Abstract
Arterial thrombosis and its associated diseases are considered to constitute a major healthcare problem. Arterial thrombosis, defined as blood clot formation in an artery that interrupts blood circulation, is associated with many cardiovascular diseases. Oxidative stress is one of many important factors that aggravates the pathophysiological process of arterial thrombosis. Apurinic/apyrimidinic endonuclease 1/redox factor-1 (Ref-1) has a multifunctional role in cells that includes the regulation of oxidative stress and anti-inflammatory function. The aim of this study was to investigate the therapeutic effect of adenovirus-mediated Ref-1 overexpression on arterial thrombosis induced by 60% FeCl3 solution in rats. Blood flow was measured to detect the time to occlusion, thrombus formation was detected by hematoxylin and eosin staining, reactive oxygen species (ROS) levels were detected by high-performance liquid chromatography, and the expression of tissue factor and other proteins was detected by Western blot. FeCl3 aggravated thrombus formation in carotid arteries and reduced the time to artery occlusion. Ref-1 significantly delayed arterial obstruction via the inhibition of thrombus formation, especially by downregulating tissue factor expression through the Akt-GSK3β-NF-κB signaling pathway. Ref-1 also reduced the expression of vascular inflammation markers ICAM-1 and VCAM-1, and reduced the level of ROS that contributed to thrombus formation. The results showed that adenovirus-mediated Ref-1 overexpression reduced thrombus formation in the rat carotid artery. In summary, Ref-1 overexpression had anti-thrombotic effects in a carotid artery thrombosis model and could be a target for the treatment of arterial thrombosis.
Arterial thrombosis (AT) is the development of a thrombus in an artery. It can affect the cardio-cerebrovascular system. AT is among the top major healthcare problems worldwide. It can be caused by trauma, erosion, or rupture of an atherosclerotic plaque, and by other events that damage the artery surface and lead to blood clotting. An insoluble blood clot becomes a thrombus and may lead to acute myocardial infarction, arterial embolism, ischemic stroke, and other cardiovascular diseases [1,2]. The incidence rates of these conditions are high, as are associated mortality and morbidity rates [3,4]. Drug therapy for the treatment and management of AT has limitations and complications; thus, more ideal treatments for AT are needed.
The coagulation cascade is initiated by tissue factor (TF) upon tissue damage in situations of hemostasis and pathological thrombosis [5-7]. TF, also known as coagulation factor III, is a 47-kD transmembrane glycoprotein. It is a key cofactor of activated coagulation factor VIIa, which forms a complex that activates the whole blood coagulation cascade [8]. In the extrinsic coagulation pathway initiated by TF, prothrombin is converted to thrombin, which causes cellular responses such as fibrin formation, platelet activation, and thrombus formation [9]. Under pathological conditions, TF is released mainly by monocytes, neutrophils, and vascular endothelial cells; this release initiates life-threatening thrombosis in the context of sepsis, tumors, and atherosclerosis [10,11]. TF expression is regulated primarily by the phosphoinositide 3-kinase (PI3K)/Akt, glycogen synthase kinase 3 beta (GSK3β), and nuclear factor (NF)-κB signaling pathways [5,12,13]. The PI3K/Akt pathway regulates TF expression negatively through site-specific phosphorylation of GSK3β, and TF is regulated positively via activation of the NF-κB pathway. Thus, inhibition of the TF pathway via modulation of these interrelated signaling pathways is an effective strategy for the prevention of AT [14].
Endothelial (vascular) dysfunction is an early correlate of coronary artery disease in humans. It is also found in the context of severe inflammatory conditions [15]. Numerous studies have shown that inflammation is associated with an increased risk of cardiovascular disease [16-18]. Furthermore, these studies have provided substantial evidence for close relationships among inflammation, reactive oxygen species (ROS), and redox signaling. Oxidative stress and inflammation are also implicated in platelet activation and thrombus development [19]. ROS have been reported to stimulate a procoagulant state via TF expression [20].
Apurinic/apyrimidinic endonuclease 1/redox factor-1 (APE1/Ref-1, henceforth referred to as Ref-1) is a multifunctional protein that is secreted from stimulated cells and has multiple roles. In addition to its anti-inflammatory function, it functions as an apurinic/apyrimidinic endonuclease in the DNA base repair pathway [21]. It modulates redox status by controlling the cellular responses to oxidative stress via the reduction of ROS production [22]. Recombinant human Ref-1 protein has been shown to inhibit proinflammatory cytokines and inflammation induced by tumor necrosis factor alpha (TNF-β) or lipopolysaccharide in endothelial cells and mice [23-25]. As chronic vascular inflammation is a key factor in the pathogenesis of various vascular diseases and in inflammatory pathways that promote thrombosis [26], the regulation of inflammatory reactions in the vascular endothelium may be a target for the treatment of thrombosis. Ref-1 could be a target for the treatment of AT, given its anti-inflammatory and anti-oxidative properties. However, whether Ref-1 inhibits AT or regulates TF-related signaling pathways in arterial vascular tissues is not known. Thus, in this study, we investigated the effect of adenovirus-mediated Ref-1 overexpression on carotid artery thrombosis and TF expression in a mouse model of thrombosis induced by treatment with FeCl3 solution. The possible role of the PI3K/Akt-GSK3β–NF-κB signaling pathway in AT was explored.
Male Sprague-Dawley rats (Samtako, Osan, Korea) aged 8–10 weeks with body weights of 250–280 g were used in this study. All experimental procedures adhered to the animal care and use policies of Chungnam National University (CNUH-017-P0069). The animals were housed in a standard environment with a 12/12-h light/dark cycle, a constant room temperature of 20°C–25°C, and 40%–60% humidity. Food and water were supplied ad libitum.
2,2,2-Tribromoethanol, urethane, 2-methyl-2-butanol, dihydroethidium (DHE), nitrosodisulfonate (NDS), diethylenetriaminepentaacetic acid, hydroethidine, heparin, and acetonitrile were purchased from Sigma-Aldrich (St. Louis, MO, USA). SurgLide transparent gel was purchased from Bio-Chem Laboratories Inc. (Los Angeles, CA, USA).
Adenovirus encoding β-galactosidase (adβ-gal) and full-length APE1/Ref-1 (adRef-1) were prepared. The carotid artery was infected with adenovirus using a previously described method with little modification [27-29]. Briefly, the rats were anesthetized by intraperitoneal injection of 0.25 g/kg 2,2,2-tribromoethanol. Following exposure of the left carotid artery by incision, artery was temporarily ligated by double approximator microvascular clamp (S&T AG, Neuhausen, Switzerland). Isolated arterial segments were washed once with saline followed by infection with saline or 1 × 108 pfu of adβ-gal or adRef-1 adenovirus and were incubated for 20 min. After incubation, temporarily ligated segments were washed again with saline and blood flow was recovered by release of microvascular clamp. The midline incision was closed with sutures and subsequent experiments were performed 24 h later.
