.1016/S0002-9416(76)90315-8. PMID: 1066051.


5. Edwards JG. 1988; A long-term prospective evaluation of the circumferential supracrestal fiberotomy in alleviating orthodontic relapse. Am J Orthod Dentofacial Orthop. 93:380–7. DOI: 10.1016/0889-5406(88)90096-0. PMID: 3163217.


6. Redlich M, Rahamim E, Gaft A, Shoshan S. 1996; The response of supraalveolar gingival collagen to orthodontic rotation movement in dogs. Am J Orthod Dentofacial Orthop. 110:247–55. DOI: 10.1016/S0889-5406(96)80007-2. PMID: 8814024.


7. Ronnerman A, Thilander B, Heyden G. 1980; Gingival tissue reactions to orthodontic closure of extraction sites: histologic and histochemical studies. Am J Orthod. 77:620–5. DOI: 10.1016/0002-9416(80)90153-0. PMID: 6930162.
8. Franchi M, D'Aloya U, De Pasquale V, Caldini E, Graziani E, Borea G, et al. 1989; Ultrastructural changes of collagen and elastin in human gingiva during orthodontic tooth movement. Bull Group Int Rech Sci Stomatol Odontol. 32:139–43. PMID: 2620139.
9. Bumann A, Carvalho RS, Schwarzer CL, Yen EH. 1997; Collagen synthesis from human PDL cells following orthodontic tooth movement. Eur J Orthod. 19:29–37. DOI: 10.1093/ejo/19.1.29. PMID: 9071043.


10. Redlich M, Palmon A, Zaks B, Geremi E, Rayzman S, Shoshan S. 1998; The effect of centrifugal force on the transcription levels of collagen type I and collagenase in cultured canine gingival fibroblasts. Arch Oral Biol. 43:313–6. DOI: 10.1016/S0003-9969(97)00108-8. PMID: 9839707.


11. Redlich M, Asher Roos H, Reichenberg E, Zaks B, Mussig D, Baumert U, et al. 2004; Expression of tropoelastin in human periodontal ligament fibroblasts after simulation of orthodontic force. Arch Oral Biol. 49:119–24. DOI: 10.1016/j.archoralbio.2003.08.002. PMID: 14693205.


12. Takano M, Yamaguchi M, Nakajima R, Fujita S, Kojima T, Kasai K. 2009; Effects of relaxin on collagen type I released by stretched human periodontal ligament cells. Orthod Craniofac Res. 12:282–8. DOI: 10.1111/j.1601-6343.2009.01463.x. PMID: 19840280.


13. Hirate Y, Yamaguchi M, Kasai K. 2012; Effects of relaxin on relapse and periodontal tissue remodeling after experimental tooth movement in rats. Connect Tissue Res. 53:207–19. DOI: 10.3109/03008207.2011.628060. PMID: 22141456.


14. Kanzaki H, Shinohara F, Itohiya-Kasuya K, Ishikawa M, Nakamura Y. 2015; Nrf2 activation attenuates both orthodontic tooth movement and relapse. J Dent Res. 94:787–94. DOI: 10.1177/0022034515577814. PMID: 25795629.


15. Alam J, Stewart D, Touchard C, Boinapally S, Choi AM, Cook JL. 1999; Nrf2, a Cap'n'Collar transcription factor, regulates induction of the heme oxygenase-1 gene. J Biol Chem. 274:26071–8. DOI: 10.1074/jbc.274.37.26071. PMID: 10473555.


16. Kanzaki H, Shinohara F, Kajiya M, Kodama T. 2013; The Keap1/Nrf2 protein axis plays a role in osteoclast differentiation by regulating intracellular reactive oxygen species signaling. J Biol Chem. 288:23009–20. DOI: 10.1074/jbc.M113.478545. PMID: 23801334. PMCID: PMC3743476.


17. Demirovic D, Rattan SI. 2011; Curcumin induces stress response and hormetically modulates wound healing ability of human skin fibroblasts undergoing ageing in vitro. Biogerontology. 12:437–44. DOI: 10.1007/s10522-011-9326-7. PMID: 21380847.


18. Hayashi R, Himori N, Taguchi K, Ishikawa Y, Uesugi K, Ito M, et al. 2013; The role of the Nrf2-mediated defense system in corneal epithelial wound healing. Free Radic Biol Med. 61:333–42. DOI: 10.1016/j.freeradbiomed.2013.04.008. PMID: 23587556.


