Abstract
Objective
Methods
References



















Figure 1
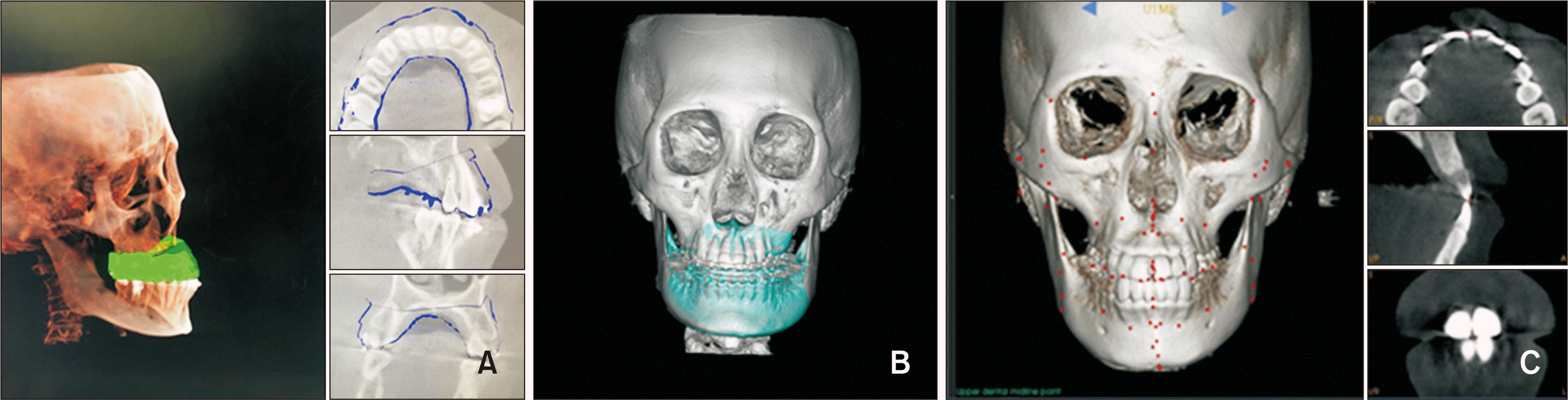
Figure 3
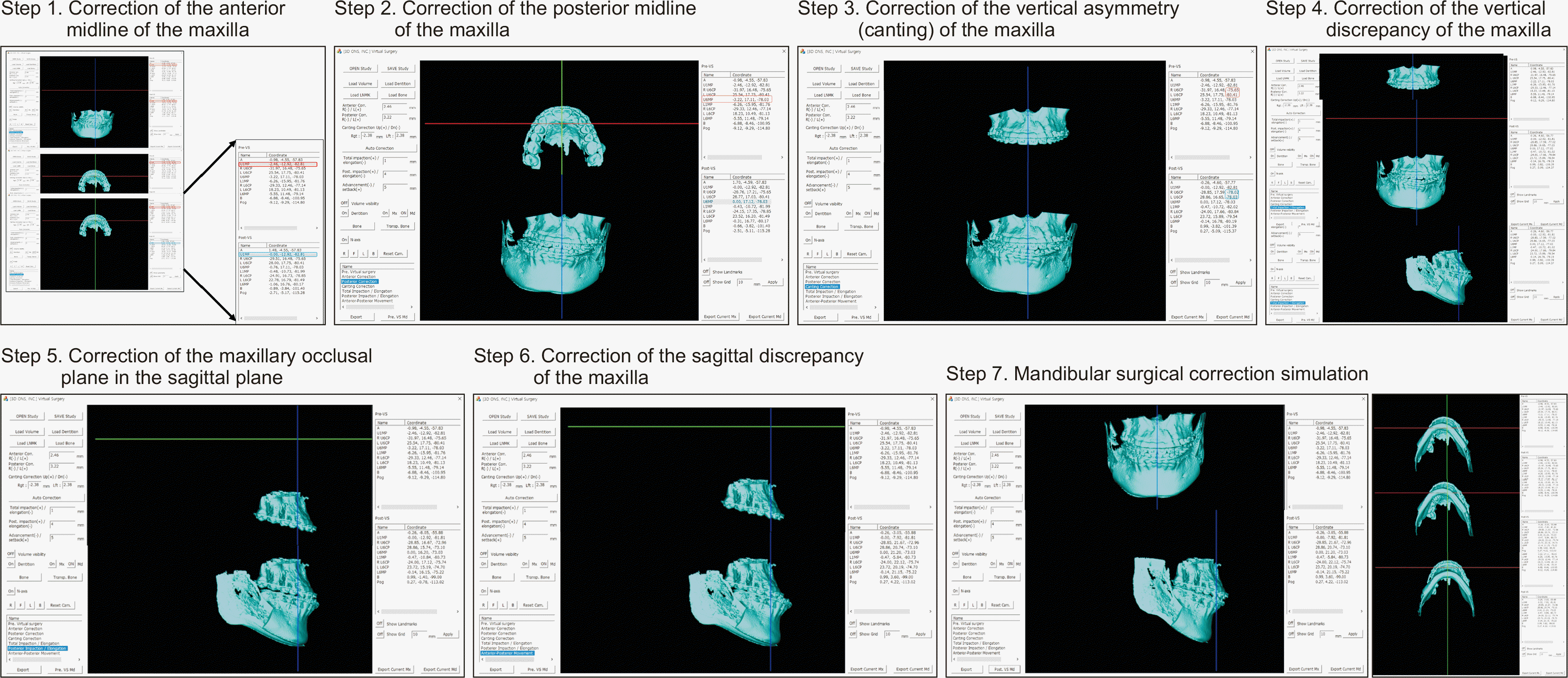
Figure 4
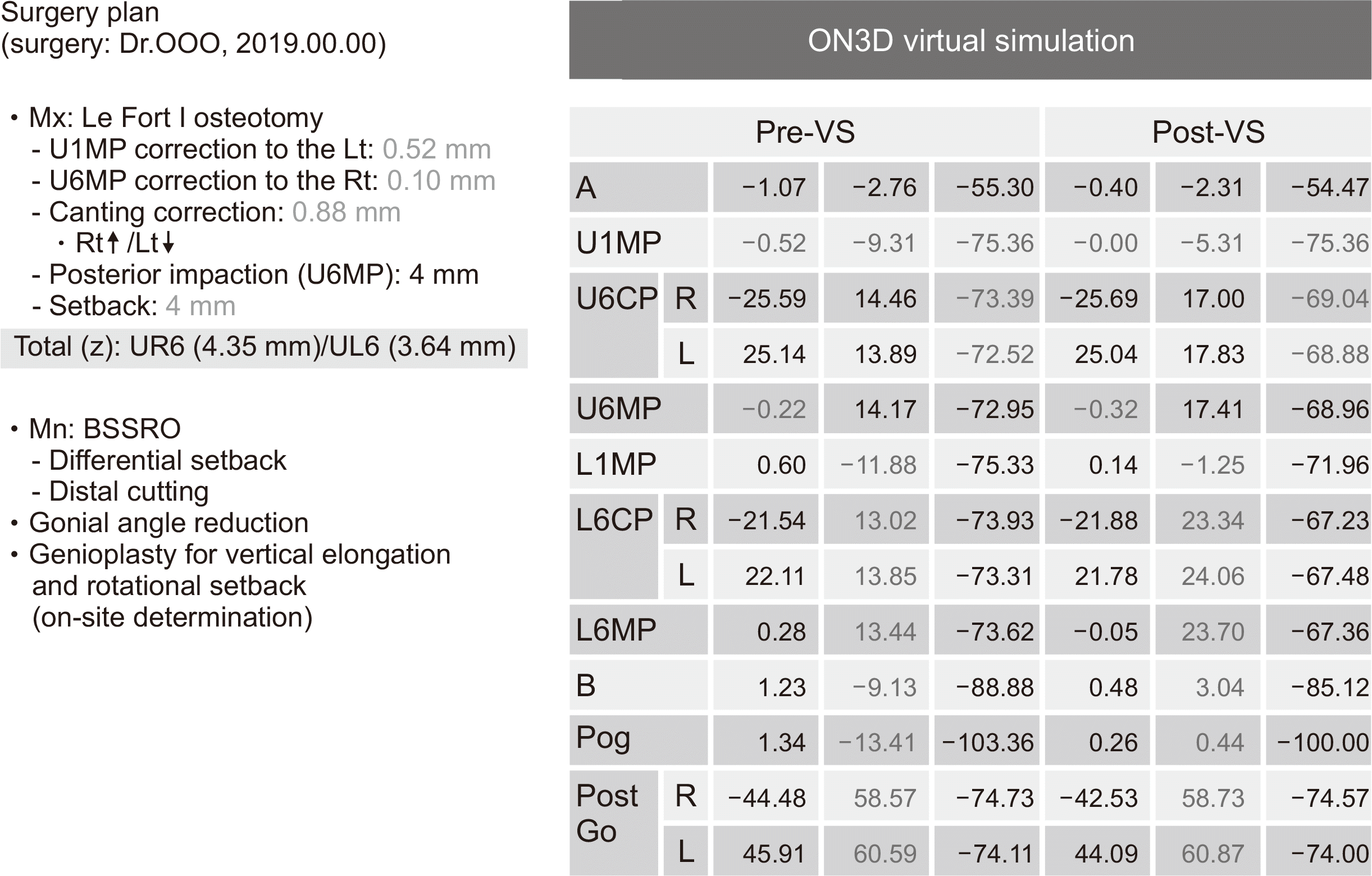
Table 1
Cephalometric variable | T0 stage | T1 stage | p-value | |||
---|---|---|---|---|---|---|
|
|
|||||
Mean | SD | Mean | SD | |||
SNA (°) | 82.3 | 4.4 | 83.2 | 3.5 | 0.246 | |
SNB (°) | 84.3 | 4.2 | 79.8 | 3.8 | 0.000*** | |
ANB (°) | −2.0 | 2.5 | 3.4 | 1.0 | 0.000*** | |
A-N perp (mm) | −0.3 | 4.7 | 1.3 | 2.9 | 0.073 | |
Pog-N perp (mm) | 5.4 | 8.7 | –0.9 | 6.0 | 0.001*** | |
FMA (°) | 25.8 | 7.8 | 29.6 | 5.3 | 0.005** | |
U1 to FH (°) | 112.9 | 6.6 | 106.4 | 4.2 | 0.001** | |
IMPA (°) | 85.4 | 6.7 | 83.1 | 7.3 | 0.110 |
The samples consisted of 15 Korean young adult patients with skeletal Class III malocclusion (9 males and 6 females; mean age, 22.3 years).
