Abstract
Delphinidin is a major anthocyanidin compound found in various vegetables and fruits. It has anti-oxidant, anti-inflammatory, and various other biological activities. In this study we demonstrated the anti-cancer activity of delphinidin, which was related to autophagy, in radiation-exposed non-small cell lung cancer (NSCLC). Radiosensitising effects were assessed in vitro by treating cells with a sub-cytotoxic dose of delphinidin (5 µM) before exposure to γ-ionising radiation (IR). We found that treatment with delphinidin or IR induced NSCLC cell death in vitro; however the combination of delphinidin pre-treatment and IR was more effective than either agent alone, yielding a radiation enhancement ratio of 1.54 at the 50% lethal dose. Moreover, combined treatment with delphinidin and IR, enhanced apoptotic cell death, suppressed the mTOR pathway, and activated the JNK/MAPK pathway. Delphinidin inhibited the phosphorylation of PI3K, AKT, and mTOR, and increased the expression of autophagy-induced cell death associated-protein in radiation-exposed NSCLC cells. In addition, JNK phosphorylation was upregulated by delphinidin pre-treatment in radiation-exposed NSCLC cells. Collectively, these results show that delphinidin acts as a radiation-sensitizing agent through autophagy induction and JNK/MAPK pathway activation, thus enhancing apoptotic cell death in NSCLC cells.
Anthocyanins show antioxidant activity and it have been shown to prevent or lower the risk of diseases such as diabetes, cardiovascular disease, arthritis, and cancer [1-3]. Notably, interest has been focused on the potential of various phytochemicals including (polyphenols, isothiocyanates and anthocyanins etc.) as radiation sensitisers [4-6]. Delphinidin, as a representative of anthocyanins, has been reported as an anti-cancer reagent in several cell lines [7-9]. The fact that delphinidin can achieve these effects with lower adverse effects supports the possibility of delphinidin as a candidate reagent for alternative chemotherapy and radiotherapy. It may provide a therapeutic advantage in the clinical management of various standard modalities, even in refractory tumours over others.
Autophagy is an evolutionarily conserved intracellular constitutive degradation process, which morphologically involves the formation of double-membrane autophagosomes that degrade and recycle organelles and broken proteins following fusion with lysosomes [10]. Autophagy can play a pivotal role in cancer self-defence by allowing cells to resist the stress conditions caused by inappropriately regulated metabolic and genetic toxicity [11]. On the contrary, excessive autophagy finally leads to programmed cell death (PCD) I, or causes type II PCD, which is a distinct cellular suicide pathway [12]. In cases where autophagy induction serves an adaptive response or a pro-survival function in response to lethal stress, repressing autophagy may increase the efficacy of treatment by increasing the chemosensitivity or radiation sensitivity of tumour cells [13]. In contrast, upregulating autophagic processes within transformed cells resistant to apoptotic cell death may be a reasonable therapeutic strategy for these cancer types [12]. Nevertheless, when stressful conditions are sustained or excessive, autophagy becomes a suicide pathway that works through the digestion of essential cellular proteins and structures [14]. Recently reported evidence also shows that the p53/DRAM-autophagy axis plays a part in anticancer strategies that cause cytotoxicity in cancer cells [15,16]. Interestingly, Kulkarni et al. [17] established that MonoD (a novel synthetic molecule) increased autophagosome formation and upregulated the expression of proteins involved in autophagy flux and found that this is related to the death of non-small cell lung cancer (NSCLC) cells. However, there have been studies showing that the autophagy process is activated in different cells during various stages of tumour development, and results in complex effects on tumour growth [18]. Previous studies have reported that activated autophagy inhibits hepatocellular carcinoma development in the dysplastic phase. In contrast, it promotes hepatocyte production in the tumour-forming period [19], suggesting that autophagy plays diverse roles at different periods of tumour development, and is affected by cell specificity and the microenvironment. Delphinidin is also known as an autophagy stimulant [20,21]. Induction of autophagy by delphinidin depends on upregulated expression of LC3-II and ATG5, which may contribute to inhibition of the AKT/mTOR pathway, resulting in the activation of AMP-activated kinase (AMPK), which promotes the expression of several autophagy-related proteins [22]. Therefore, this study aimed to investigate the possibility of delphinidin as an agent to activate autophagy in order to increase radiosensitivity in NSCLC radiation therapy.
The chemicals and reagents were not purified by further procedures before being used. Delphinidin chloride, 3-(4,5-dimethylthiazol-2-yl)-2,5-diphenyltetrazolium bromide (MTT), and anti-LC3 (#L7543) were purchased from Sigma-Aldrich (St. Louis, MO, USA). Anti-caspase 3 (#9662), anti-cleaved caspase 3 (#9664), anti-PI3K (#4249), anti-p-PI3K (#4228), anti-AKT (#4691), anti- p-AKT (#4060), anti-mTOR (#2983), anti-p-mTOR (#5536), anti-ERK (#4695), anti-p-ERK (#4377), anti-p38 (#9212), anti-p-p38 (#9215), anti-JNK (#9252), anti-p-JNK (#9251), anti-p53 (#9282), and anti-GAPDH (#2118) were purchased from Cell Signaling Technology, Inc. (Danvers, MA, USA). The antibody for DRAM (ab188648) was purchased from Abcam (Cambridge, UK). RPMI 1640, penicillin/streptomycin (P/S), and foetal bovine serum (FBS) were purchased from Lonza (Walkersville, MD, USA). The Annexin V kit was purchased from Millipore (San Francisco, CA, USA).
The A549 cell line (human, lung, carcinoma) was purchased from the Korean Cell Line Bank (Seoul, Korea). Cells were cultured under sterile conditions at 37°C in a humid environment containing CO2 (5%); the culture medium comprised RPMI 1640 medium supplemented with foetal bovine serum (10%), glutamine (4 mM), penicillin (100 U/ml), and streptomycin (100 μg/ml).
The A549 cells were seeded into 96-well or 12-well plates at a density of 1 × 104 cells or 1 × 106 cells, and were treated with different concentrations of delphinidin (0–50 µM) for 24 h. For the MTT assay; MTT solution was added to each well, and the plates were incubated for 4 h at 37°C. DMSO was then added to each well, and the plates were incubated for 30 min at room temperature. Cell viability was determined using a spectrophotometer; absorbance was measured at –540 nm (Ultrospec 7000; Biochrom, Holliston, MA, USA). For the Annexin V assay, cells were washed twice in PBS, and were harvested with trypsin-EDTA. Suspended cells were then transferred new tubes, and were incubated for 20 min at room temperature with 100 µl Annexin V reagent. Apoptosis was determined using a Muse Cell Analyzer (Millipore, Billerica, MA, USA).
γ-ray irradiation was performed at room temperature with 6 MV VARIAN linear accelerators (RapidArc; Varian Medical Systems, Inc., Paolo Alto, CA, USA) at 4 Gy/min. Irradiation was performed directly on cells in flasks.
Typically, cell survival curves were considered to represent the relationship between the fraction of cells that maintain reproductive integrity and the absorbed radiation dose. Therefore, to further investigate if delphinidin selectively enhanced the radiosensitivity of A549 cells, clonogenic survival curves were acquired. A549 cells were seeded into 6-well plates at a density of 1,000 cells/well, and treated with 5 μM delphinidin for 24 h, followed by exposure to various doses (1, 4, 8, 12, 16, and 20 Gy) of γ-ray irradiation. Following irradiation, cells were incubated with fresh media for 7 days, fixed using pure methanol for 20 min at room temperature and stained with Wright’s stain (Thermo Fisher Scientific, Inc., San Jose, CA, USA) for 20 min. The number of colonies containing more than 50 cells was counted. The radiation enhancement ratio was defined as the ratio of dose parameters for the radiation-sensitizing agent and control exposures.