19. Artaud-Macari E, Goven D, Brayer S, Hamimi A, Besnard V, Marchal-Somme J, et al. 2013; Nuclear factor erythroid 2-related factor 2 nuclear translocation induces myofibroblastic dedifferentiation in idiopathic pulmonary fibrosis. Antioxid Redox Signal. 18:66–79. DOI: 10.1089/ars.2011.4240. PMID: 22703534.


20. Kawarazaki A, Horinaka M, Yasuda S, Numajiri T, Nishino K, Sakai T. 2017; Sulforaphane suppresses cell growth and collagen expression of keloid fibroblasts. Wound Repair Regen. 25:224–33. DOI: 10.1111/wrr.12512. PMID: 28120534.


21. Kim SJ, Kang YG, Park JH, Kim EC, Park YG. 2013; Effects of low-intensity laser therapy on periodontal tissue remodeling during relapse and retention of orthodontically moved teeth. Lasers Med Sci. 28:325–33. DOI: 10.1007/s10103-012-1146-8. PMID: 22814894.


22. Svoboda EL, Shiga A, Deporter DA. 1981; A stereologic analysis of collagen phagocytosis by fibroblasts in three soft connective tissues with differing rates of collagen turnover. Anat Rec. 199:473–80. DOI: 10.1002/ar.1091990404. PMID: 7270910.


23. Lefevre M, Rucker RB. 1980; Aorta elastin turnover in normal and hypercholesterolemic Japanese quail. Biochim Biophys Acta. 630:519–29. DOI: 10.1016/0304-4165(80)90006-9. PMID: 6772235.


24. Shapiro SD, Endicott SK, Province MA, Pierce JA, Campbell EJ. 1991; Marked longevity of human lung parenchymal elastic fibers deduced from prevalence of D-aspartate and nuclear weapons-related radiocarbon. J Clin Invest. 87:1828–34. DOI: 10.1172/JCI115204. PMID: 2022748. PMCID: PMC295305.


25. Meng M, Lv C, Yang Q, He S, Wu S, Liu Y, et al. 2018; Expression of proteins of elastic fibers and collagen type I in orthodontically rotated teeth in rats. Am J Orthod Dentofacial Orthop. 154:249–59. DOI: 10.1016/j.ajodo.2017.11.030. PMID: 30075927.


26. Reiser K, McCormick RJ, Rucker RB. 1992; Enzymatic and nonenzymatic cross-linking of collagen and elastin. FASEB J. 7:2439–49. DOI: 10.1096/fasebj.6.7.1348714. PMID: 1348714.


27. Niewoehner DE, Hoidal JR. 1982; Lung fibrosis and emphysema: divergent responses to a common injury? Science. 217:359–60. DOI: 10.1126/science.7089570. PMID: 7089570.


28. Ma RH, Tsai CC, Shieh TY. 1995; Increased lysyl oxidase activity in fibroblasts cultured from oral submucous fibrosis associated with betel nut chewing in Taiwan. J Oral Pathol Med. 24:407–12. DOI: 10.1111/j.1600-0714.1995.tb01210.x. PMID: 8537914.


29. Van Doren SR. 2015; Matrix metalloproteinase interactions with collagen and elastin. Matrix Biol. 44-46:224–31. DOI: 10.1016/j.matbio.2015.01.005. PMID: 25599938. PMCID: PMC4466143.


30. Skjøt-Arkil H, Clausen RE, Nguyen QH, Wang Y, Zheng Q, Martinez FJ, et al. 2012; Measurement of MMP-9 and -12 degraded elastin (ELM) provides unique information on lung tissue degradation. BMC Pulm Med. 12:34. DOI: 10.1186/1471-2466-12-34. PMID: 22818364. PMCID: PMC3515477.


31. Yamada H, Sato T, Fukayama H. 2011; Diffusion patterns of anesthetic solution in the infiltration anesthesia depending on injection sites in the mandible of rats. Oral Sci Int. 8:50–4. DOI: 10.1016/S1348-8643(11)00026-7.