The FeCl3 thrombosis model was modified based on previous reports [30,31]. Briefly, rats were anesthetized by intraperitoneal injection of 1.2 g/kg urethane 24 h after adenoviral infection. The carotid arteries were isolated. A TS420 perivascular flow module and nanoprobes (Transonic Systems Inc., Ithaca, NY, USA) were used to measure arterial blood flow. The signals were recorded constantly by a PowerLab 8/35 device (AD Instruments Ltd., Dunedin, New Zealand). The cavity between the artery and probe was filled with transparent gel, and injury was induced to external surface of the artery by attaching 1 × 2 mm filter paper soaked with 60% FeCl3 solution for 5 min. The time to occlusion (TTO), defined as the time at which no blood flow had been detected for > 5 min, was determined. The recording time was limited to 60 min.
The following antibodies were used: Anti–Ref-1, anti–intercellular adhesion molecule-1 (ICAM-1), anti–vascular cell adhesion molecule-1 (VCAM-1), anti–P-65, anti–T-65, and anti-TF antibodies from Santa Cruz Biotechnology (Santa Cruz, CA, USA). Anti–total GSK3β antibody from Abcam (Cambridge, UK). Anti–β-actin, anti–P-GSK3β, anti–P-Akt and anti–T-Akt antibodies from Cell Signaling Technology (Danvers, MA, USA). Four hours after FeCl3 injury, the arterial segments were harvested for immunoblotting. The segments were homogenized with RIPA buffer and incubated at 4°C for 1 h. Then, the homogenates were centrifuged at 12,000 g, 4°C for 30 min. The supernatant was collected and 30 μg lysate was used for immunoblotting with the appropriate primary and secondary antibodies. The chemiluminescent signal was developed using SuperSignal West Pico or Femto substrate (Pierce Biotechnology, Rockford, IL, USA). Values were normalized to a β-actin loading control.
Hematoxylin and eosin (H&E) staining was performed following a standard procedure. Eight minutes after FeCl3 treatment, the arteries were fixed in 10% formalin for 24 h at 4°C, then washed and embedded in paraffin. The paraffinized segments were sectioned, deparaffinized, rehydrated, and stained with H&E. The sections were digitally imaged under a microscope (BA210 Series; Motic, Richmond, BC, Canada).
2-hydroxyethidium (2-EOH) was used to measure arterial superoxide production. High-performance liquid chromatography (HPLC) was used to detect 2-EOH, which is the oxidative form of DHE. For the standard, 2-EOH was made from NDS using a previously described method [32]. The arterial segments were excised 5 min after FeCl3 injury and washed with Krebs-HEPES buffer. They were then incubated with 50 μM DHE at 37°C for 30 min, washed again with Krebs-HEPES buffer at 37°C for 1 h, and homogenized with acetonitrile. The samples were centrifuged at 12,000 g, 4°C for 30 min. The supernatant was used for the HPLC assay, and the precipitate was lysed with NaOH to measure the protein concentration. 2-EOH was measured using a 1290 series device (Agilent technologies, Santa Clara, CA, USA) with a 120 Sb-C18 Poroshell column, 580 nm emission, and 480 nm excitation. The composition of the mobile phase was 37% acetonitrile and 0.1% trifluoroacetic acid. The flow rate of the mobile phase was 0.5 ml/min.
To induce Ref-1 overexpression in the carotid artery, adenovirus-mediated gene delivery was used. Left carotid artery was exposed and the adenovirus encoded with β-gal or Ref-1 was introduced into the artery. The artery was incubated with the adenovirus for 24 h, then denuded. The infection site was exposed to 60% FeCl3 solution for 5 min. The blood flow was measured for 1 h. Four hours after thrombus formation, the artery was harvested for further experiments (Fig. 1A). The expression of Ref-1 was significantly higher in the AdRef-1–treated group than in the Adβ-gal–treated group (Fig. 1B and C). This result confirms that Ref-1 adenovirus-mediated gene delivery resulted in overexpression of the Ref-1 protein in the carotid artery.
Following Ref-1 overexpression, FeCl3-soaked filter paper was attached to the infection site to initiate AT. The blood flow was then measured until occlusion. The TTO was defined as the time required for blood flow to cease entirely for > 5 min. The average TTO after FeCl3 exposure was 20 min in the control (Adβ-gal–treated) group; occlusion was delayed significantly (TTO = ~80 min) or did not occur in the AdRef-1–treated group (Fig. 1D and E).
H&E staining showed that the endothelial surface of the carotid artery was arranged in an orderly manner, with no thrombus formation in the artery lumen or inflammatory cell infiltration in the sham group. Carotid artery thrombosis was observed as compact red and white thrombus formation with thrombosis and the vascular wall connected to form a visible platelet trabecular. Most vascular endothelial cells exhibited thrombosis with a vascular wall surrounded by inflammatory cell infiltration. Adenovirus treatment alone did not result in AT formation, with outcomes similar to those in the sham group (data not shown). However, Adβ-gal + FeCl3 treatment resulted in the formation of thrombi that occupied almost 80% of the artery lumen and interrupted blood flow. In contrast, lumen treated with AdRef-1 + FeCl3 showed 50% occlusion by thrombi with reduced number of vascular endothelial cells at the surface and white blood cell adhesion and infiltration (Fig. 1F and G).
The PI3K/Akt signaling pathway negatively regulates TF expression [33-35]. We sought to investigate whether Ref-1 could regulate TF via the PI3K/Akt pathway. Ref-1 overexpression significantly increased Akt phosphorylation and the phosphorylation of GSK3β, a major downstream regulator of Akt (Fig. 2A and B). These results indicate that Ref-1 inhibited GSK3β activation by triggering its phosphorylation at the serine 9 phosphorylation site, which is related to GSK3β inactivation. GSK3β activation is required for the regulation of NF-κB activation [5,36]. Thus, we next examined the phosphorylation of p65. Ref-1 overexpression inhibited p65 phosphorylation (Fig. 2C and D). It also reduced TF expression (Fig. 2C and D), confirming that Ref-1 inhibits TF expression through PI3K/Akt signaling via the suppression of GSK3β activity.
TF expression is mainly regulated by TF pathway inhibitor (TFPI). TFPI is a single-chain polypeptide, which can reversibly inhibit Factor Xa. While Xa is inhibited, the Xa-TFPI complex can subsequently also inhibit the Factor VIIa-TF complex. In addition, thrombomodulin is an integral membrane protein expressed on the surface of endothelial cells, which serves as a cofactor for thrombin. It reduces blood coagulation by converting thrombin to an anticoagulant enzyme from a procoagulant enzyme. It also protects endothelial cells and vasculature by depressing inflammatory injuries. Therefore, we measured the protein expressions of both TFPI and thrombomodulin in the thrombotic carotid arteries as well as examined the effect of Ref-1 overexpression. As shown in Fig. 2E and F, Ref-1 overexpression increases the protein expressions of TFPI and thrombomodulin that were reduced by FeCl3 treatment.
Endothelial cell activation has been associated with the initiation of inflammation. It is identified via the expression of adhesion/inflammatory molecules, such as VCAM-1 and ICAM-1. An increase in VCAM-1 and ICAM-1 surface protein expression leads to an increase in vascular inflammation and the initiation of atherosclerotic plaque formation [37,38]. Thus, we examined the effect of Ref-1 overexpression on the expression of VCAM-1 and ICAM-1 in thrombotic carotid arteries. FeCl3 treatment significantly elevated VCAM-1 and ICAM-1 protein expression in the Adβ-gal–treated group (Fig. 3A and B). As expected, the expression of VCAM-1 and ICAM-1 was lesser in the AdRef-1 + FeCl3–treated group. This result suggests that Ref-1 inhibits endothelial activation.