The A549 cells (1 × 105 cells/well) were seeded on sterilised glass coverslips (BD Biosciences, Bedford, MA, USA) in triplicate. Following 24 h of treatment with 5 µM delphinidin, the cells were exposed 4 Gy of γ-ray. The cells were then fixed in 4% paraformaldehyde for 10 min, permeabilised with 0.25% Triton X-100 for 10 min, and then incubated with the LC3 primary antibody for 2 h at room temperature. The cells were washed and incubated with the appropriate fluorescence-labelled secondary antibodies for 1 h at room temperature. The nuclei were stained with DAPI and incubated for 5 min. After mounting, fluorescence images were acquired using a confocal microscope (LSM 700; Carl Zeiss, Thornwood, NY, USA). To quantify the immune-reacted cells, the fluorescence intensity was measured in 10 randomly selected images.
The A549 cells were washed with PBS and lysed with radio immunoprecipitation assay buffer. The proteins (30–50 μg) were separated by 10% sodium dodecyl sulphate-polyacrylamide gel electrophoresis, and transferred to polyvinylidene difluoride membranes. The membranes were then blocked with 5% non-fat dry milk for 1 h at room temperature and then incubated overnight with 1:1,000-diluted primary antibodies at 4°C. The membranes were washed with tris-buffered saline and incubated with horseradish peroxidase-conjugated secondary antibodies (anti-rabbit IgG, HRP-linked antibody, #7074; Cell Signaling Technology, Inc.) for 2 h at room temperature. The proteins were then visualised using enhanced chemiluminescent reagent (ECL; Millipore Corp.) and exposure to γ-ray film.
Total RNA was extracted from A549 cells using TRIzol (Invitrogen; Thermo Fisher Scientific, Inc.). First-strand cDNA synthesis from the total RNA template was performed using the PrimeScript RT master mix (Takara Bio, Inc., Otsu, Japan). The resulting cDNA was subjected to quantitative PCR using TOPreal qPCR 2X PreMIX SYBR (Enzynomics, Daejeon, Korea) with a CFX-96 Touch Real-Time PCR Detection system (Bio-Rad Laboratories, Inc., Hercules, CA, USA). Expression data were calculated from the cycle threshold (Cq) value using the ΔΔCq method of quantification. Gene expression values were normalised to GAPDH expression. The forward and reverse primer sequences for the target genes included: 5´-GGACAGTTGCACACACTAGG-3´ and 5´-ATCCGGGTAGCTCAGATG-3´ for ATG5; 5´-GGGAAGGACTTACGGATG-3´ and 5´-CTTCTTGGTCTGGGGAAG-3 for ATG12; 5´-CAGTGGAACAGTCTCTCGAC-3´ and 5´-CCTTAGGGTGACACTCTGGA-3 for DRAM; and 5´-CTGAACGGGAAGCTCACT-3´ and 5´-AAGTCAGAGGAGACCACCTG-3´ for GAPDH, respectively.
Each experiment was performed at least three times, and the results were expressed as the mean ± standard deviation. Statistical procedures were performed using SPSS software (SPSS Inc., Chicago, IL, USA). For multiple comparisons, One-way analysis of variance (ANOVA) was used followed by Tukey's multiple comparisons test. The significance of differences was defined as *p < 0.05, **p < 0.01, and ***p < 0.001.
The inhibitory effect of delphinidin on A549 cells was explored by evaluating cell viability using the MTT assay. As shown in Fig. 1A, delphinidin treatment inhibited the viability of A549 cells in a concentration-dependent manner. The IC50 value of delphinidin against A549 cells was approximately 30.1 μM. To explore the relevance of apoptosis, the cytotoxic effect of delphinidin in A549 cells was examined using the Annexin V assay. The total apoptotic portion (including both early and late apoptosis) in the experimental group was clearly increased compared to that in the non-treated control in a dose-dependent manner (Fig. 1B, C). Collectively, the data suggested that delphinidin has inhibitory effects on A549 cells in a dose-dependent manner.
A sub-cytotoxic dose of delphinidin (5 μM) was chosen to study the combined effect of delphinidin and radiation on cell radiosensitivity. To further demonstrate the radiosensitising effect of delphinidin on various NSCLCs, A549, NCI-H460, and HCC827 cells were treated with 5 μM of delphinidin, and then irradiated with 4 Gy. As a result, the cell viability of the ionising radiation (IR) + delphinidin group was significantly reduced compared to that of the IR alone group (A549, p < 0.01 vs. IR; NCI-H460, p <0.05 vs. IR; HCC827, p < 0.01 vs. IR) (Fig. 2A). As shown in Fig. 2B, the 50 % lethal dose (LD50) of IR alone and IR + delphinidin treated A549 cells was measured to be 7.21 Gy and 4.68 Gy, respectively. Moreover, delphinidin enhanced the radiosensitivity of A549 cells with a radiation enhancement ratio of 1.54 at LD50. Similar results were also derived from the Annexin V data as shown in Fig. 2C, D. Delphinidin alone did not induce cell apoptosis, but radiation exposure (4 Gy) increased the apoptosis rate by 42.18% ± 4.17%. Interestingly, the total apoptosis rate was the most increased in the combined treatment group by 58.37% ± 5.20%. We further explored whether caspase-dependent signalling was involved in delphinidin-induced apoptotic cell death. To elucidate the expression of cleaved caspase-3 (activation form), an immunoprecipitation assay was performed. As shown in Fig. 2E and F, in the IR + delphinidin treatment, the ratio of cleaved caspase 3 (cleaved caspase-3/total caspase-3) was significantly increased compared to that in the IR only group (p < 0.01 vs. IR). Collectively, the data suggested that delphinidin promoted apoptosis in radiation-exposed NSCLC cells.
Autophagy is expected to vary in progress depending on the tumour type and treatment conditions and is known to play a variety of functions in tumour cell development and death. To explain the radiosensitising effects of delphinidin and to explore the involvement of autophagy, we examined the induction of autophagy by delphinidin in radiation-exposed A549 cells. The protein LC3 is well-known as an autophagosomal marker, and the formation of autophagosomes is commonly considered the standard of autophagy induction [23]. In particular, lipidation of the LC3 protein is expressed as modification from LC3 I to LC3 II, which indicates autophagy induction. Therefore, immunoblot analysis was performed to detect whether delphinidin + IR induced the transformation of full-length LC3 I into LC3 II in A549 cells. Fig. 3A, B and d show that delphinidin increased the LC3-II/LC-I ratio in a concentration-dependent manner in A549 cells. Interestingly, the change in the LC3-II/LC3-I ratio was not significant in the group irradiated with 4 Gy of radiation alone (Fig. 3C, D). However, as shown in Fig. 3E and G, the lipidation of LC3 II was increased significantly in the delphinidin + IR group at 24 h time point (p < 0.001 vs. time 0). We also detected transformed LC3 using an immunofluorescence assay. Fig. 3I and J showed that the number of condensed bright red fluorescent dots were increased in the combination treatment group. These results indicate that delphinidin induces autophagosome formation in proportion with concentration, as well as enhances autophagy induction under IR-exposure conditions. The same phenomenon and trend were observed in the qPCR analysis. Autophagosome formation is controlled by various ATGs such as the ATG12-ATG5 and LC3 complexes [24]. In particular, the involvement of ATG12 and ATG5 is essential for early membrane elongation [25]. Thus, to examine whether the autophagy-related complexes are activated by delphinidin administration in radiation-exposed cells, qPCR analysis was performed to measure the mRNA expression level of ATG5 and ATG12. As shown in Fig. 3F and H, the mRNA levels of ATG 5 and ATG 12 were significantly elevated following the exposure of A549 cells to radiation. In particular, the expression levels of ATG5 (p < 0.05 vs. IR) and ATG12 (p < 0.01 vs. IR) were the highest in the irradiated group treated with 5 μM of delphinidin, and significant differences were observed when compared with the radiation alone group in the combination treatment group. To explore the mechanism of delphinidin-induced autophagy in radiation exposed A549 cells, we also examined the expression levels of the PI3K/AKT/mTOR signalling pathway which is a negative sensor in autophagic cell damage. As shown in Fig. 3K–N, delphinidin inhibited the phosphorylation of PI3K, AKT, and mTOR, which are upstream proteins in the mTOR signalling pathway, in radiation-exposed A549 cells. Taken together, these results suggest that delphinidin induces autophagy when A549 cells are exposed to radiation.
