INTRODUCTION
The genus Acinetobacter is a genetically multifarious group of aerobic, Gram negative, and non-fermentative bacteria found in numerous healthcare environments (1). Acinetobacter nosocomialis belonging to the Acinetobacter calcoaceticus-baumannii community is an opportunistic nosocomial pathogen (2). Its invasive and non-invasive diseases have been extensively studied in various nosocomial contexts, although previous studies suggest that this bacterium might be less virulent than its closely related counterpart, A. baumannii. The pathogenic mechanism that allows A. baumannii to thrive in the environment is the formation of a biofilm through attachment to both biotic and abiotic surfaces, which initiates processes that protect against various factors and enable the bacteria to survive in healthcare environments (3). Communal behaviors of bacteria, such as virulence, biofilm formation and motility, are often modulated by regulating target gene expression via the quorum sensing (QS) system (4).
One of the best-known QS systems is the LuxI/LuxR two-component system (TCS) of Vibrio harveyi (5). Autoinducer, N-acyl-homoserine lactones (AHLs) of V. harveyi is synthesized by AHL synthase, LuxI using two substrates, S-adenosylmethionine (SAM) and an acylated acyl carrier protein (ACP). At a minimum threshold level, AHLs bind to the N-terminal region of LuxR and leads to form LuxR homodimers, which enables to regulate transcription of the target genes by binding to specific DNA sequences and thereby regulate transcription of the target genes. AnoI/AnoR is known as LuxI/LuxR homologous in A. nosocomialis (6), (7). Prior studies have reported AnoI/AnoR complex is responsible for up- or down-regulation of several target genes through AHLs mediated TCS.
Previous studies have reported that the QS system is involved in the biofilm-formation process and exogenous AHLs promote or inhibit biofilm formation in other bacterial strains (8). Biofilm formation is one of the major defense mechanisms that allow bacteria to withstand challenging environmental niches and survive (9). Biofilm development is a multistep process involving initial attachment, irreversible attachment, maturation, and dispersion (10), (11). Accumulation of bacterial cells internally in an extracellular matrix enables the bacteria to form a self-protecting growth pattern, prominently comprising exopolymers (EPs), which facilitate adherence to the solid surface (12), (13). Thus, understanding about the mechanism by which the QS system contributes to biofilm development could be beneficial in eradicating the infection at the initial stage, underscoring the importance of identifying effective ways to target biofilm formation by this pathogenic bacterium.
In our previous study, we demonstrated that AHLs are secreted by A. nosocomialis and exert a significant influence on biofilm and pellicle formation (7). In the current study, we constructed an anoI-deletion mutant of A. nosocomialis to further investigate the regulatory roles of the AHLs, C6-HSL, C10-HSL and C12-HSL, in biofilm formation and motility in A. nosocomialis. Here, we report the C12-HSL is the most prominent AHL, which plays a major role in regulating biofilm and motility in A. nosocomialis, whereas C6HSL and C10HSL may act as regulators in modulating other phenotypes through the QS mechanism.
MATERIALS AND METHODS
Bacterial strains and culture conditions
The bacterial strains and plasmid used in this study are listed in Table 1. Wild-type (WT) A. nosocomialis (ATCC 17903) was obtained from American Type Culture Collection (ATCC; VA, USA) and an anoI-deletion mutant (∆anoI) was constructed as a previously described method (7). All bacterial strains were grown in Luria-Bertani (LB) medium at 37°C. AHLs were exogenously added to the WT and anoI-deletion mutant as dictated by specified experimental requirements. Synthetic N-hexanoyl-homoserine lactone (C6-HSL), N-decanoyl-homoserine lactone (C10-HSL), and N-(3-hydroxy-dodecanoyl)- homoserine lactone (C12-HSL) were purchased from Sigma-Aldrich (St. Louis, MO, USA).
Table 1.