Oxidative stress plays an important role in the development and progression of cardiovascular diseases. The majority of cardiovascular diseases are characterized by an imbalance between ROS formation and removal by cell antioxidant systems [39], which leads to the accumulation of superoxide, hydrogen peroxide, or peroxynitrite and results in loss of the steady state [40]. ROS participate directly in the regulation of platelet activation and thrombus formation [41]. Ref-1 is well known for its redox activity and antioxidant function. Thus, we measured ROS levels by quantifying the degree of DHE staining in arterial segments after FeCl3 injury with or without Ref-1 overexpression. DHE can freely permeate cell membrane and be oxidized by cellular O2•- to produce two red fluorescent products, namely ethidium, which is typically formed by a non-specific redox reaction, and 2-EOH, a specific adduct of cellular O2•-. 2-EOH can be readily separated from its parent DHE by performing HPLC and the 2-EOH peak can then be used to estimate the intercellular production of O2•-. As shown in Fig. 4A and B, the 2-EOH peak was considerably reduced by Ref-1 overexpression.
Vascular endothelial injury caused by inflammation is a key factor in the initiation of atherosclerosis and AT, as demonstrated in numerous studies [42-44]. Despite much research, however, the pathological mechanisms of AT are not completely understood, resulting in a lack of clinically safe and effective drug treatments. Under normal physiological conditions, vascular endothelial cells inhibit platelet aggregation, stimulate fibrinolysis, and inhibit abnormal coagulation. However, vascular endothelial cell activation stimulates platelet aggregation and inhibits the dissolution of fibrin, ultimately leading to abnormal luminal coagulation and thrombus formation [44]. To prevent and cure AT, the regulation of endothelial function, improvement of the coagulation balance, and alleviation of inflammation are thus essential. In this study, we used FeCl3 to induce carotid artery thrombosis. This model causes severe endothelial cell injury due to oxidative stress and increases TF expression in endothelial cells and leukocytes [45]. Thrombus formation achieved by this method is similar to clinical spontaneous thrombosis. Thus, this model has been used widely in the preclinical evaluation of thrombolytic drugs and related research [46].
Ref-1 is a protein with multiple functions. In addition to base-excision DNA repair and the transcriptional regulation of gene expression, it plays a significant role in the control of the cellular response to oxidative stress. In particular, Ref-1 reduces the intracellular production of ROS [47-49]. Ref-1 overexpression has been shown to suppress monocyte adhesion to endothelial cells induced by TNF-α [50] and to inhibit hypoxia-induced apoptosis of endothelial cells [51]. In addition, Ref-1 inhibited neointimal formation caused by balloon injury in rats [52]. These findings suggest that Ref-1 plays a protective role in the context of inflammatory vascular disease. However, no report has indicated that Ref-1 inhibits AT or modulates TF-related signaling pathways in arterial vascular tissues or endothelium. In this study, Ref-1 significantly improved blood flow in the carotid artery, delayed occlusion, and alleviated thrombosis, which is the first evidence that Ref-1 can inhibit AT (Fig. 1D–G).
Our findings demonstrate that the inhibition of TF expression in endothelial cells by Ref-1 occurs through the activation of TFPI, thrombomodulin, Akt/GSK3β signaling pathway and inhibition of the NF-κB signaling pathway (Fig. 2A–F). TF expression has been shown to be regulated primarily by the TFPI, NF-κB and PI3K/Akt signaling pathways [12,13,53,54]. NF-κB is a homo- or heterodimeric complex of proteins. The p65 heterodimer is involved primarily in the regulation of TF gene expression. In non-stimulated conditions, inactive NF-κB is localized in the cytoplasm, bound to IκB. Upon NF-κB activation, it dissociates from IκB, and the subsequent degradation of IκB proteins causes NF-κB dimers to enter the nucleus and induce TF gene expression [55]. The PI3K/Akt pathway is composed of a family of signal transduction enzymes that participate in the regulation of cellular functions such as cell proliferation and survival. PI3K/Akt is known to be a negative-feedback regulator of TF expression [34]. In addition, TFPI is a potent anticoagulant protein in the extrinsic coagulation pathway. It is a major physiological inhibitor of the coagulation initiation phase, acting as an inhibitor of TF-FVIIa complex [56]. Therefore, FeCl3 induced inhibition of TFPI in our study would allow coagulation process and enhancement of arterial thrombosis generation. Ref-1 overexpression increases TFPI protein levels that could in turn reduce TF-induced coagulation (Fig. 2E and F). Furthermore, thrombomodulin that is present on the endothelium throughout the vascular system is a vasculoprotective integral membrane glycoprotein, which is a crucial determinant of thromboresistance of ECs through anti-fibrinolytic, anti-coagulant, and anti-inflammatory effects [57]. It plays a central role in protection against excessive coagulation via activation of protein C and subsequent inactivation of coagulation factors V and VIII. FeCl3 injury reduces thrombomodulin expression. However, thrombomodulin increment following Ref-1 overexpression could be protective in terms of thrombotic events (Fig. 2E and F).
Previous studies have shown that the PI3K/Akt/NF-κB transduction pathways play a critical role in the induction of VCAM-1 and ICAM-1 expression [37]. Endothelial adhesion molecules such as VCAM-1 and ICAM-1, together with other important factors such as chemoattractants, play crucial roles in the homing of monocytes to vascular lesion sites. Significant modulation of ICAM-1 expression over time was observed in apoE-/- mice compared with that in wild-type mice [38]. Similarly, VCAM-1 expression has been identified as a very early event in the development of atherosclerotic lesions in experimental animal models [58]. In this study, Ref-1 abrogated the increased expression of VCAM-1 and ICAM-1 induced by FeCl3 injury (Fig. 3).
ROS are highly reactive metabolites that can damage various cellular constituents and are generated in vivo in several pathological conditions [59-61]. Many studies have demonstrated that ROS plays a pivotal role in the development of cardiovascular pathophysiology. Specifically, the restoration of blood flow after a period of ischemia was shown to generate an ROS surge [62]. Interestingly, ROS was also shown to promote thrombosis by inducing TF expression [60,63]. In this study, FeCl3 caused an increase in the ROS level, which was attenuated by Ref-1 (Fig. 4).
Taken together, the findings of this study reveal that Ref-1 plays a key role in the modulation of TF expression via the Akt/GSK3β/NF-κB signaling pathway, TFPI and thrombomodulin (Fig. 5). Ref-1 increases blood flow, reduces thrombus formation, and reduces oxidative stress in the carotid artery. These results provide evidence that Ref-1 plays an important role in AT. The present study might not be a confirmative but rather a preliminary study for the possibility that Ref-1 may be a new target for the treatment of AT and many other thrombotic diseases.
ACKNOWLEDGEMENTS
This work was supported by the Basic Science Research Program through the National Research Foundation of Korea (NRF) funded by the Ministry of Education (NRF-2014R1A6A1029617 and NRF-2019R1I1A3A0105949712) and by the research fund of Chungnam National University.
Notes
REFERENCES
1. Keenan NG, Sheppard MN, Nott DM, Pennell DJ, Mohiaddin RH. 2009; Carotid plaque rupture. Lancet. 374:1703. DOI: 10.1016/S0140-6736(09)60291-9. PMID: 19914514.