Previously, many studies have reported a contrastive function for delphinidin. It could either promote anticancer effects or induce a protective effect to mediate cancer cell survival depending on the cell phenotype [22,26]. In this study, to explore the possible mechanisms underlying the anticancer effect of delphinidin in radiation-exposed NSCLC cells, we examined the expression level of autophagic cell death associated proteins. The damage-regulator autophagy modulator (DRAM), a lysosomal protein, has been known to regulate autophagy and cell death in a p53-dependent manner [27,28]. Therefore, we examined the expression levels of DRAM and p53 by immunoblot analysis. Fig. 4A–C show that the expression of p53 and DRAM was upregulated by delphinidin in radiation-exposed A549 cells. In the qPCR analysis, mRNA level of DRAM was also found to be significantly elevated in the combined treatment group compared to that in cells treated with IR alone (p < 0.01 vs. IR) (Fig. 4D). These data suggest that regulation of p53 and DRAM could increase autophagy in radiation-exposed A549 cells through delphinidin treatment. Moreover, to further investigate whether the radiosensitising effect of delphinidin was associated with mitogen‐activated protein kinase (MAPK)-signalling pathways which are a key signal transmission network in eukaryotes [29], we also examined the protein expression of the MAPK family (ERK1/2, p38, and JNK) by western blot analysis. Fig. 4E–H show that the expression levels of p‐ERK1/2 in radiation-exposed A549 cells were downregulated whereas p‐JNK was upregulated. In particular, these changes in expression levels were most pronounced in the combination treatment group. On the contrary, the expression level of p-p38 was almost similar between with or without the delphinidin treatment. Overall, the results support the hypothesis that delphinidin enhances radiotherapeutic effects in NSCLC cells via modulation of ERK and JNK/MAPK, but not p38.
Autophagy is a pivotal breakdown process that balances the energy source at critical times during the development of the biological system or is activated in response to nutrient stress [30]. Thus, regulation of autophagy may be an adaptive molecular mechanism that protects cells from internal/external stimulus-induced cell damage or death. However, the role of autophagy in cancer therapy is controversial and requires detailed analysis to be considered as a treatment strategy. Some studies have shown that autophagy is a survival factor for cancer cells under chemotherapy because it is considered a defence mechanism in environments such as hypoxia, nutrient depletion, and exposure to ionising radiation [31]. However, in other cases, autophagy can be a pathway of auto-digestion and self-destruction, leading to the death of cancer cells [32,33]. Previous studies have demonstrated that NSCLC cells show low expression of autophagy-related genes and proteins, which may be associated with tumour formation and lung cancer development [34]. As the apoptotic pathway is inactivated in NSCLC cells, they become resistant during radiation therapy; therefore, enhancement of autophagy regulation by delphinidin + IR may thus represent an alternative method for removing NSCLC cells. Delphinidin has been shown to induce autophagy in numerous cancer cells, but it is not yet known whether improvement in autophagy involves cell death or cell repair in NSCLC cells. This study demonstrated that autophagy-related proteins showed notably low expression in non-treated A549 cells as compared to that in delphinidin + IR treatment, suggesting that apoptosis by delphinidin + IR may be related to autophagy (Fig. 3). Jiang reported that human lung cancer tissues express beclin-1 (a key autophagic regulator) and LC3-II at a downregulated level [35,36]. Beclin-1 protein has been found to be downregulated in NSCLC, and weak beclin-1 expression was significantly associated with worsening disease prognosis, which supports the role of autophagy as a potential tumour suppressor [37]. Furthermore, in radiation therapy for NSCLC, radiation exposure induces a significant obstacle to lysosome function, leading to an imbalance between autophagolysosome formation and degradation [38]. Therefore, enhancement of autophagy flux may be used as a new therapeutic strategy for the anti-cancer treatment of radio-resistant NSCLC. Transient homeostasis collapse can be recovered by transient stimulation of autophagy and/or compensatory upregulation of the defence system; this allows cells to overcome imbalances and achieve equilibrium through successful recycling of unnecessary cellular components [39]. However, continued stress and persistent upregulated autophagy leads to cell death through unrecoverable starvation [40]. In this experiment, delphinidin + IR co-treatment enhanced LC3 lipidation compared to IR only in A549 cells. Thus, induction of autophagy by delphinidin + IR can enhance the efficacy of radiation therapy on NSCLC cells by utilising autophagy mechanisms as mentioned earlier.
The MAPK pathway plays a key role in cancer progression [41]. Classical MAPK pathways include several routes such as extracellular signal regulatory kinases (ERK), c-jun N-terminal kinases, or stress-activated protein kinases (JNK or SAPK); and MAPK14 [42]. So far, six distinct groups of MAPK are reported to be involved in mammals—ERK1/2, ERK3/4, ERK5, ERK7/8, JNK1/2/3, and the p38 isoforms a/b/c (ERK6)/d. The MAPK pathway can be activated by extracellular stimuli such as G-Coupled Receptors (GPCR), genotoxic agents, oxidative stress, and irradiation [43]. The role of MAPK in cancer treatment is as diverse as the type of cancer itself, and stress-activated MAPKs such as p38 MAPK and JNK play a wide variety of roles in tumour characteristics [44,45]. Recently, several studies have provided new information on the JNK-MAPK pathway function, showing a pivotal role in regulating the cascades of autophagy and apoptosis in response to cellular stress [46,47]. Shajahan et al. [48] reported that JNK is a positive regulator for genotoxic stress-induced apoptosis because it mediates anti-apoptotic proteins Bcl-2/Bcl-xL phosphorylation followed by changing the mitochondrial membrane potential, resulting in the release of cytochrome C; activation of caspase 9 and then caspase 3, finally induce apoptosis. Interestingly, JNK signalling is also associated with autophagy induction. JNK induces phosphorylation of Bcl-2, resulted in dissociation of the Bcl-2:beclin-1 complex. Autophagy is stimulated by the release of Beclin-1, an essential autophagy modulator [49]. These studies indicate that JNK-MAPK can mediate apoptosis and autophagy in stress responses to extracellular stimuli. Furthermore, DRAM is a p53 target gene that induces transcription of lysosomal proteins, which induce macroautophagy as crucial factors for p53-mediated death, and p53 is mainly regarded as a cancer suppressor factor [28]. In this study, the sensitisation effects induced by delphinidin combined with IR occurred with increased phosphorylation of JNK, but decreased ERK pathway under the same condition; this suggests that the sensitisation effects were mediated by the JNK-MAPK pathway. Notably, we found that the expression of p53 and DRAM proteins was increased by the IR + delphinidin treatment, and DRAM expression as well as autophagic cell death was increased more with IR + delphinidin, indicating that IR + delphinidin upregulates autophagic cell damage signalling.
In conclusion, this study suggests that delphinidin can effectively improve anti-proliferative effects by increasing radiation sensitivity in A549 cells through upregulation of autophagy upon radiation therapy.
ACKNOWLEDGEMENTS
We thank the technical support provided from the Korea Drug Development Platform, in which radio-isotopes were used in the Korea Institute of Radiological & Medical Sciences. This project was supported by the Nuclear R&D Program of the Ministry of Science and ICT, as a branch of the Republic of Korea.
Notes
REFERENCES
1. Tsuyuki S, Fukui S, Watanabe A, Akune S, Tanabe M, Yoshida K. 2012; Delphinidin induces autolysosome as well as autophagosome formation and delphinidin-induced autophagy exerts a cell protective role. J Biochem Mol Toxicol. 26:445–453. DOI: 10.1002/jbt.21443. PMID: 23129091.