Bacterial strains and plasmid used in this study
Strains and plasmids | Relevant characteristicsa | Reference/source |
---|---|---|
A. nosocomialis ATCC17903 | Type strain | (14) |
∆anoI | A. nosocomialis derivative, ∆anoI::Kmr | This study |
DH5α λ pir | Plasmid replication | Laboratory collection |
S17-l λ pir | Conjugal donor | Laboratory collection |
pUC4K | pUC4 with nptI; Apr, Kmr | Laboratory collection |
pHKD01 | pDS132, multicloning sites; oriR6K, sacB, Cmr | (15) |
pOH01 | pHKD01 with △anoI::nptI; Cmr, Kmr | This study |
Construction of the deletion mutant ∆anoI
The anoI-deletion mutant was created from the A. nosocomialis WT strain by amplification of a DNA fragment following disruption of the open reading frame (ORF) of the target gene using an overlap extension-polymerase chain reaction (OE-PCR) method and a pair of specific primers, as listed in Table 2. Amplification of the mutated DNA fragment without the anoI gene was accomplished in two PCR steps using four PCR primers specific to upstream and downstream regions (~1 kb) of the coding region of anoI. To combine upstream and downstream regions of anoI with nptI (conferring kanamycin resistance) by overlap extension PCR, we designed ANOI02F and ANOI02R primers for amplification of the upstream region so as to contain an additional 25 nucleotides at their 5’ end homologous to the downstream region and nptI (7). The fragments were amplified using A. nosocomialis WT genomic DNA. The resulting fragments were then mixed in equimolar amounts and again subjected to OE-PCR with specific primers. The resulting 3.1-kb DNA fragment was ligated into a FspI-digested pHKD01 vector to generate pOH01. The E. coli S17-1 strain harboring the pOH01 was used as a conjugal donor for transferring the plasmid to A. nosocomialis ATCC 17903. Transconjugants were created and isolated using a previously described method (15). Briefly, the donor and recipient strains were grown in LB until the optical density at 600 nm (OD600) 0.8. The strains were mixed in equal ratio and spotted onto LB plate followed by incubation at 30 ℃ for 12 h. The bacteria were resuspended in LB broth and then plated on LB agar plates containing specific antibiotics to eliminate the donor strain and to select the merodiploid cells. The merodiploids were plated on LB agar containing 10% sucrose to excise the plasmid with the antibiotic resistance cassette from the chromosome. The deletion of the anoI gene was confirmed by PCR analysis.
Table 2.
Oligonucleotides used in this study
Growth curves
A. nosocomialis WT and the anoI-deletion mutant were cultivated in LB broth overnight and diluted into the main cultures at a ratio of 1:100. The diluted cultures were incubated at 37°C, and optical density at 600 nm (OD600) was measured at specific time points of incubation up to 24 h.
Biofilm formation assay
Biofilm formation was performed as previously described with slight modifications (7). Briefly, overnight subcultures of A. nosocomialis WT and anoI-deletion mutant were adjusted to an OD600 of 1, after which 50 μl was added to 5 ml of Mueller-Hinton (MH) medium in round-bottom polystyrene tubes (17´ 100 mm). The setup was incubated at 30°C without shaking for 48 h, then the pellicle formed in tubes was removed and the culture was carefully discarded without disturbing the biofilm attached to the surface of the tube. After air-drying tubes, 5 ml of 0.1% (w/v) crystal violet was added for 15 min to stain the biofilm, after which tubes were washed with sterile water and the crystal violet-stained biofilm was re-solubilized in 1 ml of 30% acetic acid. A 200-ml sample of the solution was then transferred to a 96-well microtiter plate and the absorbance in each sample at 595 nm was measured. Each experiment was independently repeated five times.
Motility test
Surface motility was examined on Mueller Hinton (MH) medium containing 0.25% Eiken soft agar (Eiken Chemical, Tokyo, Japan). A. nosocomialis WT and anoI-deletion mutant cultivated in MH broth overnight were diluted to adjust the OD600 to 1, and 5 μl of each sample was spotted onto the center of the motility plate, with (40 μM) or without exogenous AHLs. The plates were then incubated at 30°C for 18 h. Motility on the agar surface was observed and compared. Plates were prepared fresh for each surface motility experiment, and the test was repeated on at least three separate occasions.