2. Blanco M, Sobrino T, Montaner J, Medrano V, Jiménez C, Masjuán J, Gómez-Escalonilla C, de Luis P, Arboix A, Castillo J. MITICO Study. 2010; Stroke with polyvascular atherothrombotic disease. Atherosclerosis. 208:587–592. DOI: 10.1016/j.atherosclerosis.2009.07.041. PMID: 19695570.


3. Santarius T, Menon DK. 2003; Images in clinical medicine. Carotid-artery thrombosis secondary to basal skull fracture. N Engl J Med. 349:e5. DOI: 10.1056/ENEJMicm020664. PMID: 12890856.
4. Stoll G, Kleinschnitz C, Nieswandt B. 2008; Molecular mechanisms of thrombus formation in ischemic stroke: novel insights and targets for treatment. Blood. 112:3555–3562. DOI: 10.1182/blood-2008-04-144758. PMID: 18676880.


5. Zhang W, Wang J, Wang H, Tang R, Belcher JD, Viollet B, Geng JG, Zhang C, Wu C, Slungaard A, Zhu C, Huo Y. 2010; Acadesine inhibits tissue factor induction and thrombus formation by activating the phosphoinositide 3-kinase/Akt signaling pathway. Arterioscler Thromb Vasc Biol. 30:1000–1006. DOI: 10.1161/ATVBAHA.110.203141. PMID: 20185792. PMCID: PMC3626455.



6. Li YD, Ye BQ, Zheng SX, Wang JT, Wang JG, Chen M, Liu JG, Pei XH, Wang LJ, Lin ZX, Gupta K, Mackman N, Slungaard A, Key NS, Geng JG. 2009; NF-kappaB transcription factor p50 critically regulates tissue factor in deep vein thrombosis. J Biol Chem. 284:4473–4483. DOI: 10.1074/jbc.M806010200. PMID: 19095643. PMCID: PMC2640971.
7. Johnson GJ, Leis LA, Bach RR. 2009; Tissue factor activity of blood mononuclear cells is increased after total knee arthroplasty. Thromb Haemost. 102:728–734. DOI: 10.1160/TH09-04-0261. PMID: 19806259.


8. Ruf W. 2012; Role of thiol pathways in TF procoagulant regulation. Thromb Res. 129 Suppl 2:S11–S12. DOI: 10.1016/j.thromres.2012.02.020. PMID: 22401798. PMCID: PMC3393083.


9. Evans CH. 1991; The role of proteinases in cartilage destruction. Agents Actions Suppl. 32:135–152. DOI: 10.1007/978-3-0348-7405-2_19. PMID: 2069082.


10. Li S, Chen H, Ren J, Geng Q, Song J, Lee C, Cao C, Zhang J, Xu N. 2014; MicroRNA-223 inhibits tissue factor expression in vascular endothelial cells. Atherosclerosis. 237:514–520. DOI: 10.1016/j.atherosclerosis.2014.09.033. PMID: 25463083.


11. Dong R, Chen W, Feng W, Xia C, Hu D, Zhang Y, Yang Y, Wang DW, Xu X, Tu L. 2015; Exogenous bradykinin inhibits tissue factor induction and deep vein thrombosis via activating the eNOS/phosphoinositide 3-kinase/Akt signaling pathway. Cell Physiol Biochem. 37:1592–1606. DOI: 10.1159/000438526. PMID: 26517864.


12. Schabbauer G, Tencati M, Pedersen B, Pawlinski R, Mackman N. 2004; PI3K-Akt pathway suppresses coagulation and inflammation in endotoxemic mice. Arterioscler Thromb Vasc Biol. 24:1963–1969. DOI: 10.1161/01.ATV.0000143096.15099.ce. PMID: 15319270.


13. Guha M, Mackman N. 2002; The phosphatidylinositol 3-kinase-Akt pathway limits lipopolysaccharide activation of signaling pathways and expression of inflammatory mediators in human monocytic cells. J Biol Chem. 277:32124–32132. DOI: 10.1074/jbc.M203298200. PMID: 12052830.


14. Winckers K, ten Cate H, Hackeng TM. 2013; The role of tissue factor pathway inhibitor in atherosclerosis and arterial thrombosis. Blood Rev. 27:119–132. DOI: 10.1016/j.blre.2013.03.001. PMID: 23631910.


15. Becker L, Prado K, Foppa M, Martinelli N, Aguiar C, Furian T, Clausell N, Rohde LE. 2012; Endothelial dysfunction assessed by brachial artery ultrasound in severe sepsis and septic shock. J Crit Care. 27:316.e9–316.e14. DOI: 10.1016/j.jcrc.2011.08.002. PMID: 22172795.


16. Karbach S, Wenzel P, Waisman A, Munzel T, Daiber A. 2014; eNOS uncoupling in cardiovascular diseases--the role of oxidative stress and inflammation. Curr Pharm Des. 20:3579–3594. DOI: 10.2174/13816128113196660748. PMID: 24180381.

17. Steven S, Münzel T, Daiber A. 2015; Exploiting the pleiotropic antioxidant effects of established drugs in cardiovascular disease. Int J Mol Sci. 16:18185–18223. DOI: 10.3390/ijms160818185. PMID: 26251902. PMCID: PMC4581241.



18. Daiber A, Steven S, Weber A, Shuvaev VV, Muzykantov VR, Laher I, Li H, Lamas S, Münzel T. 2017; Targeting vascular (endothelial) dysfunction. Br J Pharmacol. 174:1591–1619. DOI: 10.1111/bph.13517. PMID: 27187006. PMCID: PMC5446575.


19. Steven S, Frenis K, Oelze M, Kalinovic S, Kuntic M, Bayo Jimenez MT, Vujacic-Mirski K, Helmstädter J, Kröller-Schön S, Münzel T, Daiber A. 2019; Vascular Inflammation and oxidative stress: major triggers for cardiovascular disease. Oxid Med Cell Longev. 2019:7092151. DOI: 10.1155/2019/7092151. PMID: 31341533. PMCID: PMC6612399.


20. Cimmino G, Cirillo P, Ragni M, Conte S, Uccello G, Golino P. 2015; Reactive oxygen species induce a procoagulant state in endothelial cells by inhibiting tissue factor pathway inhibitor. J Thromb Thrombolysis. 40:186–192. DOI: 10.1007/s11239-015-1199-1. PMID: 25712553.


21. Lee YR, Joo HK, Jeon BH. 2020; The biological role of apurinic/apyrimidinic endonuclease1/redox factor-1 as a therapeutic target for vascular inflammation and as a serologic biomarker. Biomedicines. 8:57. DOI: 10.3390/biomedicines8030057. PMID: 32164272. PMCID: PMC7148461.


22. Hao J, Du H, Liu F, Lu JC, Yang XC, Cui W. 2019; Apurinic/apyrimidinic endonuclease/redox factor 1 (APE1) alleviates myocardial hypoxia-reoxygenation injury by inhibiting oxidative stress and ameliorating mitochondrial dysfunction. Exp Ther Med. 17:2143–2151. DOI: 10.3892/etm.2019.7212. PMID: 30867702. PMCID: PMC6395998.



23. Park MS, Choi S, Lee YR, Joo HK, Kang G, Kim CS, Kim SJ, Lee SD, Jeon BH. 2016; Secreted APE1/Ref-1 inhibits TNF-α-stimulated endothelial inflammation via thiol-disulfide exchange in TNF receptor. Sci Rep. 6:23015. DOI: 10.1038/srep23015. PMID: 26964514. PMCID: PMC4786854.


24. Joo HK, Lee YR, Lee EO, Park MS, Choi S, Kim CS, Park JB, Jeon BH. 2019; The extracellular role of Ref-1 as anti-inflammatory function in lipopolysaccharide-induced septic mice. Free Radic Biol Med. 139:16–23. DOI: 10.1016/j.freeradbiomed.2019.05.013. PMID: 31100475.


25. Lee HM, Jeon BH, Won KJ, Lee CK, Park TK, Choi WS, Bae YM, Kim HS, Lee SK, Park SH, Irani K, Kim B. 2009; Gene transfer of redox factor-1 inhibits neointimal formation: involvement of platelet-derived growth factor-beta receptor signaling via the inhibition of the reactive oxygen species-mediated Syk pathway. Circ Res. 104:219–227. 5p following 227DOI: 10.1161/CIRCRESAHA.108.178699. PMID: 19038866.

26. Libby P. 2002; Inflammation in atherosclerosis. Nature. 420:868–874. DOI: 10.1038/nature01323. PMID: 12490960.


27. Perlman H, Luo Z, Krasinski K, Le Roux A, Mahfoudi A, Smith RC, Branellec D, Walsh K. 1999; Adenovirus-mediated delivery of the Gax transcription factor to rat carotid arteries inhibits smooth muscle proliferation and induces apoptosis. Gene Ther. 6:758–763. DOI: 10.1038/sj.gt.3300893. PMID: 10505098.