2. Wang H, Nair MG, Strasburg GM, Chang YC, Booren AM, Gray JI, DeWitt DL. 1999; Antioxidant and antiinflammatory activities of anthocyanins and their aglycon, cyanidin, from tart cherries. J Nat Prod. 62:294–296. DOI: 10.1021/np980501m. PMID: 10075763.


3. Kim B, Lee SG, Park YK, Ku CS, Pham TX, Wegner CJ, Yang Y, Koo SI, Chun OK, Lee JY. 2016; Blueberry, blackberry, and blackcurrant differentially affect plasma lipids and pro-inflammatory markers in diet-induced obesity mice. Nutr Res Pract. 10:494–500. DOI: 10.4162/nrp.2016.10.5.494. PMID: 27698956. PMCID: PMC5037066.


4. Kasten-Pisula U, Windhorst S, Dahm-Daphi J, Mayr G, Dikomey E. 2007; Radiosensitization of tumour cell lines by the polyphenol Gossypol results from depressed double-strand break repair and not from enhanced apoptosis. Radiother Oncol. 83:296–303. DOI: 10.1016/j.radonc.2007.04.024. PMID: 17521756.


5. Bump EA, Hoffman SJ, Foye WO. Abraham DJ, editor. 2003. Radiosensitizers and radioprotective agents. Burger's medicinal chemistry and drug discovery. 6th ed. Wiley;Hoboken (NJ): p. 151–214. DOI: 10.1002/0471266949.bmc077.


