Abstract
Transplant renal artery stenosis (TRAS) is one cause of allograft dysfunction. TRAS causes parenchymal necrosis and graft insufficiency. Herein, we report the case of a 40-year-old female with end-stage renal disease due to immunoglobulin A nephropathy, who underwent kidney transplantation with her elder sister. The surgery was successful and the allograft showed primary graft function. At postoperative day (POD) 2, urine output decreased sharply. We checked a non-enhanced abdominal computed tomography scan which showed subcapsular and pelvic cavity hematomas. She underwent hematoma removal surgery with renal upper polar capsulotomy. Bleeding control was successful, but her serum creatinine was 5.4 mg/dL. At POD 25, abdomen magnetic resonance angiography showed significant stenosis at the anastomosis site between the graft renal artery and the recipient’s internal iliac artery. Then, percutaneous transluminal angioplasty was implemented. Significant stenosis (>80%) was detected at the anastomotic site and a 5-mm stent was inserted at stenotic lesion with post-stent balloon angioplasty using a 5-mm balloon catheter. The renal arterial diameter and blood flow were normalized. At postoperative 5 months, a 99mTc dimercaptosuccinic acid scan showed multiple focal radioisotope defects. At 54 months after renal transplantation, her serum creatinine level was 4.0 mg/dL and her glomerular filtration rate was 13 mL/min/1.73 m2. Hence, we report that TRAS can cause parenchymal necrosis and allograft dysfunction.
Transplant renal artery stenosis (TRAS) is one of the causes of graft insufficiency. It is a common cause of mechanical problems at the initial stage of kidney transplantation with an incidence of 1%–23% [1]. TRAS is associated with surgical damage, acute rejection, viral infections, such as cytomegalovirus (CMV) infection, and atherosclerosis [2-4]. Although subclinical stenosis that does not cause clinical symptoms may occur, significant stenosis can cause clinical symptoms, such as decreased urine volume, increased serum creatinine level, and surgical site pain. Early detection and treatment of the stenotic region can restore graft function by maintaining the blood flow; however, delayed detection and treatment may lead to hypoperfusion and necrosis of the kidney parenchyma causing permanent deterioration of function. We report a case of persistent renal parenchymal necrosis and renal dysfunction on the second day after transplantation, despite stenting and balloon angioplasty for TRAS after subcapsular hematoma removal.
A 40-year-old woman was diagnosed with end-stage renal disease due to immunoglobulin A nephropathy. She underwent renal transplantation, with her elder sister as the donor, on October 14, 2014. Human leukocyte antigen typing showed no mismatch, panel reactive antibody was class II (8.3%), and complement-dependent cytotoxicity crossmatch was negative. The donor kidney had a single artery, a single vein, and weighed 210 g. The renal artery and recipient’s right internal iliac artery were anastomosed end-to-end, and the renal vein and recipient’s right external iliac vein were anastomosed end-to-side. The total ischemic time was 110 minutes (cold ischemic time, 68 minutes; warm ischemic time, 42 minutes) and immediate graft function was observed. On postoperative day 2, there was a sudden decrease in urine output and an increase in serum creatinine level. On the non-contrast abdominal computed tomography (CT) scan, subcapsular hematoma in the upper part of the kidney and bleeding around the bladder were observed (Fig. 1). The surgical team decided to remove the hematoma immediately.
Hemorrhage was not controlled during the laparoscopic hematoma removal. After removing the capsule at the upper pole of the graft, the lesion was closed with mesh material and the bleeding was stopped successfully. After hematoma removal, the patient did not experience any abdominal discomfort, and no change in blood pressure was observed. However, the serum creatinine level continued to increase. Ultrasonography (US) was performed 24 days posttransplantation. The peak systolic velocity (PSV) was observed to be 16.1 cm/s and the resistance index (RI) was normal (Fig. 2).