SNA, Sella-nasion-point A angle; SNB, sella-nasion-point B angle; ANB, point A-nasion-poing B angle; A-N perp, point A-nasion perpendicular line; Pog-N perp, pogonion-nasion perpendicular line; FMA, Frankfort horizontal plane to mandibular plane angle; U1 to FH, maxillary incisor inclination to Frankfort horizontal plane; IMPA, incisor mandibular plane angle; SD, standard deviation.
Table 2
Surgical movementtypes of the maxilla | Number of patients who underwent the specific type of surgical movement (%) | Virtually planned movement(mm) | Actual movement(mm) | Discrepancy between virtually plannedand actual movement† (mm) | |||||||||
---|---|---|---|---|---|---|---|---|---|---|---|---|---|
|
|
|
|||||||||||
Mean | SD | Range | Mean | SD | Range | Mean | SD | Range | p-value‡ | ||||
Transverse | |||||||||||||
Anterior midline correction | 14/15 (93.3) | 1.38 | 0.99 | 0.13–3.00 | 1.45 | 1.37 | −0.78–4.13 | −0.07 | 0.86 | −1.24–1.15 | |||
Posterior midline correction | 13/15 (86.7) | 1.49 | 1.00 | 0.50–3.22 | 1.53 | 1.51 | −1.08–4.16 | −0.04 | 0.91 | −1.23–1.76 | |||
Sagittal | |||||||||||||