28. Jeon BH, Gupta G, Park YC, Qi B, Haile A, Khanday FA, Liu YX, Kim JM, Ozaki M, White AR, Berkowitz DE, Irani K. 2004; Apurinic/apyrimidinic endonuclease 1 regulates endothelial NO production and vascular tone. Circ Res. 95:902–910. DOI: 10.1161/01.RES.0000146947.84294.4c. PMID: 15472121.

29. Lee SK, Chung JI, Park MS, Joo HK, Lee EJ, Cho EJ, Park JB, Ryoo S, Irani K, Jeon BH. 2011; Apurinic/apyrimidinic endonuclease 1 inhibits protein kinase C-mediated p66shc phosphorylation and vasoconstriction. Cardiovasc Res. 91:502–509. DOI: 10.1093/cvr/cvr095. PMID: 21467074. PMCID: PMC3139447.



30. Kurz KD, Main BW, Sandusky GE. 1990; Rat model of arterial thrombosis induced by ferric chloride. Thromb Res. 60:269–280. DOI: 10.1016/0049-3848(90)90106-M. PMID: 2087688.


31. Robinson MA, Welsh DC, Bickel DJ, Lynch JJ, Lyle EA. 2003; Differential effects of sodium nitroprusside and hydralazine in a rat model of topical FeCl3-induced carotid artery thrombosis. Thromb Res. 111:59–64. DOI: 10.1016/j.thromres.2003.08.012. PMID: 14644081.

32. Zielonka J, Hardy M, Kalyanaraman B. 2009; HPLC study of oxidation products of hydroethidine in chemical and biological systems: ramifications in superoxide measurements. Free Radic Biol Med. 46:329–338. DOI: 10.1016/j.freeradbiomed.2008.10.031. PMID: 19026738. PMCID: PMC3375818.


33. Di Santo A, Amore C, Dell'Elba G, Manarini S, Evangelista V. 2011; Glycogen synthase kinase-3 negatively regulates tissue factor expression in monocytes interacting with activated platelets. J Thromb Haemost. 9:1029–1039. DOI: 10.1111/j.1538-7836.2011.04236.x. PMID: 21320285. PMCID: PMC3091995.



34. Breitenstein A, Camici GG, Tanner FC. 2009; Tissue factor: beyond coagulation in the cardiovascular system. Clin Sci. 118:159–172. DOI: 10.1042/CS20080622. PMID: 19845509.


35. Eisenreich A, Malz R, Pepke W, Ayral Y, Poller W, Schultheiss HP, Rauch U. 2009; Role of the phosphatidylinositol 3-kinase/protein kinase B pathway in regulating alternative splicing of tissue factor mRNA in human endothelial cells. Circ J. 73:1746–1752. DOI: 10.1253/circj.CJ-99-0225. PMID: 19597299.


36. Risbud MV, Fertala J, Vresilovic EJ, Albert TJ, Shapiro IM. 2005; Nucleus pulposus cells upregulate PI3K/Akt and MEK/ERK signaling pathways under hypoxic conditions and resist apoptosis induced by serum withdrawal. Spine. 30:882–889. DOI: 10.1097/01.brs.0000159096.11248.6d. PMID: 15834331.


37. Olivares-Silva F, Landaeta R, Aránguiz P, Bolivar S, Humeres C, Anfossi R, Vivar R, Boza P, Muñoz C, Pardo-Jiménez V, Peiró C, Sánchez-Ferrer CF, Díaz-Araya G. 2018; Heparan sulfate potentiates leukocyte adhesion on cardiac fibroblast by enhancing Vcam-1 and Icam-1 expression. Biochim Biophys Acta Mol Basis Dis. 1864:831–842. DOI: 10.1016/j.bbadis.2017.12.002. PMID: 29222072.


38. Zibara K, Chignier E, Covacho C, Poston R, Canard G, Hardy P, McGregor J. 2000; Modulation of expression of endothelial intercellular adhesion molecule-1, platelet-endothelial cell adhesion molecule-1, and vascular cell adhesion molecule-1 in aortic arch lesions of apolipoprotein E-deficient compared with wild-type mice. Arterioscler Thromb Vasc Biol. 20:2288–2296. DOI: 10.1161/01.ATV.20.10.2288. PMID: 11031217.


39. Griendling KK, FitzGerald GA. 2003; Oxidative stress and cardiovascular injury: part II: animal and human studies. Circulation. 108:2034–2040. DOI: 10.1161/01.CIR.0000093661.90582.c4. PMID: 14581381.

40. Sies H. 2015; Oxidative stress: a concept in redox biology and medicine. Redox Biol. 4:180–183. DOI: 10.1016/j.redox.2015.01.002. PMID: 25588755. PMCID: PMC4309861.



41. Qiao J, Arthur JF, Gardiner EE, Andrews RK, Zeng L, Xu K. 2018; Regulation of platelet activation and thrombus formation by reactive oxygen species. Redox Biol. 14:126–130. DOI: 10.1016/j.redox.2017.08.021. PMID: 28888895. PMCID: PMC5596263.


42. Furie B, Furie BC. 2008; Mechanisms of thrombus formation. N Engl J Med. 359:938–949. DOI: 10.1056/NEJMra0801082. PMID: 18753650.


43. Lu J, Xiang G, Liu M, Mei W, Xiang L, Dong J. 2015; Irisin protects against endothelial injury and ameliorates atherosclerosis in apolipoprotein E-Null diabetic mice. Atherosclerosis. 243:438–448. DOI: 10.1016/j.atherosclerosis.2015.10.020. PMID: 26520898.


44. Yau JW, Teoh H, Verma S. 2015; Endothelial cell control of thrombosis. BMC Cardiovasc Disord. 15:130. DOI: 10.1186/s12872-015-0124-z. PMID: 26481314. PMCID: PMC4617895.



45. Ren M, Li R, Luo M, Chen N, Deng X, Yan K, Zeng M, Wu J. 2014; Endothelial cells but not platelets are the major source of Toll-like receptor 4 in the arterial thrombosis and tissue factor expression in mice. Am J Physiol Regul Integr Comp Physiol. 307:R901–R907. DOI: 10.1152/ajpregu.00324.2014. PMID: 25275013.


46. Neeves KB. 2015; Physiochemical artifacts in FeCl3 thrombosis models. Blood. 126:700–701. DOI: 10.1182/blood-2015-05-644708. PMID: 26251225.

47. Angkeow P, Deshpande SS, Qi B, Liu YX, Park YC, Jeon BH, Ozaki M, Irani K. 2002; Redox factor-1: an extra-nuclear role in the regulation of endothelial oxidative stress and apoptosis. Cell Death Differ. 9:717–725. DOI: 10.1038/sj.cdd.4401025. PMID: 12058277.


48. Ozaki M, Suzuki S, Irani K. 2002; Redox factor-1/APE suppresses oxidative stress by inhibiting the rac1 GTPase. FASEB J. 16:889–890. DOI: 10.1096/fj.01-0664fje. PMID: 12039869.

49. Guo Y, Chen J, Zhao T, Fan Z. 2008; Granzyme K degrades the redox/DNA repair enzyme Ape1 to trigger oxidative stress of target cells leading to cytotoxicity. Mol Immunol. 45:2225–2235. DOI: 10.1016/j.molimm.2007.11.020. PMID: 18179823.


50. Kim CS, Son SJ, Kim EK, Kim SN, Yoo DG, Kim HS, Ryoo SW, Lee SD, Irani K, Jeon BH. 2006; Apurinic/apyrimidinic endonuclease1/redox factor-1 inhibits monocyte adhesion in endothelial cells. Cardiovasc Res. 69:520–526. DOI: 10.1016/j.cardiores.2005.10.014. PMID: 16325162.