32. Cantu R, Steffe JA. Maxey L, Magnusson J, editors. 2013. Soft tissue healing considerations after surgery. Rehabilitation for the postsurgical orthopedic patient. 3rd ed. Elsevier Mosby;Louis, Mo: p. 15–25. DOI: 10.1016/B978-0-323-07747-7.00002-2.


Figure 1
A, Occlusal view of a beagle’s lower teeth and orthodontic buttons with elastic chains. Mandibular lateral incisors (I2 teeth) of beagle dogs were rotated for 6 weeks by applying rotational couple force using a power chain. B, C, The needle injection points of gingiva of experimental teeth. After the needle was inserted at right angles to the attached gingiva area located directly below the boundary between free gingiva and attached gingiva, vertically, and at both centers of half of the buccal gingiva of the experimental teeth, horizontally, sulforaphane (SFN) and dimethylsulfoxide (DMSO) were injected into each side of 3 units (UI) (B, frontal view; C, sagittal view).
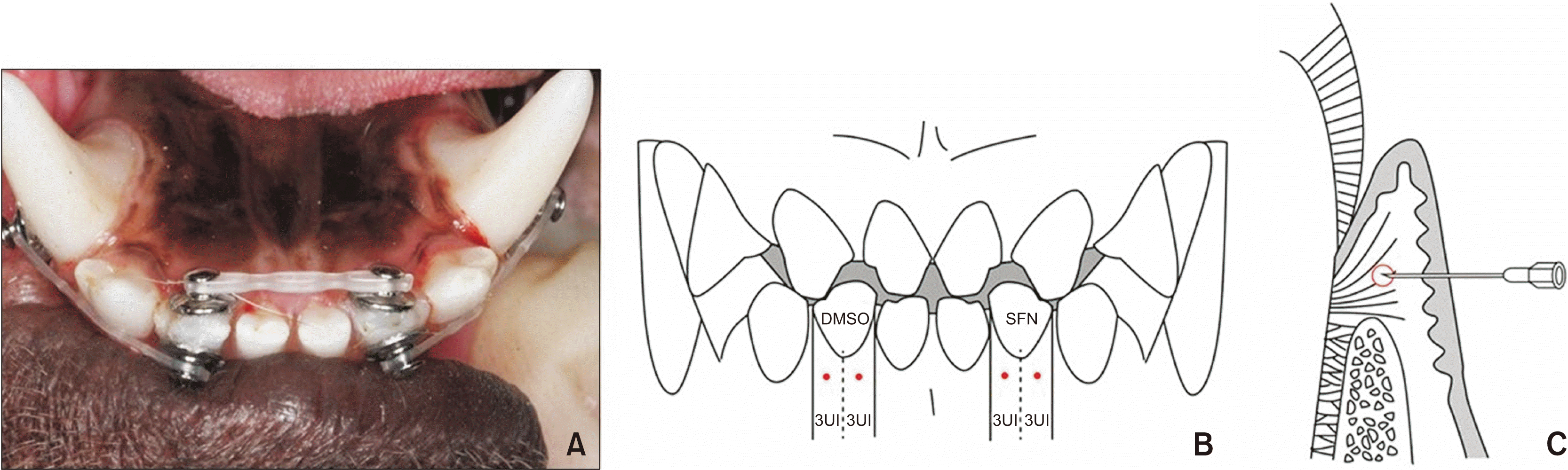
Figure 2
Schema. The experimental schedule for the beagle dogs. The experimental teeth were rotated by applying rotational couple force with a power chain for 6 weeks. During a retention period of 4 weeks, SFN (experimental group) and DMSO (control group) were injected into the supra-alveolar gingiva of the buccal side of the rotated teeth once per week, four times total. One week after the last injection, the appliances were removed to allow relapse of the rotated teeth. An intraoral impression was obtained to measure the extent of tooth rotation and relapse.
SFN, Sulforaphane; DMSO, dimethylsulfoxide; T0, initial; T1, immediately after retention and SFN treatment for 4 weeks; T2, after relapse for 2 weeks; T3, after relapse for 4 weeks.
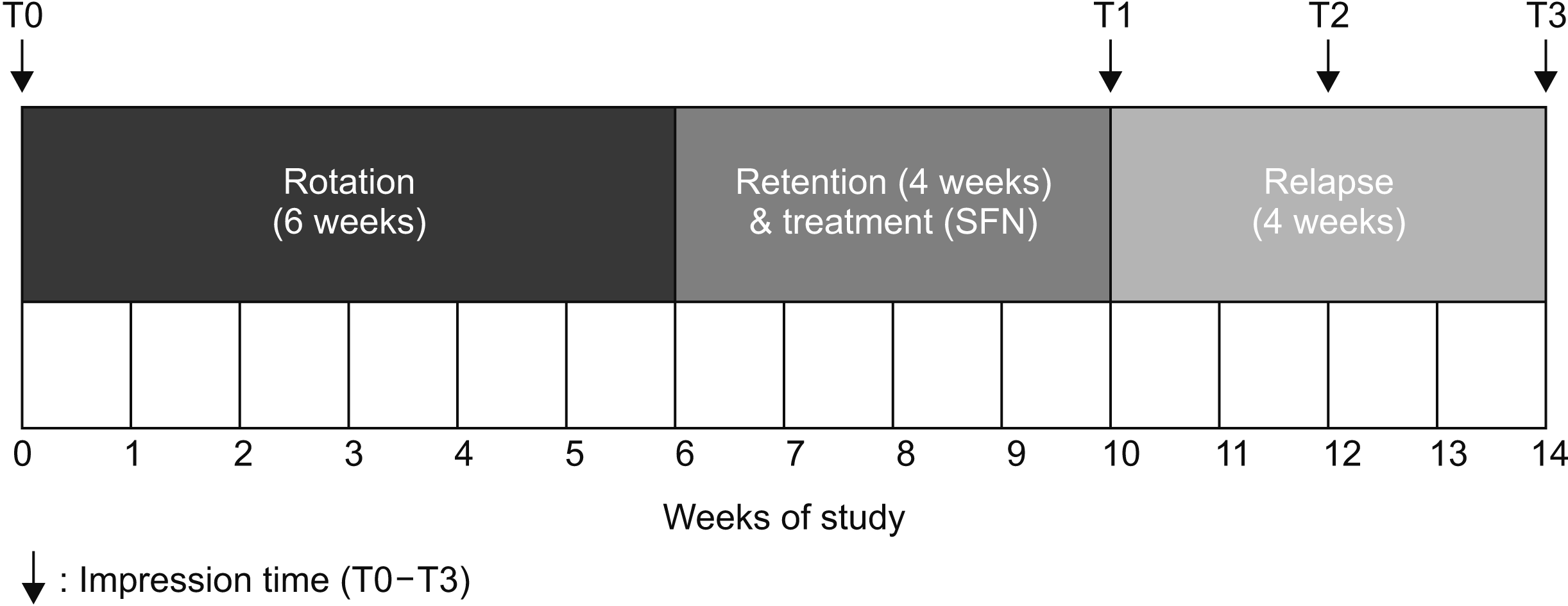
Figure 3