Maxillary advancement | 3/15 (20.0) | 4.33 | 1.53 | 3.00–6.00 | 3.10 | 2.20 | 0.68–4.98 | 1.24 | 0.99 | 0.37–2.32 | |||
Maxillary setback | 10/15 (66.7) | 2.80 | 1.55 | 1.00–5.00 | 2.66 | 1.69 | −0.61–5.50 | 0.14 | 0.78 | −0.75–1.61 | 0.023* | ||
Vertical | |||||||||||||
Maxillary total impaction | 5/15 (33.3) | 1.20 | 0.45 | 0.99–2.00 | 0.75 | 0.76 | −0.37–1.55 | 0.44 | 0.73 | −0.55–1.37 | |||
Maxillary anterior elongation | 6/15 (40.0) | 2.08 | 1.11 | 1.00–4.00 | 2.94 | 1.42 | 1.08–4.34 | −0.85 | 0.77 | −2.15–−0.08 | |||
Maxillary posterior impaction | 12/15 (80.0) | 2.38 | 1.23 | 1.00–5.00 | 1.73 | 1.54 | −1.39–4.45 | 0.65 | 0.79 | −0.65–2.39 | |||
Total number of the specific type of surgical movement | 63 | 0.13 | 0.92 | −2.15–2.39 |
Anterior midline correction, maxillary advancement, maxillary setback, maxillary total impaction, and maxillary anterior elongation were evaluated using U1MP, while posterior midline correction and maxillary posterior impaction were assessed using U6MP.