51. Hall JL, Wang X, Van Adamson , Zhao Y, Gibbons GH. 2001; Overexpression of Ref-1 inhibits hypoxia and tumor necrosis factor-induced endothelial cell apoptosis through nuclear factor-kappab-independent and -dependent pathways. Circ Res. 88:1247–1253. DOI: 10.1161/hh1201.091796. PMID: 11420300.

52. Yuk JM, Yang CS, Shin DM, Kim KK, Lee SK, Song YJ, Lee HM, Cho CH, Jeon BH, Jo EK. 2009; A dual regulatory role of apurinic/apyrimidinic endonuclease 1/redox factor-1 in HMGB1-induced inflammatory responses. Antioxid Redox Signal. 11:575–588. DOI: 10.1089/ars.2008.2196. PMID: 18715145.


53. Zhang WJ, Wei H, Hagen T, Frei B. 2007; Alpha-lipoic acid attenuates LPS-induced inflammatory responses by activating the phosphoinositide 3-kinase/Akt signaling pathway. Proc Natl Acad Sci U S A. 104:4077–4082. DOI: 10.1073/pnas.0700305104. PMID: 17360480. PMCID: PMC1805485.


54. Yu M, Ge C, Zeng W, Mi Y, Zhang C. 2012; Retinoic acid promotes proliferation of chicken primordial germ cells via activation of PI3K/Akt-mediated NF-κB signalling cascade. Cell Biol Int. 36:705–712. DOI: 10.1042/CBI20110542. PMID: 22548360.


55. Sorensen BB, Rao LV, Tornehave D, Gammeltoft S, Petersen LC. 2003; Antiapoptotic effect of coagulation factor VIIa. Blood. 102:1708–1715. DOI: 10.1182/blood-2003-01-0157. PMID: 12738672.


56. Mast AE. 2016; Tissue factor pathway inhibitor: multiple anticoagulant activities for a single protein. Arterioscler Thromb Vasc Biol. 36:9–14. DOI: 10.1161/ATVBAHA.115.305996. PMID: 26603155. PMCID: PMC4690769.

57. Maeda NY, Clavé MM, Bydlowski SP, Lopes AA. 2016; Decreased circulating thrombomodulin is improved by tadalafil therapy in hypoxemic patients with advanced pulmonary arterial hypertension. Thromb Res. 146:15–19. DOI: 10.1016/j.thromres.2016.08.016. PMID: 27564658.


58. Cybulsky MI, Gimbrone MA Jr. 1991; Endothelial expression of a mononuclear leukocyte adhesion molecule during atherogenesis. Science. 251:788–791. DOI: 10.1126/science.1990440. PMID: 1990440.


59. Ambrosio G, Tritto I, Chiariello M. 1995; The role of oxygen free radicals in preconditioning. J Mol Cell Cardiol. 27:1035–1039. DOI: 10.1016/0022-2828(95)90072-1. PMID: 7563100.


60. Golino P, Ragni M, Cirillo P, Avvedimento VE, Feliciello A, Esposito N, Scognamiglio A, Trimarco B, Iaccarino G, Condorelli M, Chiariello M, Ambrosio G. 1996; Effects of tissue factor induced by oxygen free radicals on coronary flow during reperfusion. Nat Med. 2:35–40. DOI: 10.1038/nm0196-35. PMID: 8564835.


61. Tritto I, D'Andrea D, Eramo N, Scognamiglio A, De Simone C, Violante A, Esposito A, Chiariello M, Ambrosio G. 1997; Oxygen radicals can induce preconditioning in rabbit hearts. Circ Res. 80:743–748. DOI: 10.1161/01.RES.80.5.743. PMID: 9130455.


62. Tritto I, Duilio C, Santoro G, Elia PP, Cirillo P, De Simone C, Chiariello M, Ambrosio G. 1998; A short burst of oxygen radicals at reflow induces sustained release of oxidized glutathione from postischemic hearts. Free Radic Biol Med. 24:290–297. DOI: 10.1016/S0891-5849(97)00229-3. PMID: 9433904.


63. Khechai F, Ollivier V, Bridey F, Amar M, Hakim J, de Prost D. 1997; Effect of advanced glycation end product-modified albumin on tissue factor expression by monocytes. Role of oxidant stress and protein tyrosine kinase activation. Arterioscler Thromb Vasc Biol. 17:2885–2890. DOI: 10.1161/01.ATV.17.11.2885. PMID: 9409271.

Fig. 1
Redox factor-1 (Ref-1) suppresses FeCl3-induced arterial thrombosis formation.
(A) Timeline of experimental thrombus induction. (B) The arteries were infected with adβ-gal or adRef-1 for 24 h. Thrombosis was induced for 5 min, followed by 4 h incubation. The arteries were then harvested and Western blotting was performed to confirm Ref-1 overexpression. β-actin was used as an internal control. (C) The expression level of Ref-1 was quantified by densitometric analysis using ImageJ software. (D) FeCl3-mediated injury was induced in the adenovirus-infected carotid arteries for 5 min, and arterial blood flow was monitored constantly using a flow module until it had ceased entirely for 5 min. (E) Statistical graph of the TTO data. (F) After adenovirus infection and FeCl3-mediated injury, the arterial segments were isolated and stained with H&E. Scale bar 200 μm. (G) Statistical data are presented as ratios of the thrombus area. All data are presented as means ± standard deviations of three independent experiments. *p < 0.05 vs. Adβ-gal + FeCl3 (n = 3/group).
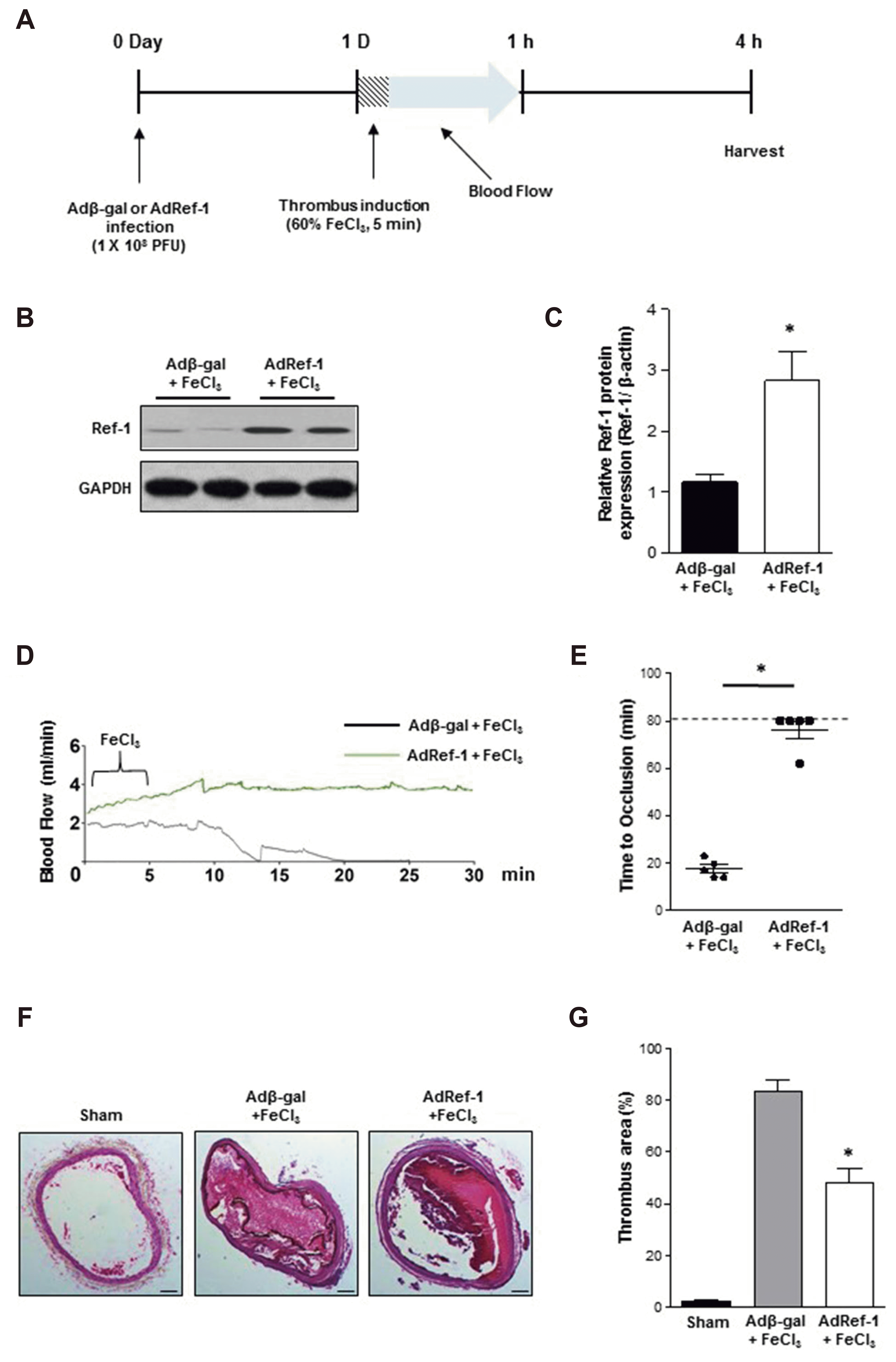
Fig. 2
Effect of redox factor-1 (Ref-1) overexpression on the Akt/glycogen synthase kinase 3 beta (GSK3β)/nuclear factor (NF)-κB signaling pathway and the expression of TF pathway inhibitor (TFPI), tissue factor (TF) and thrombomodulin. The arteries were infected with adβ-gal or adRef-1 for 24 h. Thrombosis was induced for 5 min, followed by 4 h incubation.
The arteries were harvested and Western blotting was performed to measure the phosphorylation of (A) Akt and GSK3β, (C) p65, and the protein expressions of (C) TF, (E) TFPI and thrombomodulin. The total forms of the proteins and GAPDH were used as internal controls. The expression levels of the proteins were quantified by densitometric analysis using ImageJ software (B, D), and (F). All data are presented as means ± standard deviations of three independent experiments. *p < 0.05 vs. Adβ-gal + FeCl3 (n = 3/group).