6. Nambiar D, Rajamani P, Singh RP. 2011; Effects of phytochemicals on ionization radiation-mediated carcinogenesis and cancer therapy. Mutat Res. 728:139–157. DOI: 10.1016/j.mrrev.2011.07.005. PMID: 22030216.


7. Kim MH, Jeong YJ, Cho HJ, Hoe HS, Park KK, Park YY, Choi YH, Kim CH, Chang HW, Park YJ, Chung IK, Chang YC. 2017; Delphinidin inhibits angiogenesis through the suppression of HIF-1α and VEGF expression in A549 lung cancer cells. Oncol Rep. 37:777–784. DOI: 10.3892/or.2016.5296. PMID: 27959445.


8. Jeong MH, Ko H, Jeon H, Sung GJ, Park SY, Jun WJ, Lee YH, Lee J, Lee SW, Yoon HG, Choi KC. 2016; Delphinidin induces apoptosis via cleaved HDAC3-mediated p53 acetylation and oligomerization in prostate cancer cells. Oncotarget. 7:56767–56780. DOI: 10.18632/oncotarget.10790. PMID: 27462923. PMCID: PMC5302952.


9. Chen J, Zhou J, Li F, Zhu Y, Zhang W, Yu X. 2017; [Delphinidin induces autophagy in HER-2+ breast cancer cells via inhibition of AKT/mTOR pathway]. Zhong Nan Da Xue Xue Bao Yi Xue Ban. 42:264–270. Chinese. DOI: 10.11817/j.issn.1672-7347.2017.03.005. PMID: 28364098.
10. Levine B, Kroemer G. 2008; Autophagy in the pathogenesis of disease. Cell. 132:27–42. DOI: 10.1016/j.cell.2007.12.018. PMID: 18191218. PMCID: PMC2696814.


11. Jin S, White E. 2007; Role of autophagy in cancer: management of metabolic stress. Autophagy. 3:28–31. DOI: 10.4161/auto.3269. PMID: 16969128. PMCID: PMC2770734.


12. Kondo Y, Kondo S. 2006; Autophagy and cancer therapy. Autophagy. 2:85–90. DOI: 10.4161/auto.2.2.2463. PMID: 16874083.


13. Bialik S, Dasari SK, Kimchi A. 2018; Autophagy-dependent cell death - where, how and why a cell eats itself to death. J Cell Sci. 131:jcs215152. DOI: 10.1242/jcs.215152. PMID: 30237248.


14. Gozuacik D, Kimchi A. 2004; Autophagy as a cell death and tumor suppressor mechanism. Oncogene. 23:2891–2906. DOI: 10.1038/sj.onc.1207521. PMID: 15077152.


15. Kim TH, Kim HS, Kang YJ, Yoon S, Lee J, Choi WS, Jung JH, Kim HS. 2015; Psammaplin A induces Sirtuin 1-dependent autophagic cell death in doxorubicin-resistant MCF-7/adr human breast cancer cells and xenografts. Biochim Biophys Acta. 1850:401–410. DOI: 10.1016/j.bbagen.2014.11.007. PMID: 25445714.


16. Mohammadpour R, Safarian S, Ejeian F, Sheikholya-Lavasani Z, Abdolmohammadi MH, Sheinabi N. 2014; Acetazolamide triggers death inducing autophagy in T-47D breast cancer cells. Cell Biol Int. 38:228–238. DOI: 10.1002/cbin.10197. PMID: 24155029.
17. Kulkarni YM, Kaushik V, Azad N, Wright C, Rojanasakul Y, O'Doherty G, Iyer AK. 2016; Autophagy-induced apoptosis in lung cancer cells by a novel digitoxin analog. J Cell Physiol. 231:817–828. DOI: 10.1002/jcp.25129. PMID: 26264876. PMCID: PMC4751068.


18. Degenhardt K, Mathew R, Beaudoin B, Bray K, Anderson D, Chen G, Mukherjee C, Shi Y, Gélinas C, Fan Y, Nelson DA, Jin S, White E. 2006; Autophagy promotes tumor cell survival and restricts necrosis, inflammation, and tumorigenesis. Cancer Cell. 10:51–64. DOI: 10.1016/j.ccr.2006.06.001. PMID: 16843265. PMCID: PMC2857533.


19. Liu L, Liao JZ, He XX, Li PY. 2017; The role of autophagy in hepatocellular carcinoma: friend or foe. Oncotarget. 8:57707–57722. DOI: 10.18632/oncotarget.17202. PMID: 28915706. PMCID: PMC5593678.


20. Feng R, Wang SY, Shi YH, Fan J, Yin XM. 2010; Delphinidin induces necrosis in hepatocellular carcinoma cells in the presence of 3-methyladenine, an autophagy inhibitor. J Agric Food Chem. 58:3957–3964. DOI: 10.1021/jf9025458. PMID: 20025272.


21. Lee DY, Park YJ, Hwang SC, Kim KD, Moon DK, Kim DH. 2018; Cytotoxic effects of delphinidin in human osteosarcoma cells. Acta Orthop Traumatol Turc. 52:58–64. DOI: 10.1016/j.aott.2017.11.011. PMID: 29290536. PMCID: PMC6136320.


22. Chen J, Zhu Y, Zhang W, Peng X, Zhou J, Li F, Han B, Liu X, Ou Y, Yu X. 2018; Delphinidin induced protective autophagy via mTOR pathway suppression and AMPK pathway activation in HER-2 positive breast cancer cells. BMC Cancer. 18:342. DOI: 10.1186/s12885-018-4231-y. PMID: 29587684. PMCID: PMC5870693.


23. Tanida I, Minematsu-Ikeguchi N, Ueno T, Kominami E. 2005; Lysosomal turnover, but not a cellular level, of endogenous LC3 is a marker for autophagy. Autophagy. 1:84–91. DOI: 10.4161/auto.1.2.1697. PMID: 16874052.


24. Otomo C, Metlagel Z, Takaesu G, Otomo T. 2013; Structure of the human ATG12~ATG5 conjugate required for LC3 lipidation in autophagy. Nat Struct Mol Biol. 20:59–66. DOI: 10.1038/nsmb.2431. PMID: 23202584. PMCID: PMC3540207.