Hence, renal magnetic resonance angiography (MRA) was performed 20 days posttransplantation. MRA revealed an acute angle with significant stenosis at the anastomotic site of the renal artery and the recipient's internal iliac artery (Fig. 3). Focal hypoperfusion was also observed in the upper pole of the graft. Thus, the authors decided to perform percutaneous transluminal angioplasty (PTA). The left femoral artery was punctured and a 6-F sheath was inserted. During angiography, more than 80% stenosis was observed at the anastomotic site and blood flow to the cortex was decreased (Fig. 4).
After deployment of a 5-mm¡¿40-mm self-expandable stent (Epic; Boston Scientific Inc., Marlborough, MA, USA), balloon dilation was performed with a 5 mm¡¿40-mm balloon (Mustang; Boston Scientific Inc.) (Fig. 5). Thereafter, the TRAS disappeared and blood flow improved. Because a bare-metal stent was inserted, rivaroxaban 15 mg and aspirin 100 mg were administered once daily to prevent stent restenosis. Apixaban and aspirin have been administered to the patient since April 2019. US was performed 45 days posttransplantation and 21 days poststent insertion. The PSV was 19.8 cm/sec and the RI was normal (Fig. 6).
Three days after the procedure, the patient was discharged and is currently being observed in the outpatient clinic. Multiple localized radioisotope uptake deficits were noted on a 99mTc dimercaptosuccinic acid scan 5 months after transplantation (Fig. 7). Four years and 6 months posttransplantation, her serum creatinine and estimated glomerular filtration rate levels were 4.0 mg/dL and 13 mL/min/1.73 m2, respectively (Fig. 8).
TRAS is a common cause of posttransplant hypertension, graft failure, and graft loss [5]. It is usually observed within three months to 2 years after renal transplantation; however, it may occur immediately or 10 years after transplantation. It may be caused by donor or recipient vessel trauma during extraction, clamping, irrigation, suture, or intimal scar formation; by hyperplasia due to peripheral artery disease [1,6]; or by arterial atheroma caused by donor or recipient's atherosclerosis [4]. Immune-mediated damage caused by acute rejection may also cause TRAS [7]. It is more common in recipients of cadaveric kidneys than in those that received kidneys from living donors because of the long cold ischemic time, which can cause vascular fibrosis [8]. The mitogenic effect of CMV is also associated with TRAS.
TRAS may cause symptoms of hypertension, systemic edema, or heart failure posttransplantation because of water and sodium retention due to the activation of the renin-angiotensin system and the sympathetic nervous system [9]. The plasma renin activity test is not usually performed for TRAS diagnosis. This test is nonspecific, and a positive result can be observed during rejection reactions or due to other drug-induced causes [5]. The most easily performed test is color Doppler US. It is noninvasive and can be performed even when renal function is decreased. This is because a radiologic contrast agent is not administered. Moreover, it can be performed several times to assess the change in pattern [7]. US findings can be used to diagnose TRAS if the focal PSV is 180–200 cm/sec. In addition, if the PSV of other segments of the artery is ≤40–50 cm/sec, the possibility of TRAS diagnosis increases [7]. The specificity of the diagnosis increases when the PSV at the site of TRAS is 2.5 times higher than that of the poststenotic artery. Additionally, a reduced RI (<0.5) or the observation of a tardus-parvus waveform may be additional evidence of TRAS; however, the specificity is low because these characteristics can also be observed in rejection cases or in cases that arise due to other causes. Despite its advantages, color Doppler US is operator-dependent, and finding a transplant vessel using this method is difficult.
CT angiography is a noninvasive, accurate, and widely used diagnostic technique; however, caution is required in cases where renal function is compromised by contrast agents. Unlike CT angiography, MRA is a nonnephrotoxic gadolinium-based test that may be helpful in TRAS diagnosis; however, it is expensive and may lead to nephrogenic systemic sclerosis [10]. Arteriography with renal angiography, which is an invasive method, can be used. Although knowing the site, range, and exact location of the stenosis is advantageous, arterial puncture must be performed, and complications such as hemorrhage, vascular injury, and hematoma may occur. There is also the risk of contrast-induced acute kidney injury because contrast media must be injected into the blood vessels of the kidney.
