Abstract
Actinobacillus pleuropneumoniae (APP) causes a form of porcine pleuropneumonia that leads to significant economic losses in the swine industry worldwide. The apxIBD gene is responsible for the secretion of the ApxI and ApxII toxins and the pnp gene is responsible for the adaptation of bacteria to cold temperature and a virulence factor. The apxIBD and pnp genes were deleted successfully from APP serotype 1 and 5 by transconjugation and sucrose counter-selection. The APP1Δ apxIBDΔ pnp and APP5Δ apxIBDΔ pnp mutants lost hemolytic activity and could not secrete ApxI and ApxII toxins outside the bacteria because both mutants lost the ApxI- and ApxII-secreting proteins by deletion of the apxIBD gene. Besides, the growth of these mutants was defective at low temperatures resulting from the deletion of pnp. The APP1Δ apxIBDΔ pnp and APP5Δ apxIBDΔ pnp mutants were significantly attenuated compared with wild-type ones. However, mice vaccinated intraperitoneally with APP5Δ apxIBDΔ pnp did not provide any protection when challenged with a 10-times 50% lethal dose of virulent homologous (APP5) and heterologous (APP1) bacterial strains, while mice vaccinated with APP1Δ apxIBDΔ pnp offered 75% protection against a homologous challenge. The Δ apxIBDΔ pnp mutants were significantly attenuated and gave different protection rate against homologous virulent wild-type APP challenging.
References
1. Sassu EL, Bossé JT, Tobias TJ, Gottschalk M, Langford PR, Hennig-Pauka I. Update on Actinobacillus pleuropneumoniae-knowledge, gaps and challenges. Transbound Emerg Dis. 2018; 65(Suppl 1):72–90.


2. Bossé JT, Li Y, Sárközi R, Fodor L, Lacouture S, Gottschalk M, Casas Amoribieta M, Angen Ø, Nedbalcova K, Holden MT, Maskell DJ, Tucker AW, Wren BW, Rycroft AN, Langford PR. BRaDP1T consortium. Proposal of serovars 17 and 18 of Actinobacillus pleuropneumoniae based on serological and genotypic analysis. Vet Microbiol. 2018; 217:1–6.


3. Lee KE, Choi HW, Kim HH, Song JY, Yang DK. Prevalence and characterization of Actinobacillus pleuropneumoniae isolated from Korean pigs. J Bacteriol Virol. 2015; 45:19–25.


4. Komal JP, Mittal KR. Grouping of Actinobacillus pleuropneumoniae strains of serotypes 1 through 12 on the basis of their virulence in mice. Vet Microbiol. 1990; 25:229–240.


5. Yuan F, Liu J, Guo Y, Tan C, Fu S, Zhao J, Chen H, Bei W. Influences of ORF1 on the virulence and immunogenicity of Actinobacillus pleuropneumoniae. Curr Microbiol. 2011; 63:574–580.


6. Frey J. Virulence in Actinobacillus pleuropneumoniae and RTX toxins. Trends Microbiol. 1995; 3:257–261.
7. Jansen R, Briaire J, Kamp EM, Gielkens AL, Smits MA. Structural analysis of the Actinobacillus pleuropneumoniae-RTX-toxin I (ApxI) operon. Infect Immun. 1993; 61:3688–3695.


8. Jones GH. Novel aspects of polynucleotide phosphorylase function in Streptomyces. Antibiotics (Basel). 2018; 7:25.
9. Barria C, Malecki M, Arraiano CM. Bacterial adaptation to cold. Microbiology. 2013; 159:2437–2443.


10. Rosenzweig JA, Chopra AK. The exoribonuclease polynucleotide phosphorylase influences the virulence and stress responses of yersiniae and many other pathogens. Front Cell Infect Microbiol. 2013; 3:81.


11. De Lay N, Gottesman S. Role of polynucleotide phosphorylase in sRNA function in Escherichia coli. RNA. 2011; 17:1172–1189.