SD, Standard deviation; U1MP, the midpoint between maxillary central incisors at the occlusal level; U6MP, the midpoint between two mesiobuccal cusp tips of maxillary right and left first molar crowns.
Post-hoc analysis after Kruskal–Wallis test showed a significant difference between the mean discrepancy values of maxillary anterior elongation and maxillary posterior impaction (–0.85 mm [overcorrection] vs. 0.65 mm [undercorrection], *p < 0.05).
Table 3
Surgical movementtypes of the maxilla | Surgical achievement percentage (%) | Absolute difference value | p-value† | ||||||||
---|---|---|---|---|---|---|---|---|---|---|---|
|
|
||||||||||
Mean | SD | Range | p-value* | ≤ 2.00 mm | ≤ 1.00 mm(precision percentage‡) | ||||||
|
|
||||||||||
Number of patient | Percentage | Number of patient | Percentage | ||||||||
Transverse | |||||||||||
Anterior midline correction | 103.4 | 149.3 | −260.0–400.0 | 14/14 | 100.0 | 9/14 | 64.3 | ||||
Posterior midline correction | 95.8 | 112.5 | −158.8–305.0 | 13/13 | 100.0 | 9/13 | 69.2 | ||||
Sagittal | |||||||||||
Maxillary advancement | 65.5 | 37.3 | 22.7–90.8 | 2/3 | 66.7 | 1/3 | 33.3 | ||||
Maxillary setback | 89.8 | 56.4 | −61.0–132.0 | 0.066 | 10/10 | 100.0 | 8/10 | 80.0 | 0.717 | ||
Vertical | |||||||||||
Maxillary total impaction | 63.1 | 70.7 | −37.0–155.0 | 5/5 | 100.0 | 4/5 | 80.0 | ||||
Maxillary anterior elongation | 142.6 | 36.8 | 108.0–207.5 | 5/6 | 83.3 | 3/6 | 50.0 | ||||
Maxillary posterior impaction | 58.9 | 68.3 | −139.0–132.5 | 11/12 | 91.7 | 9/12 | 75.0 | ||||
Total (n=63) | 89.9 | 97.4 | −260.0–400.0 | 60/63 | 95.2 | 43/63 | 68.3 |
Surgical achievement percentage, (amount of movement in actual surgery/amount of movement in virtual surgery) × 100 (%); Precision percentage, [number of precise samples (≤ 1.00 mm)/number of samples] × 100 (%).
Anterior midline correction, maxillary advancement, maxillary setback, maxillary total impaction, and maxillary anterior elongation were evaluated using U1MP, while posterior midline correction and maxillary posterior impaction were assessed using U6MP.
SD, Standard deviation; U1MP, the midpoint between maxillary central incisors at the occlusal level; U6MP, the midpoint between two mesiobuccal cusp tips of maxillary right and left first molar crowns; T1, post-surgery.
‡A discrepancy of less than 1 mm between virtual surgical simulation and T1 landmarks was considered as a precise outcome. Adapted from the article of Choi et al. (Angle Orthod 2009;79:306-311).4