Close observation is the treatment method for TRAS without clinical symptoms. During TRAS with symptoms such as hypertension and systemic edema, appropriate antihypertensive medications and loop diuretics may be helpful. However, in cases involving hemodynamically abnormal findings or allograft dysfunction, PTA with stent placement is the gold standard treatment [11]. Most of these treatments are known to be more effective in cases where the lesion is linear or distant from the anastomosis. According to Greenstein et al. [12], among 547 TRAS patients, 76% of patients in the PTA group were either cured or experienced improvements. Chew et al. [13] reported a clinical success rate of 76.9% in a 10-year retrospective study. However, Merkus et al. [14] reported that PTA was effective in only one third of TRAS cases. In a United States Renal Data System registry study, 145 of 823 TRAS patients underwent PTA, but improvements in allograft survival were not observed (P=0.4) [15]. In addition, PTA has been associated with renal artery dissection, stent restenosis, thromboembolism, hematoma, and pseudoaneurysm at the puncture site. If PTA is unsuccessful, open revision surgery may be an alternative treatment. This includes revision of the anastomosis, bypass grafting of the stenosis, localized endarterectomy, and excision/reimplantation of the renal artery [15]. The success rate is similar to that of PTA; however, the occurrence of additional surgical complications can lead to increased graft loss or high mortality rate.
The TRAS in our patient was cause by an acute angle that formed at the anastomotic site due to the transposition of the graft site after the hematoma removal surgery, and subsequent stenosis caused an ischemic lesion in the graft parenchyma and graft insufficiency. When early transplantation dysfunction occurs, the cause is often rejection. However, in the present case, the cause was TRAS-induced blood flow decrease after postoperative complications.
To prevent TRAS, bleeding or hematoma that may occur during surgery should be treated in advance. Additionally, care should be taken to prevent kinking, redundancy, warping, or bending in the anastomosis area of the graft's blood vessels and the recipient's blood vessels. In the future, TRAS may be identified by using US to verify whether the blood is flowing freely.
In the present case, TRAS was caused by mechanical complications due to reoperation for hematoma removal. Laparoscopic surgery was performed to avoid adhesions and reduce graft damage; however, this resulted in anastomosis stenosis. TRAS is curable and reversible; however, if not diagnosed early, it may cause renal parenchymal necrosis and persistent and unresolved allograft dysfunction. TRAS may cause renal dysfunction posttransplantation or early reoperation; early detection and treatment may restore graft function.
ACKNOWLEDGMENTS
Conflict of Interest
No potential conflict of interest relevant to this article was reported.
Author Contributions
Conceptualization: HSK, JHL. Data curation: DYL, HYK, DHK. Formal analysis: JSO, YHS, JKK. Investigation, Methodology, Project administration, Visualization, and Writing–original draft: HSK, JHL. Writing–original draft: HSK, JHL. Writing–review & editing: JHL, SDH.
REFERENCES
1. Fervenza FC, Lafayette RA, Alfrey EJ, Petersen J. 1998; Renal artery stenosis in kidney transplants. Am J Kidney Dis. 31:142–8. DOI: 10.1053/ajkd.1998.v31.pm9428466. PMID: 9428466.


2. Irshad A, Ackerman SJ, Campbell AS, Anis M. 2009; An overview of renal transplantation: current practice and use of ultrasound. Semin Ultrasound CT MR. 30:298–314. DOI: 10.1053/j.sult.2009.03.001. PMID: 19711642.


3. Becker BN, Odorico JS, Becker YT, Leverson G, McDermott JC, Grist T, et al. 1999; Peripheral vascular disease and renal transplant artery stenosis: a reappraisal of transplant renovascular disease. Clin Transplant. 13:349–55. DOI: 10.1034/j.1399-0012.1999.130412.x. PMID: 10485378.