12. Cameron TA, Matz LM, De Lay NR. Polynucleotide phosphorylase: not merely an RNase but a pivotal post-transcriptional regulator. PLoS Genet. 2018; 14:e1007654.


13. Fuller TE, Kennedy MJ, Lowery DE. Identification of Pasteurella multocida virulence genes in a septicemic mouse model using signature-tagged mutagenesis. Microb Pathog. 2000; 29:25–38.


14. Engman J, Negrea A, Sigurlásdóttir S, Geörg M, Eriksson J, Eriksson OS, Kuwae A, Sjölinder H, Jonsson AB. Neisseria meningitidis polynucleotide phosphorylase affects aggregation, adhesion, and virulence. Infect Immun. 2016; 84:1501–1513.


15. Fuller TE, Martin S, Teel JF, Alaniz GR, Kennedy MJ, Lowery DE. Identification of Actinobacillus pleuropneumoniae virulence genes using signature-tagged mutagenesis in a swine infection model. Microb Pathog. 2000; 29:39–51.


16. Oswald W, Tonpitak W, Ohrt G, Gerlach G. A single-step transconjugation system for the introduction of unmarked deletions into Actinobacillus pleuropneumoniae serotype 7 using a sucrose sensitivity marker. FEMS Microbiol Lett. 1999; 179:153–160.


17. Lin L, Bei W, Sha Y, Liu J, Guo Y, Liu W, Tu S, He Q, Chen H. Construction and immunogencity of a ΔapxIC/ΔapxIIC double mutant of Actinobacillus pleuropneumoniae serovar 1. FEMS Microbiol Lett. 2007; 274:55–62.


18. Hu J, McCormick RJ, Means WJ, Zhu MJ. Polynucleotide phosphorylase is required for Escherichia coli O157: H7 growth above refrigerated temperature. Foodborne Pathog Dis. 2014; 11:177–185.


19. Nielsen R, van den Bosch JF, Plambeck T, Sørensen V, Nielsen JP. Evaluation of an indirect enzyme-linked immunosorbent assay (ELISA) for detection of antibodies to the Apx toxins of Actinobacillus pleuropneumoniae. Vet Microbiol. 2000; 71:81–87.


20. Xu F, Chen X, Shi A, Yang B, Wang J, Li Y, Guo X, Blackall PJ, Yang H. Characterization and immunogenicity of an apxIA mutant of Actinobacillus pleuropneumoniae. Vet Microbiol. 2006; 118:230–239.


21. Bei W, He Q, Yan L, Fang L, Tan Y, Xiao S, Zhou R, Jin M, Guo A, Lv J, Huang H, Chen H. Construction and characterization of a live, attenuated apxIICA inactivation mutant of Actinobacillus pleuropneumoniae lacking a drug resistance marker. FEMS Microbiol Lett. 2005; 243:21–27.


22. Liu J, Chen X, Lin L, Tan C, Chen Y, Guo Y, Jin M, Guo A, Bei W, Chen H. Potential use an Actinobacillus pleuropneumoniae double mutant strain ΔapxIICΔapxIVA as live vaccine that allows serological differentiation between vaccinated and infected animals. Vaccine. 2007; 25:7696–7705.


23. Seah JN, Frey J, Kwang J. The N-terminal domain of RTX toxin ApxI of Actinobacillus pleuropneumoniae elicits protective immunity in mice. Infect Immun. 2002; 70:6464–6467.


24. Frey J. The role of RTX toxins in host specificity of animal pathogenic Pasteurellaceae. Vet Microbiol. 2011; 153:51–58.


25. Ramjeet M, Deslandes V, Gouré J, Jacques M. Actinobacillus pleuropneumoniae vaccines: from bacterins to new insights into vaccination strategies. Anim Health Res Rev. 2008; 9:25–45.