The extent of tooth rotation and relapse. Measurements were made using the Geomagic Control XTM (3D SYSTEMS, Seoul, Korea) software. The degree of rotation and amount of relapse after 2 and 4 weeks, and percentage of relapse after 2 and 4 weeks were calculated. A, Scanning image of T1 model from beagle no. 1. B, Scanning image of T2 model from beagle no. 1.
T1, Immediately after retention and SFN treatment for 4 weeks; T2, after relapse for 2 weeks.
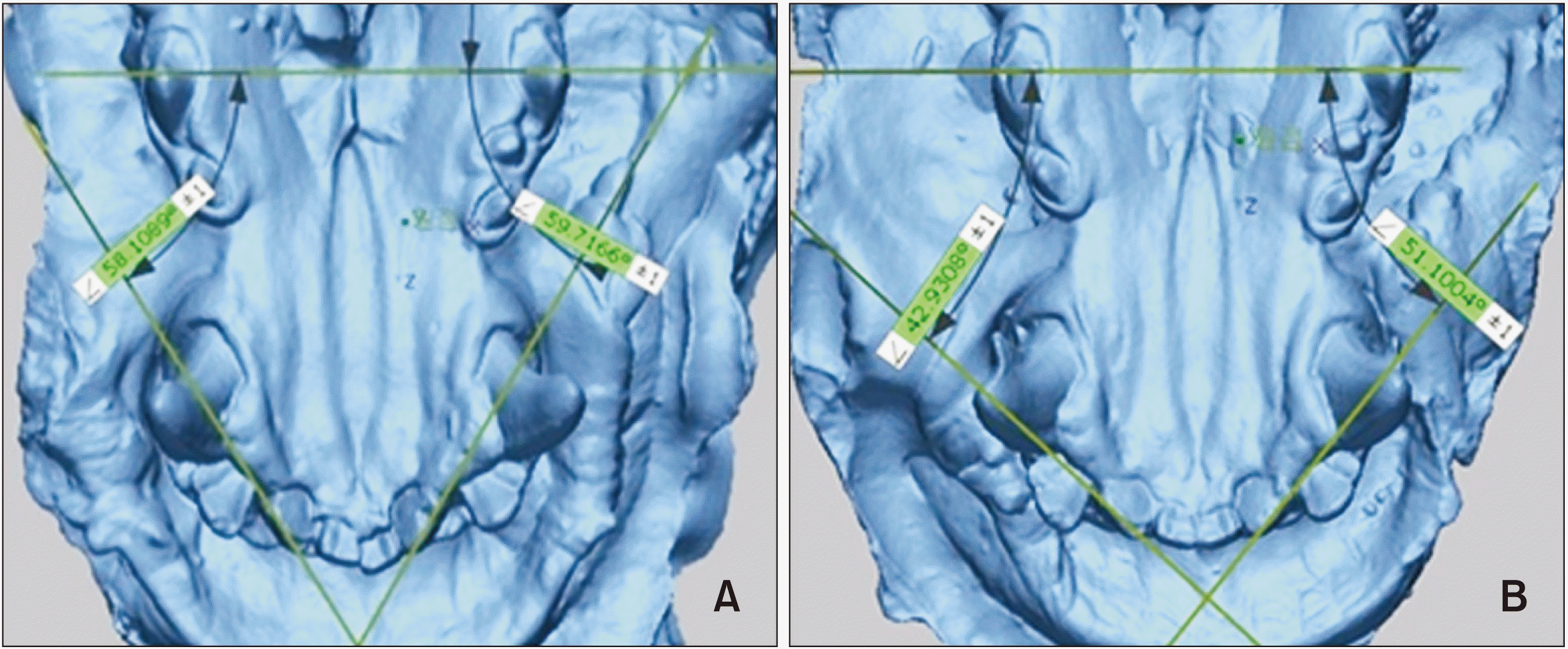
Figure 4
Relative mRNA expression of genes encoding COL1A1, LOX, ELN, MMP 1, MMP 12, and TIMP 1 in gingival tissue of beagle dogs after sulforaphane treatment. COL1A1 (A), LOX (B), ELN (C), MMP 1 (D), MMP 12 (E), and TIMP 1 (F) mRNA levels in gingival tissue were quantified by real-time polymerase chain reaction. The symbol (*) indicates statistical significance between the control group and experimental group (*p < 0.05, Wilcoxon signed rank test).