4. Pouria S, State OI, Wong W, Hendry BM. 1998; CMV infection is associated with transplant renal artery stenosis. QJM. 91:185–9. DOI: 10.1093/qjmed/91.3.185. PMID: 9604070.


5. Bruno S, Remuzzi G, Ruggenenti P. 2004; Transplant renal artery stenosis. J Am Soc Nephrol. 15:134–41. DOI: 10.1097/01.ASN.0000099379.61001.F8. PMID: 14694165.
6. Salomon-Perzyński A, Walter-Croneck A, Usnarska-Zubkiewicz L, Dytfeld D, Zielińska P, Wojciechowska M, et al. 2019; Efficacy of daratumumab monotherapy in real-world heavily pretreated patients with relapsed or refractory multiple myeloma. Adv Med Sci. 64:349–55. DOI: 10.1016/j.advms.2019.05.001. PMID: 31125864.


7. Granata A, Clementi S, Londrino F, Romano G, Veroux M, Fiorini F, et al. 2014; Renal transplant vascular complications: the role of Doppler ultrasound. J Ultrasound. 18:101–7. DOI: 10.1007/s40477-014-0085-6. PMID: 26191097. PMCID: PMC4504861.


8. Halimi JM, Al-Najjar A, Buchler M, Birmelé B, Tranquart F, Alison D, et al. 1999; Transplant renal artery stenosis: potential role of ischemia/reperfusion injury and long-term outcome following angioplasty. J Urol. 161:28–32. DOI: 10.1016/S0022-5347(01)62051-2. PMID: 10037360.


9. Messerli FH, Bangalore S, Makani H, Rimoldi SF, Allemann Y, White CJ, et al. 2011; Flash pulmonary oedema and bilateral renal artery stenosis: the Pickering syndrome. Eur Heart J. 32:2231–5. DOI: 10.1093/eurheartj/ehr056. PMID: 21406441.


10. Jain R, Sawhney S. 2005; Contrast-enhanced MR angiography (CE-MRA) in the evaluation of vascular complications of renal transplantation. Clin Radiol. 60:1171–81. DOI: 10.1016/j.crad.2005.05.004. PMID: 16223613.


11. Patel NH, Jindal RM, Wilkin T, Rose S, Johnson MS, Shah H, et al. 2001; Renal arterial stenosis in renal allografts: retrospective study of predisposing factors and outcome after percutaneous transluminal angioplasty. Radiology. 219:663–7. DOI: 10.1148/radiology.219.3.r01jn30663. PMID: 11376251.


12. Greenstein SM, Verstandig A, McLean GK, Dafoe DC, Burke DR, Meranze SG, et al. 1987; Percutaneous transluminal angioplasty: the procedure of choice in the hypertensive renal allograft recipient with renal artery stenosis. Transplantation. 43:29–32. DOI: 10.1097/00007890-198701000-00007. PMID: 2948308.
13. Chew LL, Tan BS, Kumar K, Htoo MM, Wong KS, Cheng CW, et al. 2014; Percutaneous transluminal angioplasty of transplant renal artery stenosis. Ann Acad Med Singapore. 43:39–43. PMID: 24557464.
14. Merkus JW, Huysmans FT, Hoitsma AJ, Buskens FG, Skotnicki SH, Koene RA. 1993; Renal allograft artery stenosis: results of medical treatment and intervention. A retrospective analysis. Transpl Int. 6:111–5. DOI: 10.1111/j.1432-2277.1993.tb00761.x. PMID: 8447924.


15. Shames BD, Odorico JS, D'Alessandro AM, Pirsch JD, Sollinger HW. 2003; Surgical repair of transplant renal artery stenosis with preserved cadaveric iliac artery grafts. Ann Surg. 237:116–22. DOI: 10.1097/00000658-200301000-00016. PMID: 12496538. PMCID: PMC1513976.


FIGURES
Fig. 3
Magnetic resonance angiography: transplant renal artery stenosis (arrow) and focal parenchymal necrosis (arrowheads).