25. Mizushima N. Deretic V, editor. 2006. Transgenic models of autophagy. Autophagy in immunity and infection: a novel immune effector. Wiley-VCH;Weinheim: p. 55–67. DOI: 10.1002/352760880X.ch3.


26. Hafeez BB, Siddiqui IA, Asim M, Malik A, Afaq F, Adhami VM, Saleem M, Din M, Mukhtar H. 2008; A dietary anthocyanidin delphinidin induces apoptosis of human prostate cancer PC3 cells in vitro and in vivo: involvement of nuclear factor-kappaB signaling. Cancer Res. 68:8564–8572. DOI: 10.1158/0008-5472.CAN-08-2232. PMID: 18922932. PMCID: PMC3149885.
27. Crighton D, Wilkinson S, Ryan KM. 2007; DRAM links autophagy to p53 and programmed cell death. Autophagy. 3:72–74. DOI: 10.4161/auto.3438. PMID: 17102582.


28. Crighton D, Wilkinson S, O'Prey J, Syed N, Smith P, Harrison PR, Gasco M, Garrone O, Crook T, Ryan KM. 2006; DRAM, a p53-induced modulator of autophagy, is critical for apoptosis. Cell. 126:121–134. DOI: 10.1016/j.cell.2006.05.034. PMID: 16839881.


29. Wilkinson MG, Millar JB. 2000; Control of the eukaryotic cell cycle by MAP kinase signaling pathways. FASEB J. 14:2147–2157. DOI: 10.1096/fj.00-0102rev. PMID: 11053235.


30. Mizushima N. 2007; Autophagy: process and function. Genes Dev. 21:2861–2873. DOI: 10.1101/gad.1599207. PMID: 18006683.


31. Gewirtz DA. 2014; The four faces of autophagy: implications for cancer therapy. Cancer Res. 74:647–651. DOI: 10.1158/0008-5472.CAN-13-2966. PMID: 24459182.


32. Sui X, Chen R, Wang Z, Huang Z, Kong N, Zhang M, Han W, Lou F, Yang J, Zhang Q, Wang X, He C, Pan H. 2013; Autophagy and chemotherapy resistance: a promising therapeutic target for cancer treatment. Cell Death Dis. 4:e838. DOI: 10.1038/cddis.2013.350. PMID: 24113172. PMCID: PMC3824660.


33. Notte A, Leclere L, Michiels C. 2011; Autophagy as a mediator of chemotherapy-induced cell death in cancer. Biochem Pharmacol. 82:427–434. DOI: 10.1016/j.bcp.2011.06.015. PMID: 21704023.


34. Liu Q, Wang JJ, Pan YC, Meng LF, Zhan X, Zheng QF. 2008; [Expression of autophagy-related genes Beclin1 and MAPLC3 in non-small cell lung cancer]. Ai Zheng. 27:25–29. Chinese. PMID: 18184459.
35. Jiang ZF, Shao LJ, Wang WM, Yan XB, Liu RY. 2012; Decreased expression of Beclin-1 and LC3 in human lung cancer. Mol Biol Rep. 39:259–267. DOI: 10.1007/s11033-011-0734-1. PMID: 21556768.


36. Fu LL, Cheng Y, Liu B. 2013; Beclin-1: autophagic regulator and therapeutic target in cancer. Int J Biochem Cell Biol. 45:921–924. DOI: 10.1016/j.biocel.2013.02.007. PMID: 23420005.


37. Valente G, Morani F, Nicotra G, Fusco N, Peracchio C, Titone R, Alabiso O, Arisio R, Katsaros D, Benedetto C, Isidoro C. 2014; Expression and clinical significance of the autophagy proteins BECLIN 1 and LC3 in ovarian cancer. Biomed Res Int. 2014:462658. DOI: 10.1155/2014/462658. PMID: 25136588. PMCID: PMC4127242.


38. Karagounis IV, Kalamida D, Mitrakas A, Pouliliou S, Liousia MV, Giatromanolaki A, Koukourakis MI. 2016; Repression of the autophagic response sensitises lung cancer cells to radiation and chemotherapy. Br J Cancer. 115:312–321. DOI: 10.1038/bjc.2016.202. PMID: 27380135. PMCID: PMC4973160.


39. Chu CT. 2006; Autophagic stress in neuronal injury and disease. J Neuropathol Exp Neurol. 65:423–432. DOI: 10.1097/01.jnen.0000229233.75253.be. PMID: 16772866. PMCID: PMC1885377.


40. Ouyang L, Shi Z, Zhao S, Wang FT, Zhou TT, Liu B, Bao JK. 2012; Programmed cell death pathways in cancer: a review of apoptosis, autophagy and programmed necrosis. Cell Prolif. 45:487–498. DOI: 10.1111/j.1365-2184.2012.00845.x. PMID: 23030059. PMCID: PMC6496669.


41. Huang P, Han J, Hui L. 2010; MAPK signaling in inflammation-associated cancer development. Protein Cell. 1:218–226. DOI: 10.1007/s13238-010-0019-9. PMID: 21203968. PMCID: PMC4875080.


42. Zhang W, Liu HT. 2002; MAPK signal pathways in the regulation of cell proliferation in mammalian cells. Cell Res. 12:9–18. DOI: 10.1038/sj.cr.7290105. PMID: 11942415.


43. Kyriakis JM, Avruch J. 2012; Mammalian MAPK signal transduction pathways activated by stress and inflammation: a 10-year update. Physiol Rev. 92:689–737. DOI: 10.1152/physrev.00028.2011. PMID: 22535895.


44. Reddy KB, Nabha SM, Atanaskova N. 2003; Role of MAP kinase in tumor progression and invasion. Cancer Metastasis Rev. 22:395–403. DOI: 10.1023/A:1023781114568. PMID: 12884914.
45. Burotto M, Chiou VL, Lee JM, Kohn EC. 2014; The MAPK pathway across different malignancies: a new perspective. Cancer. 120:3446–3456. DOI: 10.1002/cncr.28864. PMID: 24948110. PMCID: PMC4221543.


46. Sui X, Kong N, Ye L, Han W, Zhou J, Zhang Q, He C, Pan H. 2014; p38 and JNK MAPK pathways control the balance of apoptosis and autophagy in response to chemotherapeutic agents. Cancer Lett. 344:174–179. DOI: 10.1016/j.canlet.2013.11.019. PMID: 24333738.