DMSO, Dimethylsulfoxide; COL1A1, collagen type I alpha 1; LOX, lysyl oxidase; ELN, elastin; MMP, matrix metalloproteinase; TIMP, tissue inhibitor of metalloproteinase.
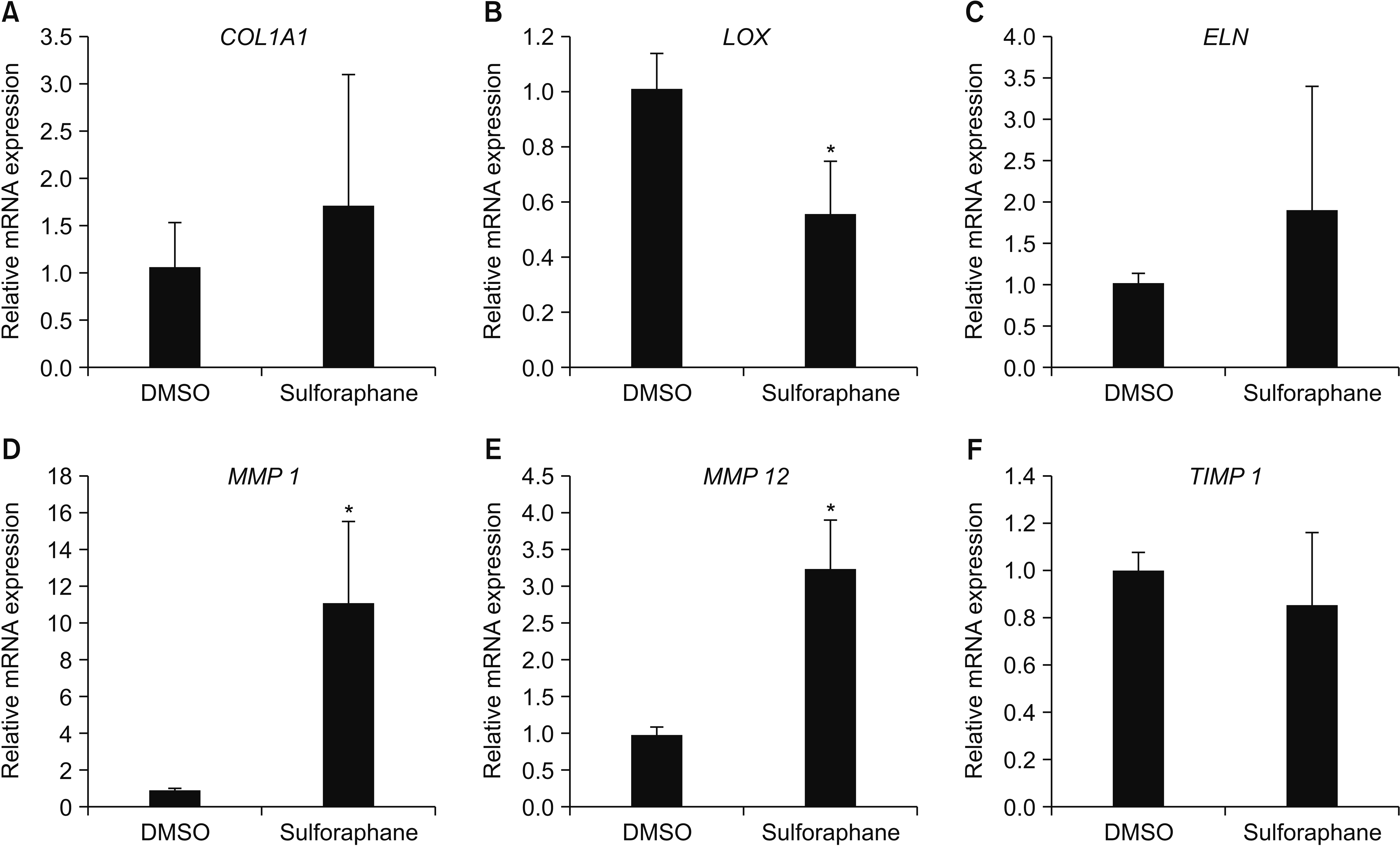
Figure 5
Histologic appearance of collagen fibers in buccal supra-alveolar gingiva. Massonʼs trichome staining of the buccal-lingual longitudinal sections of the lower lateral incisors of beagle dogs injected with either dimethylsulfoxide (DMSO) or sulforaphane (SFN) for 4 weeks. The control and experimental samples were from same beagle subject. Collagen fibers bundles were longer and more connected in the DMSO-treated group than in the SFN-treated group. A, C, DMSO group. B, D, SFN group. Scale bar = 1 mm (A, B), 400 μm (C, D). Higher magnification views of the box areas can be seen Figure 6.
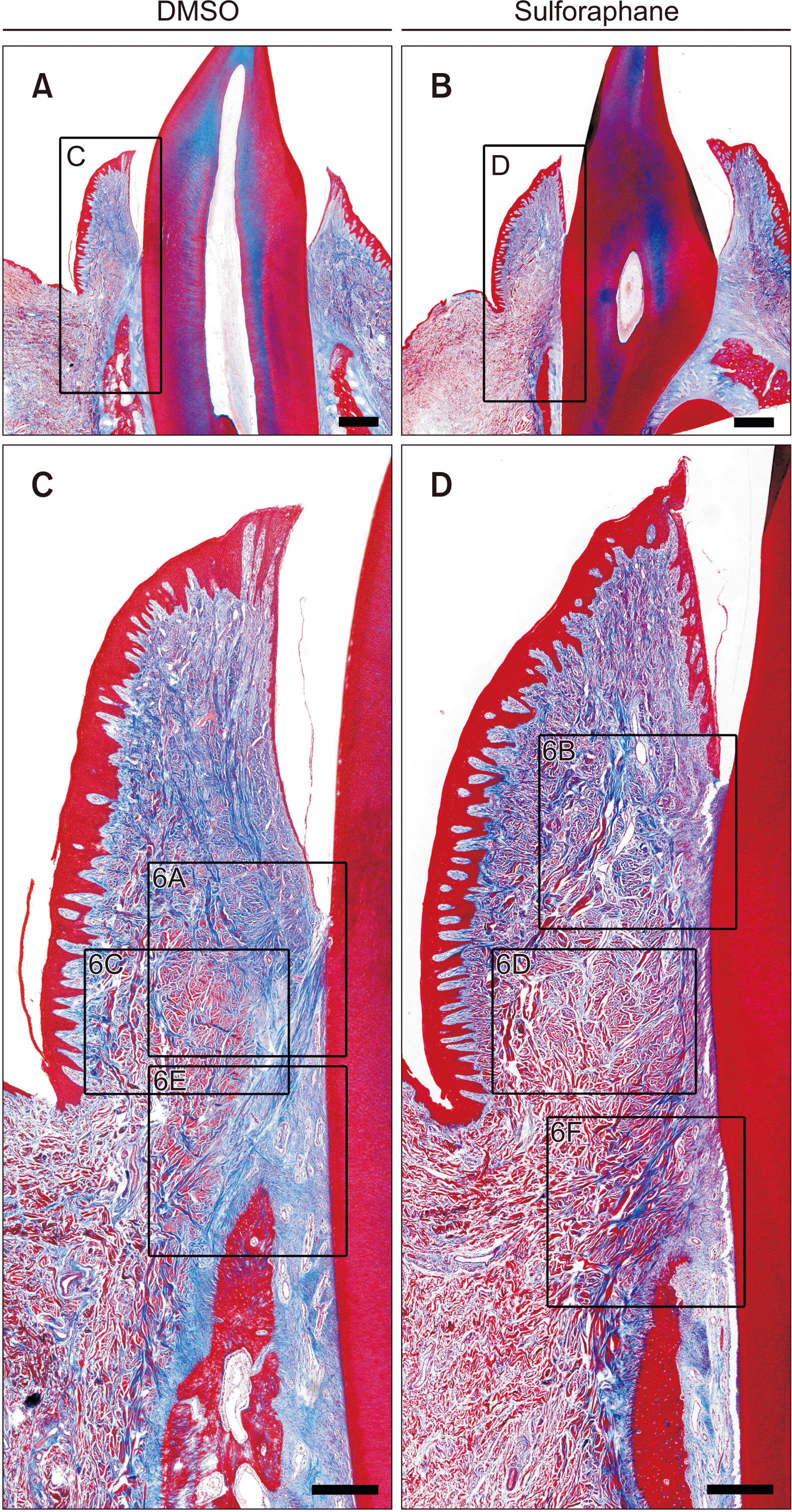