26. Yuan F, Liao Y, You W, Liu Z, Tan Y, Zheng C, Wang Bin, Zhou D, Tian Y, Bei W. Deletion of the znuA virulence factor attenuates Actinobacillus pleuropneumoniae and confers protection against homologous or heterologous strain challenge. Vet Microbiol. 2014; 174:531–539.


27. Xie F, Li G, Zhou L, Zhang Y, Cui N, Liu S, Wang C. Attenuated Actinobacillus pleuropneumoniae double-deletion mutant S-8∆clpP/apxIIC confers protection against homologous or heterologous strain challenge. BMC Vet Res. 2017; 13:14.
28. Wang L, Qin W, Yang S, Zhai R, Zhou L, Sun C, Pan F, Ji Q, Wang Y, Gu J, Feng X, Du C, Han W, Langford PR, Lei L. The Adh adhesin domain is required for trimeric autotransporter Apa1-mediated Actinobacillus pleuropneumoniae adhesion, autoaggregation, biofilm formation and pathogenicity. Vet Microbiol. 2015; 177:175–183.


29. Fu S, Ou J, Zhang M, Xu J, Liu H, Liu J, Yuan F, Chen H, Bei W. The live attenuated Actinobacillus pleuropneumoniae triple-deletion mutant ΔapxIC ΔapxIIC ΔapxIV-ORF1 strain, SLW05, Immunizes pigs against lethal challenge with Haemophilus parasuis. Clin Vaccine Immunol. 2013; 20:134–139.


30. Chiers K, De Waele T, Pasmans F, Ducatelle R, Haesebrouck F. Virulence factors of Actinobacillus pleuropneumoniae involved in colonization, persistence and induction of lesions in its porcine host. Vet Res. 2010; 41:65.


31. Park C, Ha Y, Kim S, Chae C, Ryu DY. Construction and characterization of an Actinobacillus pleuropneumoniae serotype 2 mutant lacking the Apx toxin secretion protein genes apxIIIB and apxIIID. J Vet Med Sci. 2009; 71:1317–1323.


32. Bao CT, Xiao JM, Liu BJ, Liu JF, Zhu RN, Jiang P, Li L, Langford PR, Lei LC. Establishment and comparison of Actinobacillus pleuropneumoniae experimental infection model in mice and piglets. Microb Pathog. 2019; 128:381–389.


33. Li Y, Cao S, Zhang L, Yuan J, Zhao Q, Wen Y, Wu R, Huang X, Yan Q, Huang Y, Ma X, Han X, Miao C, Wen X. A requirement of TolC1 for effective survival, colonization and pathogenicity of Actinobacillus pleuropneumoniae. Microb Pathog. 2019; 134:103596.
34. Li T, Zhang Q, Wang R, Zhang S, Pei J, Li Y, Li L, Zhou R. The roles of flp1 and tadD in Actinobacillus pleuropneumoniae pilus biosynthesis and pathogenicity. Microb Pathog. 2019; 126:310–317.


35. Loera-Muro A, Angulo C. New trends in innovative vaccine development against Actinobacillus pleuropneumoniae. Vet Microbiol. 2018; 217:66–75.


36. Inzana TJ, Todd J, Ma JN, Veit H. Characterization of a non-hemolytic mutant of Actinobacillus pleuropneumoniae serotype 5: role of the 110 kilodalton hemolysin in virulence and immunoprotection. Microb Pathog. 1991; 10:281–296.