Quantitative real-time PCR analysis
Overnight subcultures of A. nosocomialis WT and anoI-deletion mutant were added to 10 ml of LB medium with (40 or 80 μM) and without AHLs (C6-HSL, C10-HSL and C12-HSL) and incubated under steady-state culture conditions until the OD600 1.5. Total RNA was extracted using a High Pure RNA Isolation Kit (Roche Diagnostics GmbH, Germany) according to the manufacturer’s instructions. cDNA was synthesized from 1 mg of DNase-treated total RNA using Reverse Transcription Master Premix (Elpis Biotech Inc., Korea) as per the manufacturer’s instructions. Quantitative RT-PCR was performed using a Bio-Rad CFX Real-Time PCR system with Bio-Rad CFX Maestro software (Bio-Rad, Hercules, CA, USA) and the indicated primers (Table 2).
Data analysis and statistics
Data presented in this study represent the results from at least three independent experiments that yielded consistent results. The significance of differences between two groups was determined using unpaired Student’s t-test, and differences among more than three groups were evaluated using a one-way analysis of variance (ANOVA) followed by Tukey’s multiple comparison test using Graph-Pad Prism Software (ver.5.01; GraphPad Software, San Diego, CA, USA).
RESULTS
Confirmation of the anoI-deletion mutant
The anoI-deletion mutant was confirmed by PCR of anoI gene (Fig. 1A) using the primer sets AnoIF and AnoR. 0.2 Kb PCR product of anoI gene was detected from WT, whereas none from anoI mutant. To determine whether deletion of anoI affected the growth of A. nosocomialis, we compared the growth in LB broth (Fig. 1B). The kinetics of growth of the anoI-deletion mutant were not different from those of the WT, suggesting that growth of these bacterial cells is not dependent on the product of the anoI gene. We also found that anoI mRNA was not expressed in the anoI-deletion mutant, further confirming deletion of the anoI gene (Fig. 1C).