47. Wong CH, Iskandar KB, Yadav SK, Hirpara JL, Loh T, Pervaiz S. 2010; Simultaneous induction of non-canonical autophagy and apoptosis in cancer cells by ROS-dependent ERK and JNK activation. PLoS One. 5:e9996. DOI: 10.1371/journal.pone.0009996. PMID: 20368806. PMCID: PMC2848860.


48. Shajahan AN, Dobbin ZC, Hickman FE, Dakshanamurthy S, Clarke R. 2012; Tyrosine-phosphorylated caveolin-1 (Tyr-14) increases sensitivity to paclitaxel by inhibiting BCL2 and BCLxL proteins via c-Jun N-terminal kinase (JNK). J Biol Chem. 287:17682–17692. DOI: 10.1074/jbc.M111.304022. PMID: 22433870. PMCID: PMC3366801.
49. Tian PG, Jiang ZX, Li JH, Zhou Z, Zhang QH. 2015; Spliced XBP1 promotes macrophage survival and autophagy by interacting with Beclin-1. Biochem Biophys Res Commun. 463:518–523. DOI: 10.1016/j.bbrc.2015.05.061. PMID: 26026678.
Fig. 1
Cytotoxicity of delphinidin on A549 cells after 24 h of incubation.
(A) MTT assay to evaluate A549 cell viability; (B) Statistical graph of Annexin V-positive A549 cells; (C) Annexin V-positive A549 cells. The results revealed no statistically significant cytotoxicity for media with delphinidin concentrations lower than 5 µM. Statistical differences were compared between the control group and the treated groups. MTT, 3-(4,5-dimethylthiazol-2-yl)-2,5-diphenyltetrazolium bromide. **p < 0.01, ***p < 0.001.
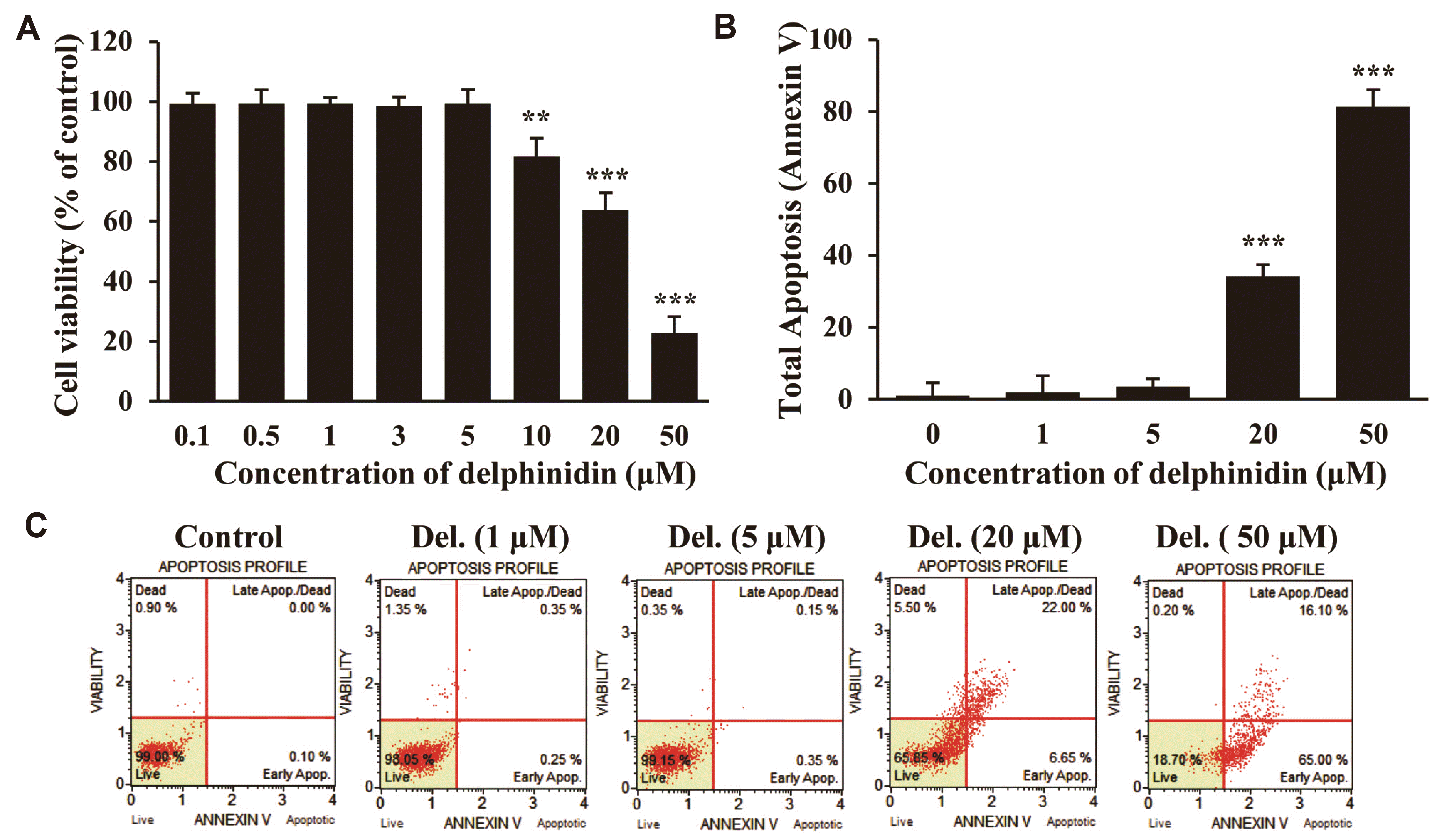
Fig. 2
Delphinidin increased apoptosis in gamma ray-exposed NSCLC cells.
(A) Cell viability detection by the CCK-8 assay in A549, NCI-H460, and HCC827 cells. (B) Clonogenic assay to evaluate A549 cell viability. (C) Representatieve images of Annexin V-positive reaction of A549 cells. (D) Statistical graph of the muse analysis. (E) Expression level of cleaved caspase-3 protein in A549 cells. (F) Statistical analysis of the cleaved caspase-3/caspase 3 ratio. IR, ionising radiation. *p < 0.05, **p < 0.01 vs. the IR group.
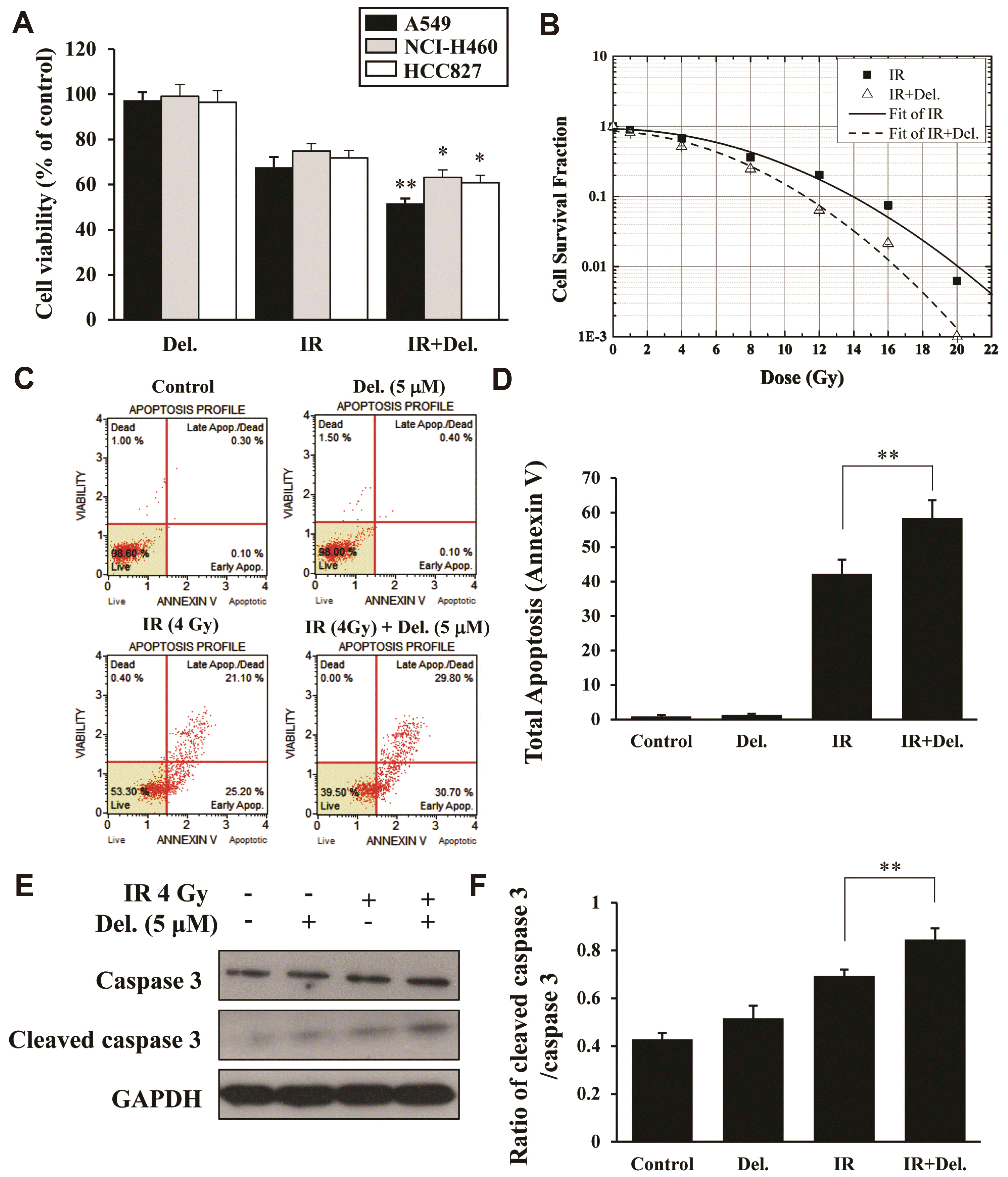
Fig. 3
Delphinidin induced autophagy and suppressed the PI3K/AKT/mTOR signalling pathway in gamma ray-exposed A549 cells.