Fig. 3
Redox factor-1 (Ref-1) suppresses the expression of inflammatory molecules in the carotid artery.
The arteries were infected with adβ-gal or adRef-1 for 24 h. Thrombosis was induced for 5 min, followed by 4 h incubation. The arteries were harvested and Western blotting was performed to measure the protein expressions of (A) vascular cell adhesion molecule-1 (VCAM-1) and intercellular adhesion molecule-1 (ICAM-1). GAPDH was used as an internal control. (B) The expression levels of VCAM-1 and ICAM-1 were quantified by densitometric analysis using ImageJ software. All data are presented as means ± standard deviations of three independent experiments. *p < 0.05 vs. Adβ-gal + FeCl3 (n = 3/group).
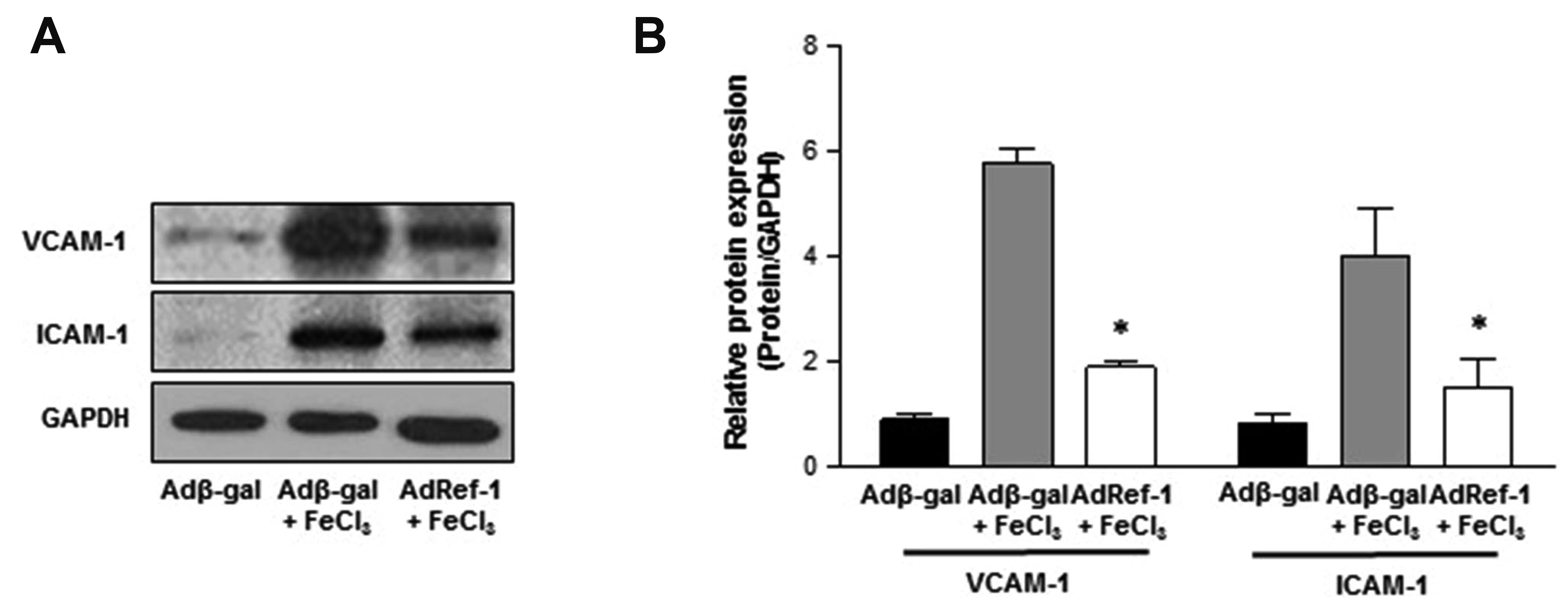
Fig. 4
Redox factor-1 (Ref-1) suppresses reactive oxygen species (ROS) production in the carotid artery.