Fig. 1
Confirmation of the anoI-deletion mutant. (A) Gel image showing PCR analysis of the WT and anoI-deletion mutant (∆anoI), confirming deletion of the target gene. (B) Representative growth curves for the WT and anoI-deletion mutant. (C) anoI mRNA expression was nearly absent in the anoI-deletion mutant. ***P < 0.001 versus WT.
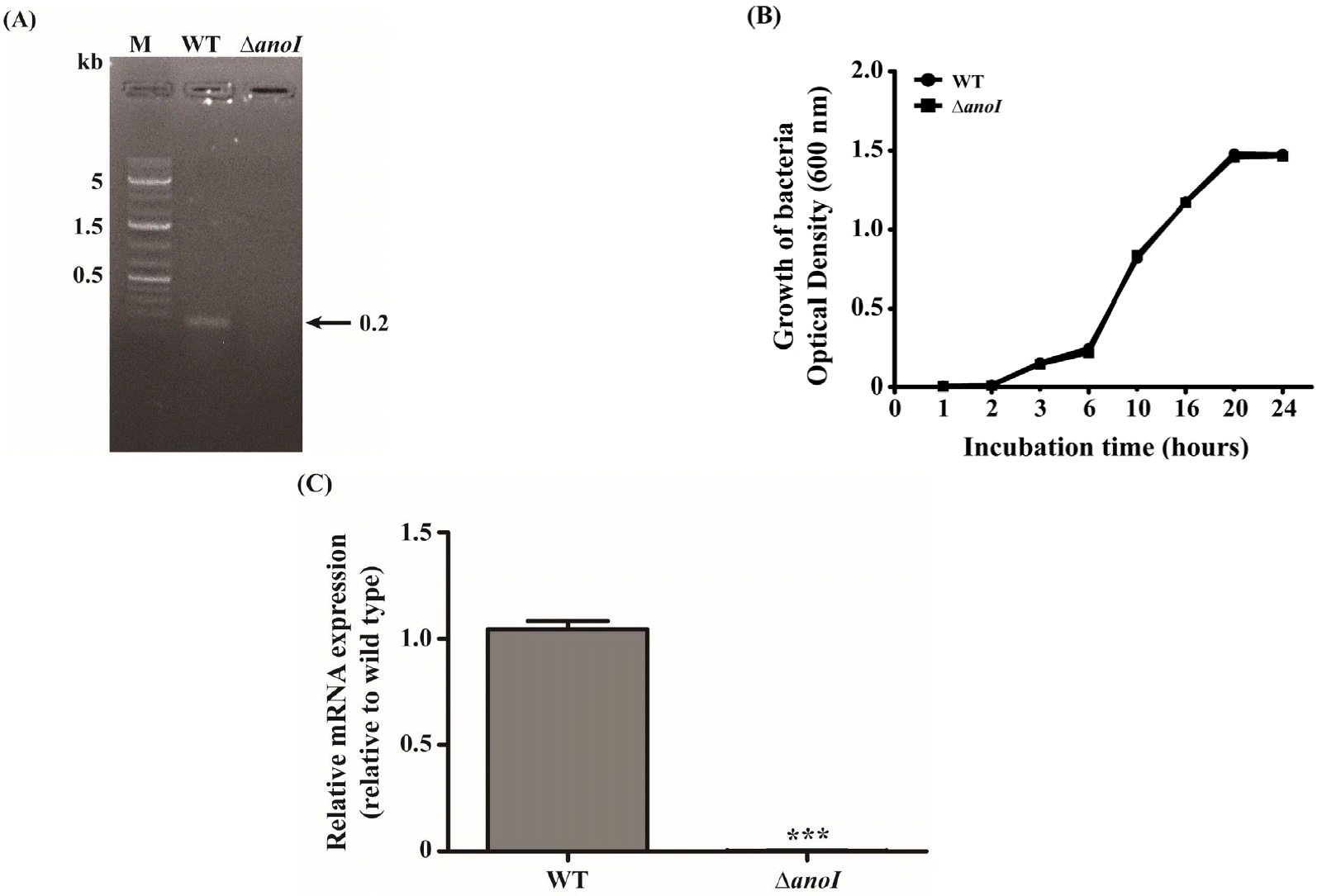
Effects of exogenous AHLs on anoR expression
To study the impact of anoI-deletion in A. nosocomialis, we compared changes in the levels of anoR mRNA in the anoI-deletion mutant with those in the WT strain following treatment with different types of AHL. Under baseline conditions, expression of anoR mRNA was reduced in the anoI-deletion mutant compared with that in the WT strain, a finding clearly consistent with our previous report (7). However, exogenously applied AHLs especially 40 μM greatly stimulated the expression of anoR mRNA in the anoI-deletion mutant (Fig. 2A, Fig. 2B, Fig. 2C), hence, 40 μM was set as the standard concentration for subsequent experiments on AHLs in this study. When the mutant was incubated with 40 μM C6-HSL, C10-HSL, or C12-HSL, anoR expression was up-regulated at 1.3, 2.0, or 2.65 times compared to the untreated anoI mutant. The highest anoR expression difference was observed with C12-HSL (Fig. 2C).
Fig. 2
Relative mRNA expression of anoR in the absence and presence of the indicated concentration (40 and 80 mM) and type of AHL. (A–D) anoR mRNA expression in the WT and anoI-deletion mutant following exposure to different concentrations of C6-HSL (A), C10-HSL (B) or C12-HSL (C). The differences among different sample groups were evaluated using a one-way analysis of variance (ANOVA) followed by Tukey’s multiple comparison test using Graph-Pad Prism Software. ***P < 0.001 versus the anoI-deletion mutant.