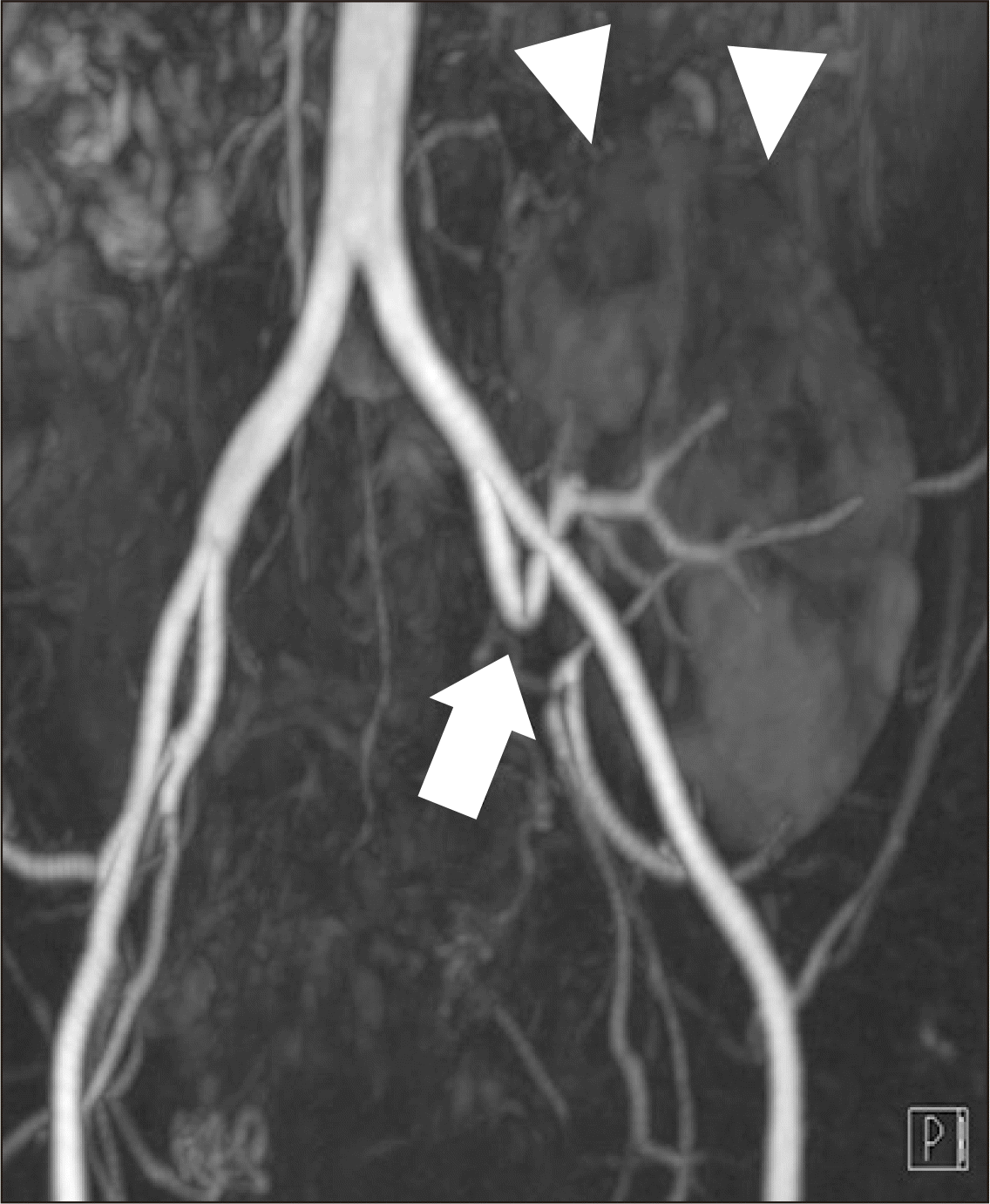
Fig. 5
Allograft angiography: postpercutaneous transluminal angioplasty and stent deployment (arrow).