Arterial segments were isolated and stained with dihydroethidium (DHE) after adenovirus infection and FeCl3-mediated injury. (A) The fluorescent signal of 2-hydroxyethidium (2-EOH, the oxidative form of DHE) was measured by high-performance liquid chromatography to detect ROS levels. Arrows indicate the 2-EOH signals. (B) Statistical graph of the 2-EOH signal. All data are presented as means ± standard deviations of three independent experiments. *p < 0.05 vs. Adβ-gal + FeCl3 (n = 3/group).
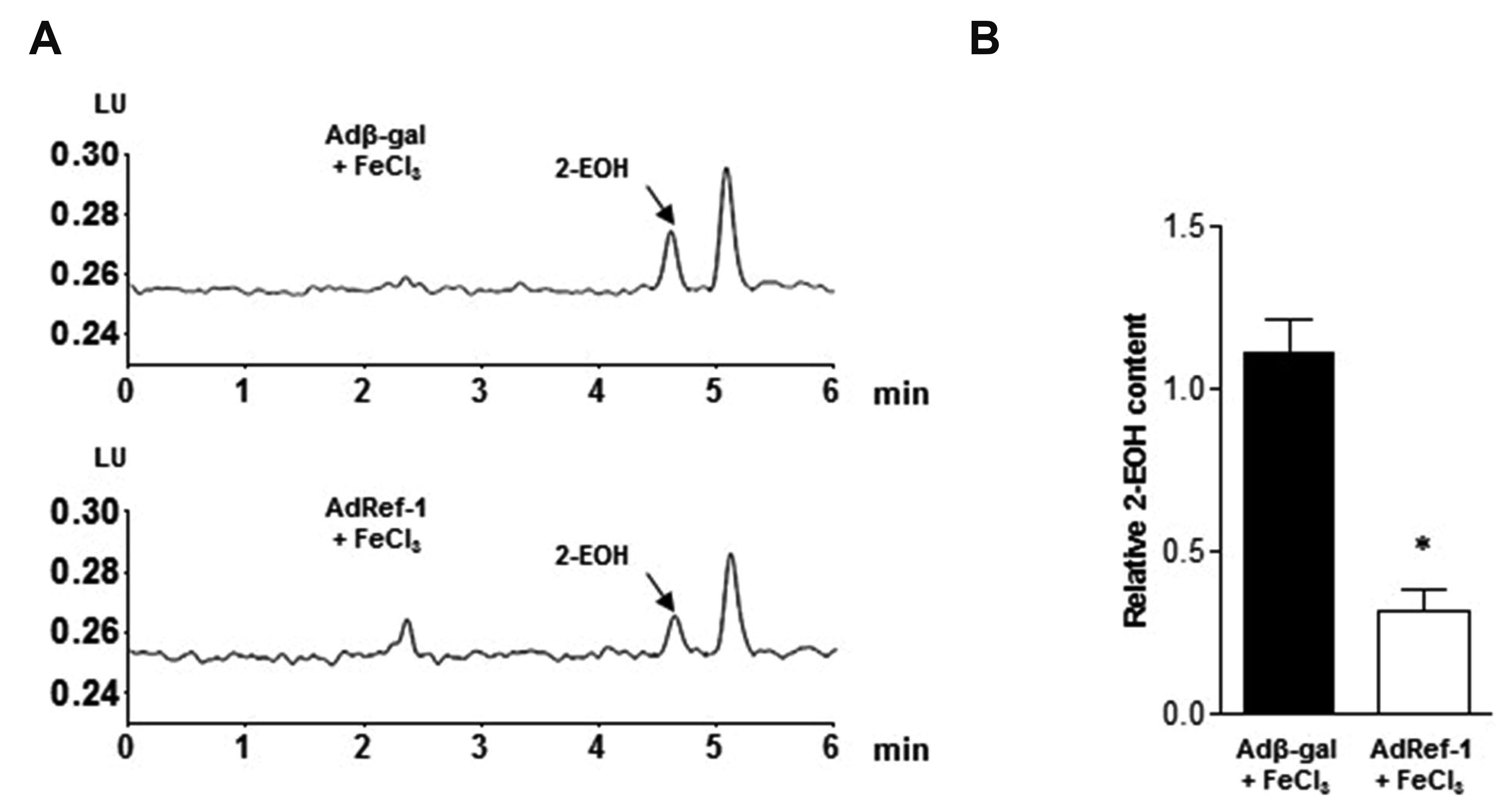
Fig. 5
Schematic model of the pathways involved in FeCl3-induced thrombus formation and the possible role of apyrimidinic endonuclease 1/redox factor-1 (APE1/Ref-1) in the inhibition of thrombus formation.
GSK3β, glycogen synthase kinase 3 beta; TF, tissue factor; TFPI, TF pathway inhibitor; ROS, reactive oxygen species.
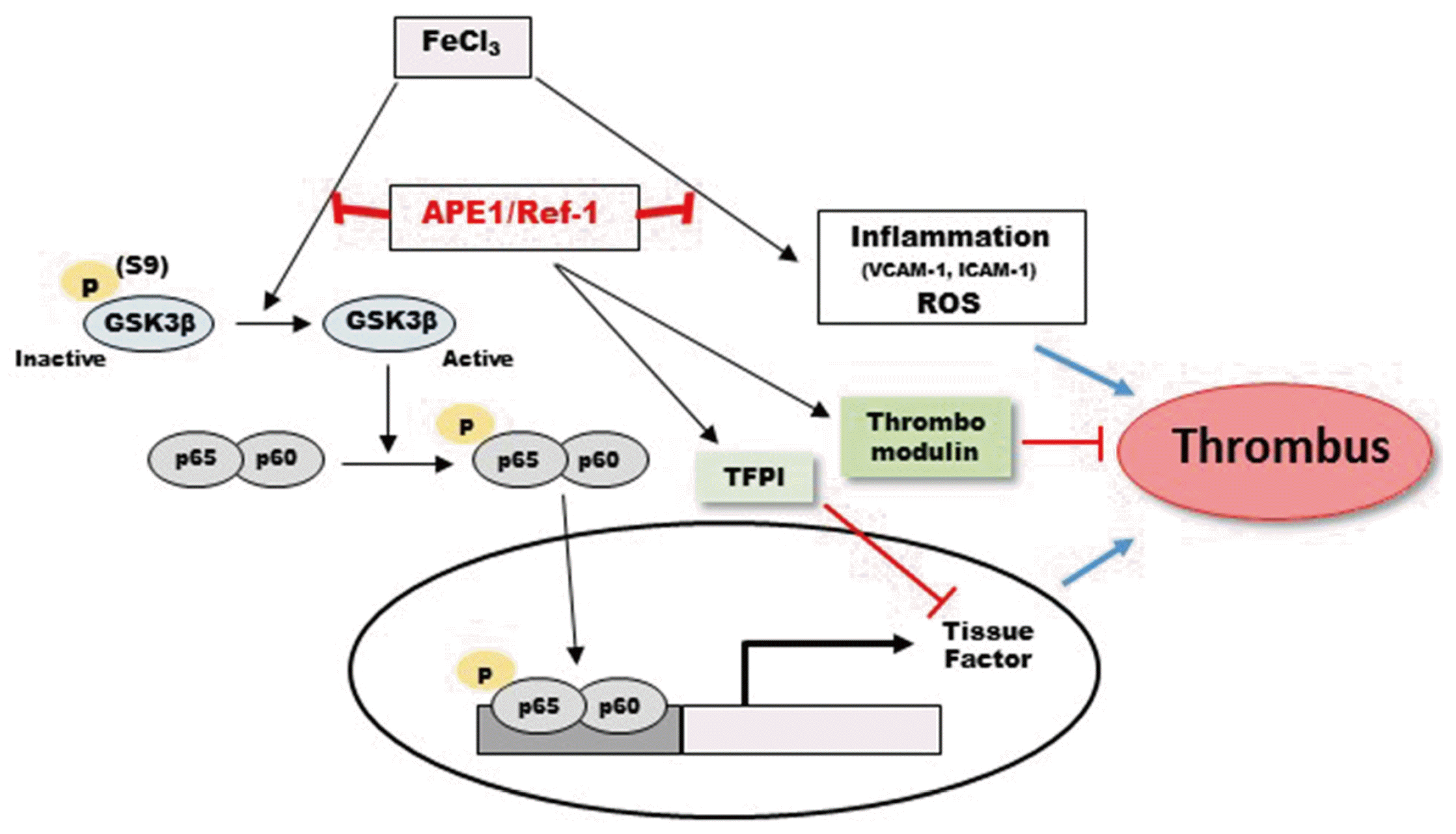