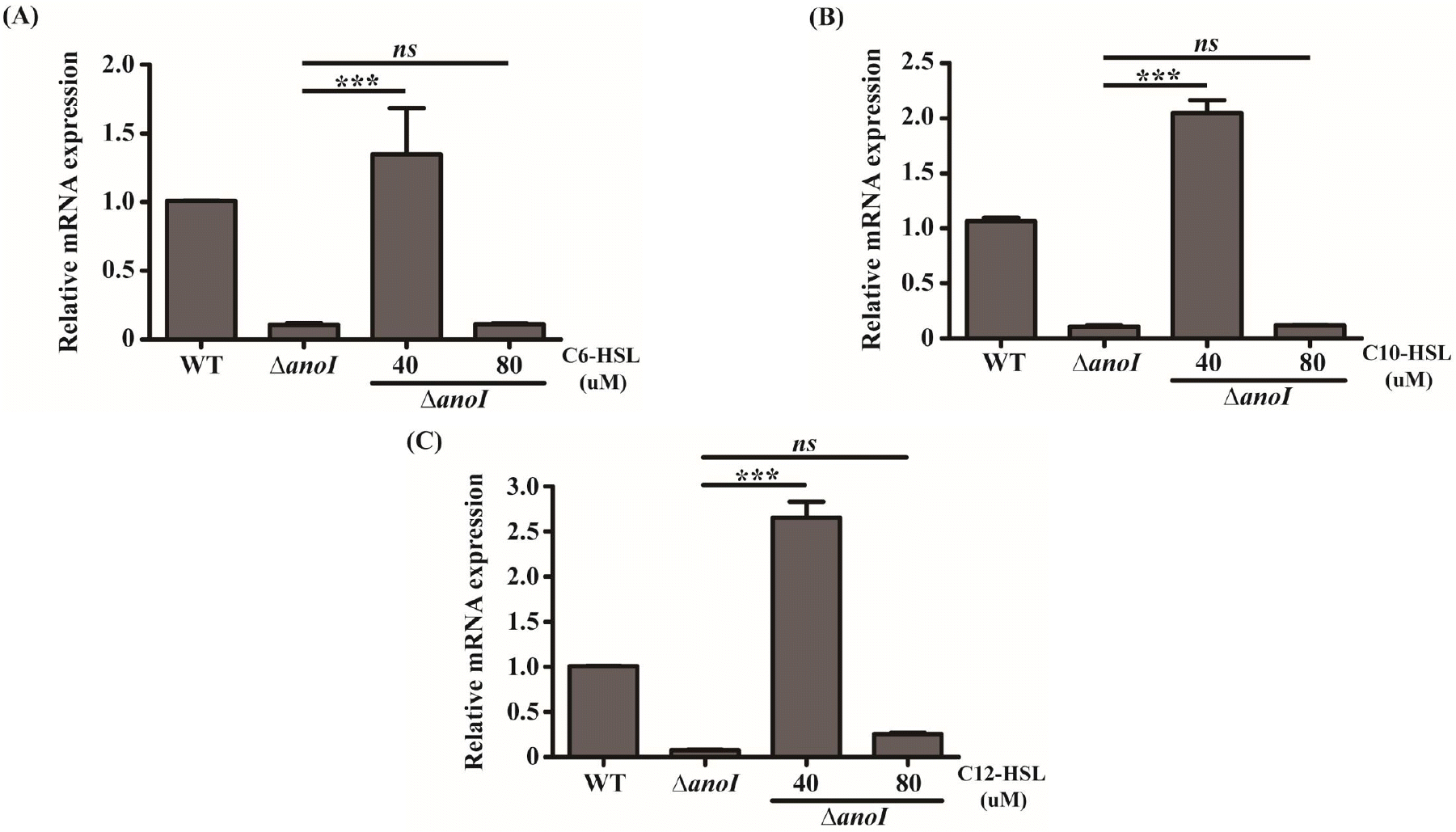
Biofilm formation and motility tests
To examine the effects of exogenous AHLs on biofilm formation in the anoI-deletion mutant, we employed crystal violet staining. Crystal violet staining showed reduced biofilm formation by the anoI-deletion mutant compared with the WT strain. Notably, addition of exogenous AHLs to the anoI-deletion mutant increased biofilm development, as shown in Fig. 3A. Quantification of biofilm formation indicated that the greatest increase occurred in the presence of exogenous C12-HSL, which restored biofilm-formation ability to WT levels. Although the treatment of C10 or C6-HSL enhance the biofilm formation of anoI-deletion mutant, relative levels of biofilm was lower than C12-HSL treated anoI-deletion mutant (Fig. 3B). To test motility, we spotted overnight cultures of A. nosocomialis WT and the anoI-deletion mutant onto MH medium containing 0.25% Eiken soft agar, with or without exogenous AHLs. Motility was restored in anoI-deletion mutants treated with C12-HSL. In contrast, C6-HSL and C10-HSL show no effect on the motility of the anoI-deletion mutant, possibly indicating that suppression of the motility phenotype may reflect a certain threshold activity of a particular AHL. To better understand this relationship, we combined C12-HSL with C6-HSL or C10-HSL, and observed that each combination restored motility, suggesting a synergistic effect of AHLs. These data indicate that C12-HSL is the predominant AHL type involve in biofilm formation and motility phenotypes in A. nosocomialis.