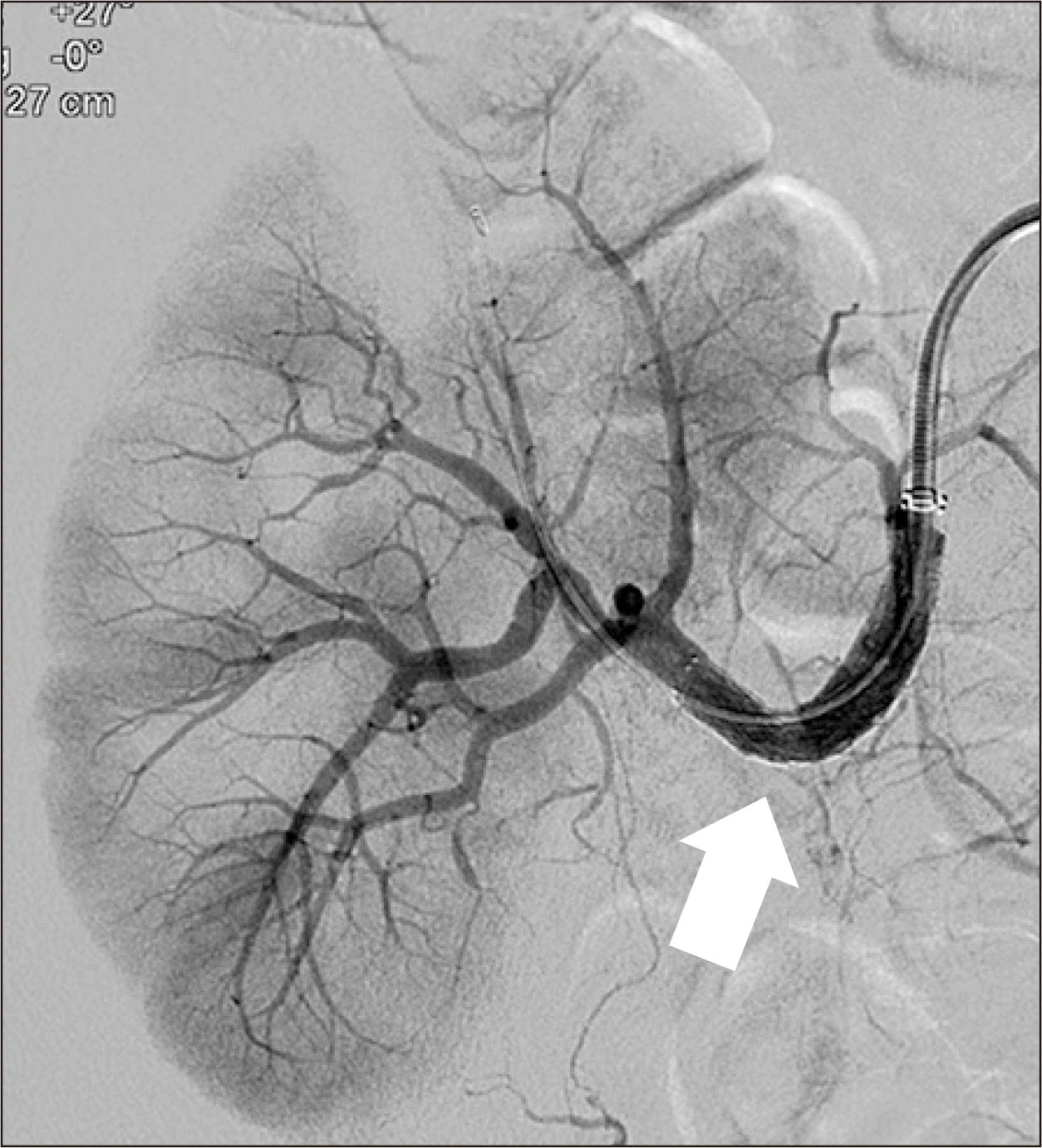
Fig. 6
Allograft ultrasonography (postoperative day 45) showing slightly increased peak systolic velocity.