(A) Western blotting for LC3 and GAPDH after treatment of cells with the indicated concentration of delphinidin for 24 h, (B) bar graph, quantitative evaluation of densitometric analysis. (D) Western blotting for LC3 and GAPDH after IR exposure to cells, (C) bar graph, quantitative evaluation of densitometric analysis. (G) Western blotting for LC3 and GAPDH after IR exposure with 5 μM delphinidin-pretreated A549 cells, (E) bar graph, quantitative evaluation of densitometric analysis. The relative expression of (F) ATG5 and (H) ATG12 mRNA was determined by qRT-PCR. (J) LC3 immunofluorescence-positive dots in A549 cells (×400). Quantification of the LC3 puncta average number from the image in (I) performed using the Image J quantification tool. (K) Suppression of upstream proteins from the mTOR signalling pathway, including PI3K, phospho-PI3K, AKT, phospho-AKT, mTOR and phospho-mTOR after IR. (L) The phospho-PI3K/PI3K, (M) the phospho-AKT/AKT, (N) the phospho-mTOR/mTOR ratio in the mTOR signalling pathway. Statistical analysis was performed for results from three independent experiments ± standard deviation. IR, ionising radiation. *p < 0.05, **p < 0.01, ***p < 0.001 compared with IR.

Fig. 4
Delphinidin activated the autophagic cell death pathway and JNK/MAPK signalling pathway in gamma-ray exposed A549 cells.
(A) The activity of up/downstream proteins from the autophagic cell death signalling pathway including p53 and DRAM after IR. (B) Statistical analysis of the p53/GAPDH ratio. (C) Statistical analysis of the DRAM/GAPDH ratio. (D) The relative expression of DRAM mRNA was determined by qRT-PCR. (E) The suppression and activation of MAPK signalling pathway, including ERK, phospho-ERK, p38, phospho-p38, JNK, and phospho-JNK after IR. (F) Statistical analysis of phospho-ERK/ERK. (G) Statistical analysis of phospho-p38/p38. (H) Statistical analysis of phospho-JNK/JNK. Statistical analysis was performed for results from three independent experiments ± standard deviation. DRAM, damage-regulator autophagy modulator; IR, ionising radiation; MAPK, mitogen‐activated protein kinase. **p < 0.01 compared with IR.