Fig. 3
Biofilm formation and motility. (A) Crystal violet staining of biofilm formed by the WT or anoI-deletion mutant in the presence (40 μM) and absence of exogenous AHLs. (B) Graphical representation of biofilm formation, quantified as absorbance at OD590 nm. The differences among different sample groups were evaluated using a one-way analysis of variance (ANOVA) followed by Tukey’s multiple comparison test using Graph-Pad Prism Software. (C) Motility assay of the WT in the absence of exogenous AHLs and of the anoI-deletion mutant in both the absence and presence of AHLs. **P < 0.01 and ***P < 0.001 versus the anoI-deletion mutant.
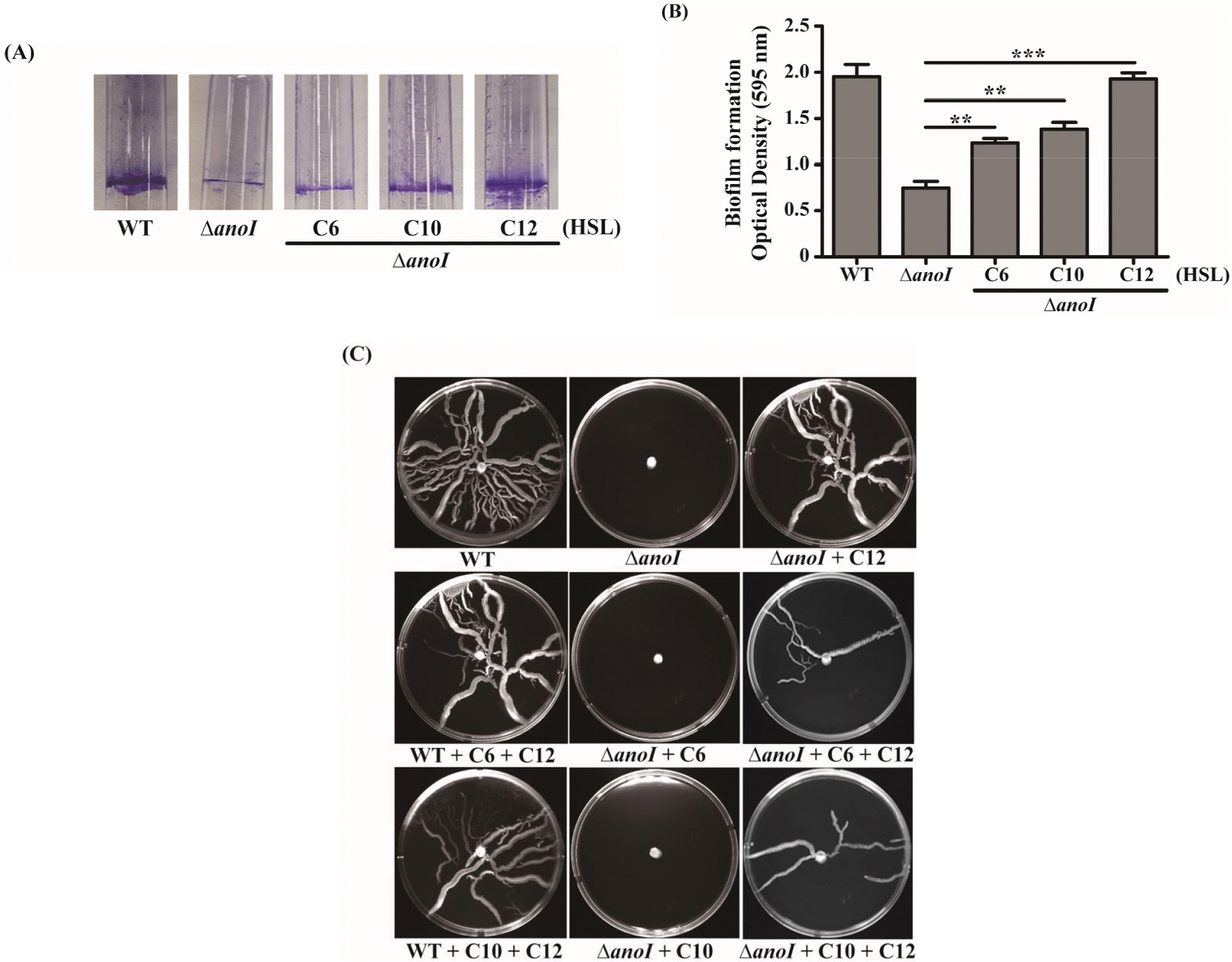
Expression of csuC, csuD and pilT
To further understand the QS mechanism with respect to the genetic basis of biofilm formation and motility, we assessed the effects of exogenous AHLs on three biofilm related genes, csuC, csuD and pilT. The mRNA expression of these genes were significantly increased in anoI-deletion mutant treated with C6-, 10-, or C12-HSL compared to the mutant without AHLs. Among AHLs, C12-HSL induced the highest expression of csuC, csuD and pilT genes (Fig. 4). These data show that exogenous AHLs affect the expression of genes related to biofilm and motility and that C12-HSL is the most prominent QS molecule in A. nosocomialis.