Figure 6
Morphology and arrangement of principal gingival fibers in the buccal supra-alveolar gingiva. The higher magnification views of dentogingival fibers (black arrows indicate dentogingival fibers) and dentoperiosteal fibers (white arrows indicate dentoperiosteal fibers) showed that collagen fiber bundles connected to form the principal gingival fibers were irregularly and not well connected in the sulforaphane (SFN)-treated group compared to the dimethylsulfoxide (DMSO)-treated group. Circular fibers (indicated in black circle dotted lines) of the SFN-treated group were arranged in a thinner and less compact manner compared to the DMSO-treated group. A, C, E, DMSO group. B, D, F, SFN group. Scale bar = 200 μm.
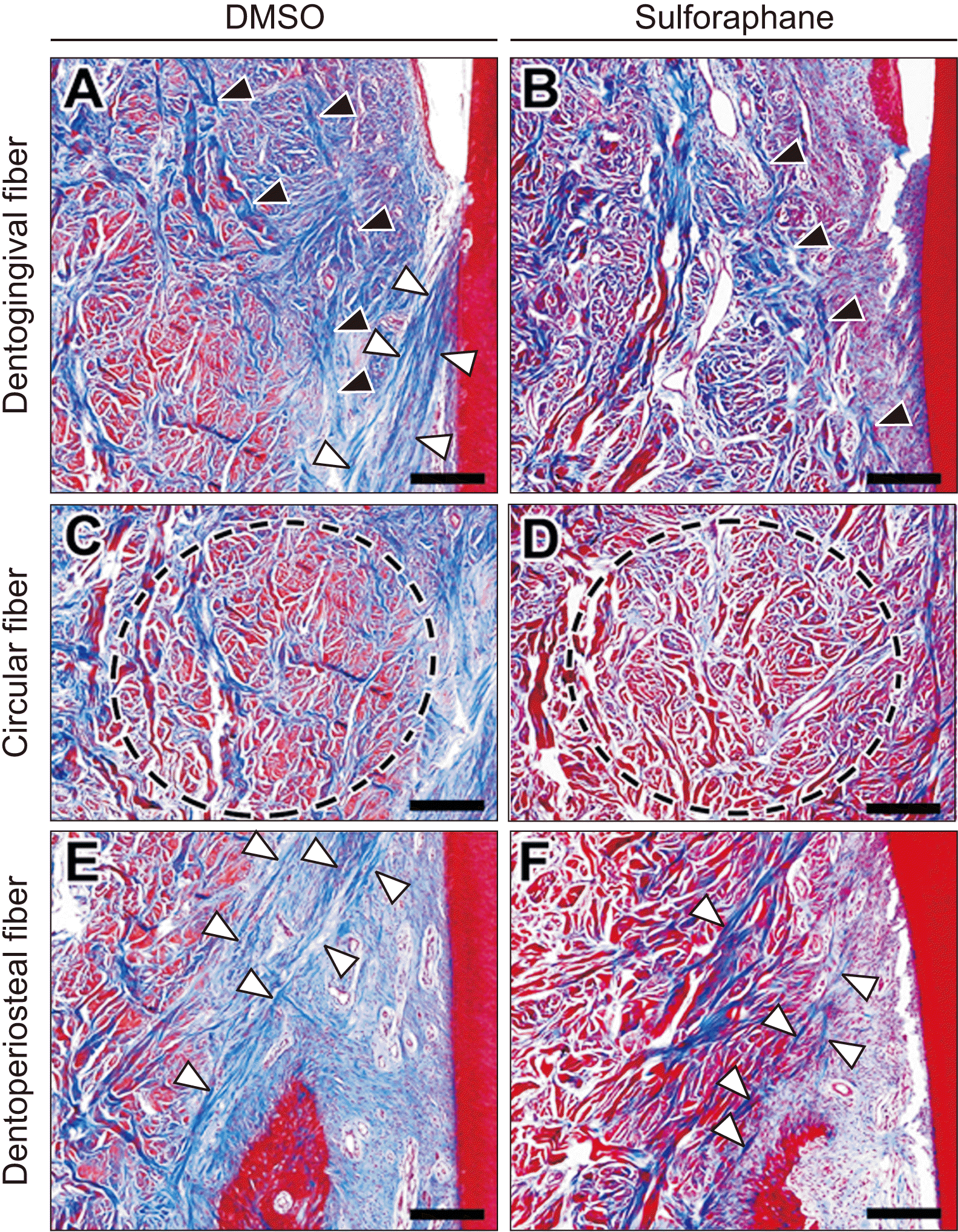
Table 1
Primer sequences used for real-time PCR performed on beagle gingival fibroblasts
Table 2
Statistical analysis of intergroup and intragroup comparisons of the amount and percentage of relapse between control and experimental groups
Group | Degree of rotation (°) | Amount of relapse (°) | Percentage of relapse (%) | |||
---|---|---|---|---|---|---|
|
|
|||||
After 2 weeks | After 4 weeks | After 2 weeks | After 4 weeks | |||
Control | 21.40 ± 5.95 | 13.19 ± 3.88 | 16.96 ± 5.55 | 64.57 ± 18.86 | 81.09 ± 18.80 | |
Experimental | 23.32 ± 2.57 | 6.71 ± 2.99* | 9.19 ± 3.31*,† | 28.01 ± 10.68* | 38.85 ± 11.85*,† |
The symbol (*) indicates statistical significance between the control group and experimental group based on intergroup comparisons (*p < 0.05, paired t-test). The symbol (†) indicates statistical significance of the mean amount and percentage of rotational relapse between 2 and 4 weeks after the retention period based on intragroup comparisons (†p < 0.05, paired t-test).