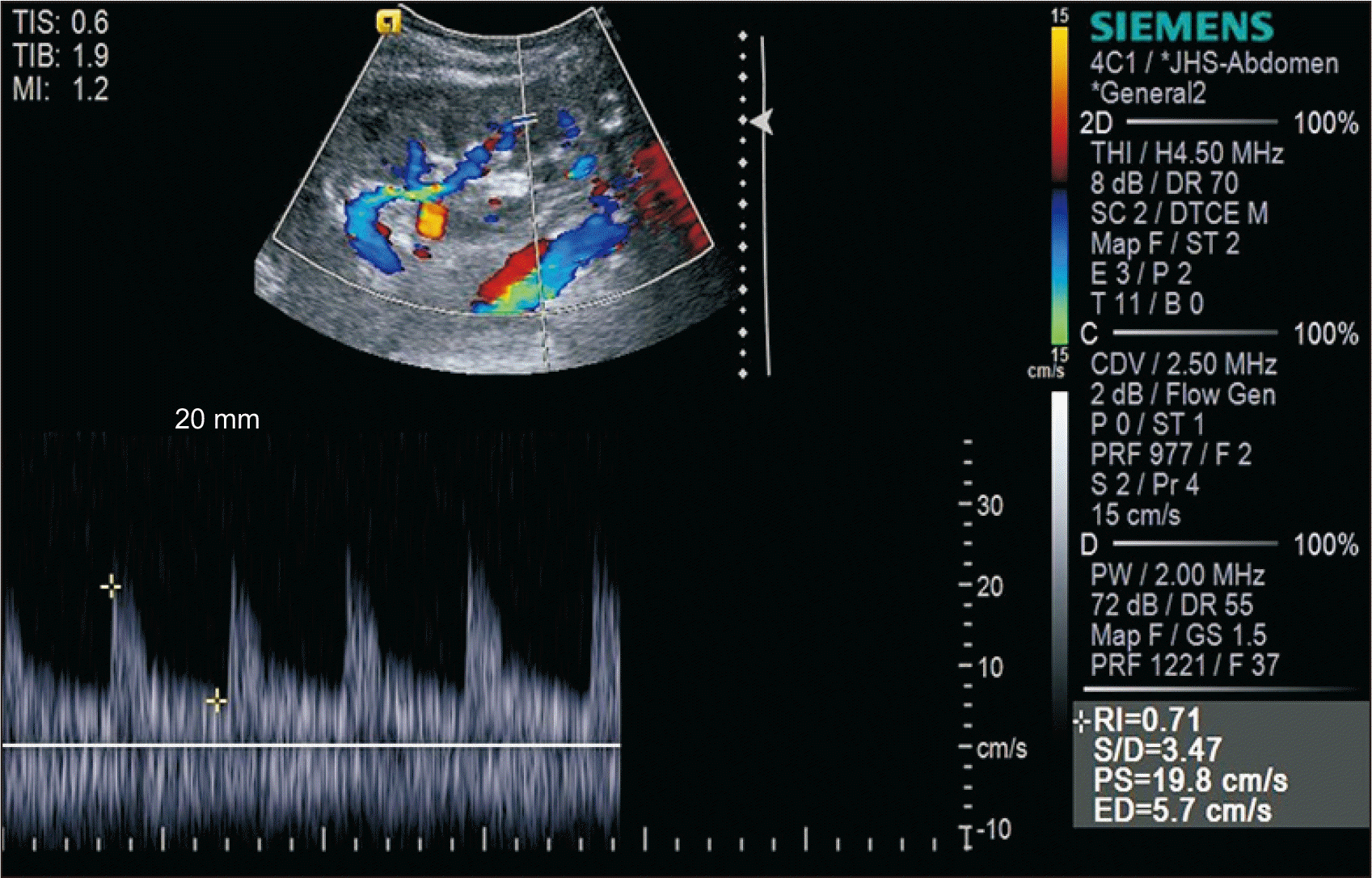