Fig. 4
Relative mRNA expression of various genes related to biofilm formation and motility. Expression of csuC, csuD and pilT genes in the WT and anoI-deletion mutant strain in the presence (40 μM) and absence of exogenous AHLs, measured by qRT-PCR. The differences among different sample groups were evaluated using a one-way analysis of variance (ANOVA) followed by Tukey’s multiple comparison test using Graph-Pad Prism Software. *P < 0.05, **P < 0.01 and ***P < 0.001 versus the anoI-deletion mutant.
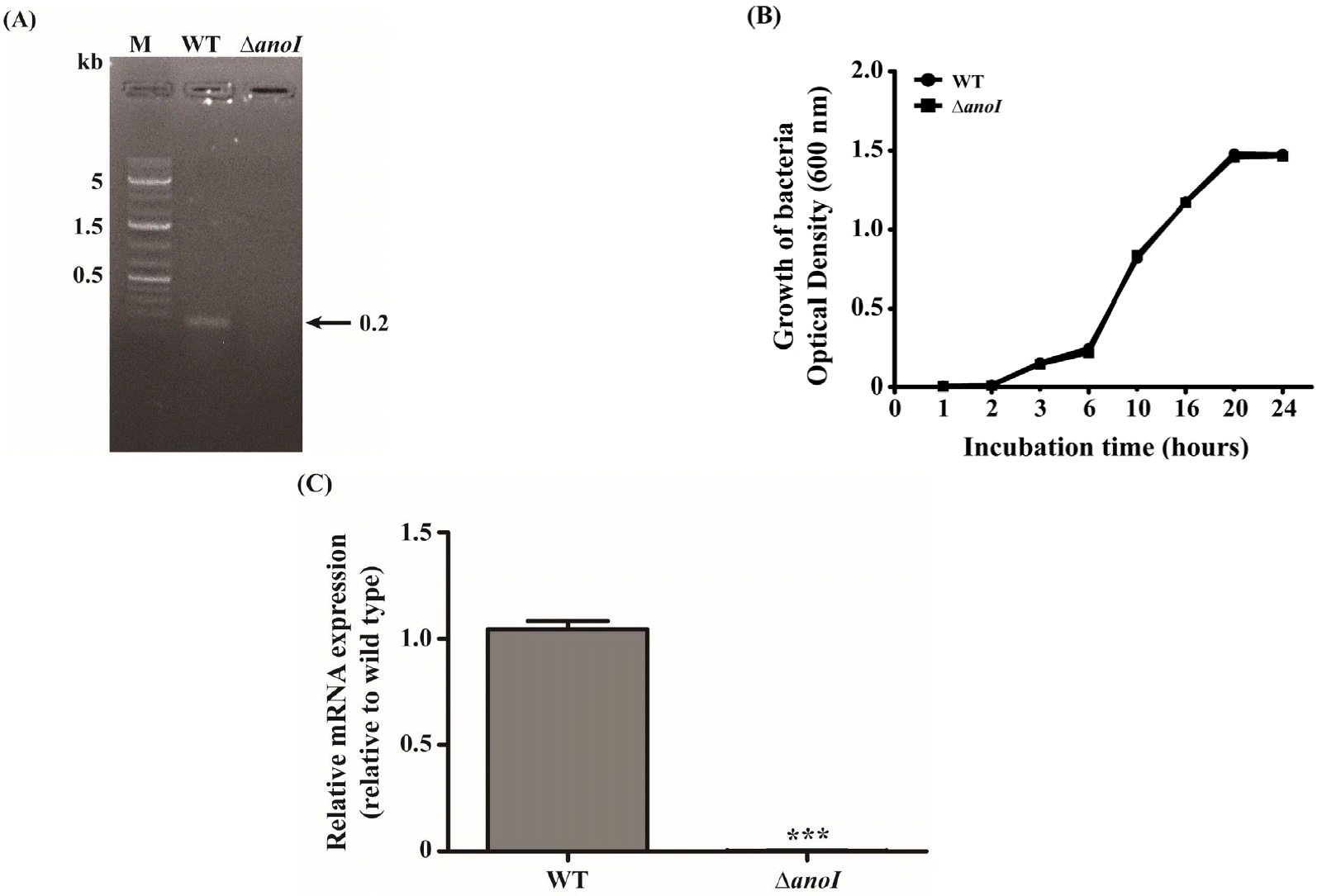
DISCUSSION
In this study, we found that different types of AHLs differentially affect QS gene activity in the A. nosocomialis strain in an anoI-deleted setting, exerting effects on biofilm formation and motility. Previous studies have reported that when local AHL levels are high, AHLs diffuse back into cells, resulting in expression of the anoR gene (16). Hence, to study the regulation of biofilm formation and motility in A. nosocomialis, we used different types of AHLs—specifically, C6-HSL, C10-HSL and C12-HSL—that have been identified in other Acinetobacter strains. In this study, we found that expression of the anoR gene in the anoI-deletion mutant requires induction by exogenous AHLs (Fig. 2), with a concentration of 40 μM exerting the greatest stimulatory effect (17).
The QS system makes a major contribution to biofilm formation and motility, both of which are important virulence factors. In this study, biofilm formation was enhanced by addition of exogenous AHLs, with C12-HSL causing the greatest increase in biofilm formation. In the case of motility, only exogenous C12-HSL, or a combination of C12-HSL with other AHL types, restored motility (Fig. 3C) (18). The inability of C6-HSL and C10-HSL to support motility was correlated with their limited induction of csuC, csuD and pilT genes. These relationships may indicate that exogenous AHL types have an abrupt threshold below which they are unable to activate certain genes or operons to express a certain phenotype (19), (20).
It has been reported that genes such as csuC, csuD and pilT, are known to be involved in biofilm formation and motility. In the current study, these genes exhibited increased mRNA expression upon addition of exogenous AHLs in the QS-deficient mutant, ∆anoI (Fig. 4). This result is similar to that reported for Hafnia alvei and Sinorhizobium meliloti (8) (21), in which the QS genes, luxI and sinI, respectively, were deleted. Previous studies on the activity of AHLs in the QS system (8), (22), (23) reported that different AHLs may regulate different phenotypes in bacteria. We found that among the exogenous AHLs tested, the C12-HSL depicted increased mRNA expression of csuC, csuD and pilT genes in the anoI-deletion mutant (Fig. 4) indicating that C12-HSL could be the key AHL for biofilm and motility in A. nosocomialis.
Conclusions
The AHL-dependent QS system plays a significant role in biofilm formation and motility in A. nosocomialis. In this study, we showed that addition of exogenous AHLs to an A. nosocomialis strain lacking the QS gene, anoI, rescued the mutant phenotype and restored anoR expression levels. Among the different AHLs tested in this study, C12-HSL exerted a significant impact on biofilm formation and the expression of various biofilm and motility-related genes. Notably, C12-HSL was the only AHL that rescued motility in the anoI-deletion mutant strain, but the combination of C12-HSL with C6-HSL or C10-HSL was able to restore motility owing to possible synergistic effects of the AHLs. This suggests that the motility phenotype in A. nosocomialis might be regulated only by C12-HSL, as it may be the most abundant AHL produced by this bacterium. Further studies focusing on the regulation of AHLs in A. nosocomialis will be important in understanding the QS system, particularly as it relates to the potential to target anoI/R gene with QS inhibitors, as to design better strategies to control the spread of this infection in the environment.