Fig. 1.
PCR analysis of the wild-type and apxIBD, pnp-deleted mutant of APP1 (A) and APP5 (B) using primer pairs P5–P6 and P11–P12. Lane M, DNA molecular weight ladder 1 kb; lane 1, negative control; lane 2, apxIBD gene amplified from genomic DNA of wild-type APP; lane 3, apxIBD gene amplified from genomic DNA of the Δ apxIBDΔ pnp mutant; lane 4, pnp gene amplified from genomic DNA of the wild-type APP; lane 5, pnp gene amplified from genomic DNA of Δ apxIBDΔ pnp mutant. PCR, polymerase chain reaction; APP, Actinobacillus pleuropneumoniae.
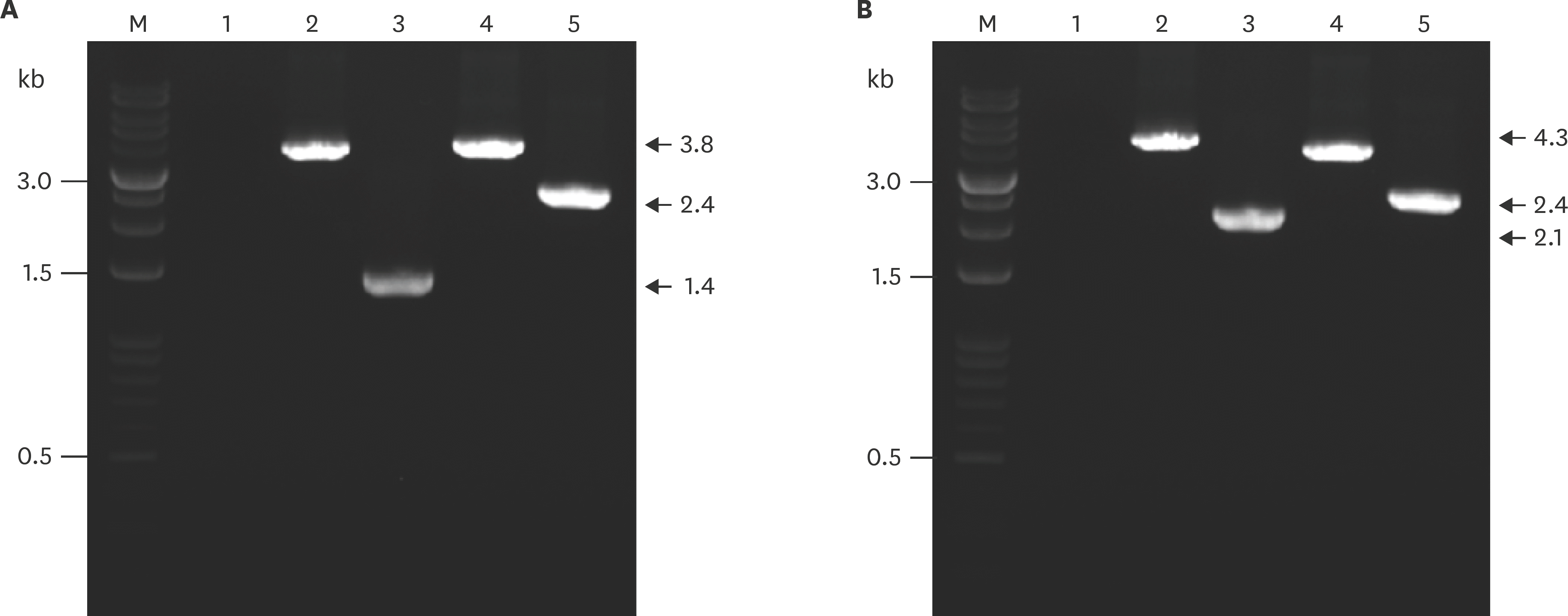
Fig. 2.
Phenotypes of the APP mutants. Hemolytic activity of wild-type and mutants of APP1 (A) and APP5 (B). The black arrow indicates the clear zone caused by the hemolytic activity surrounding bacteria on blood agar. Cold shock adaptive ability of wild-type and mutant APP1 (C) and APP5 (D). Open arrow indicates colony growth on BHI agar containing a final amount of 10 µg/mL NAD. APP, Actinobacillus pleuropneumoniae; BHI, brain heart infusion; NAD, nicotinamide adenine dinucleotide.
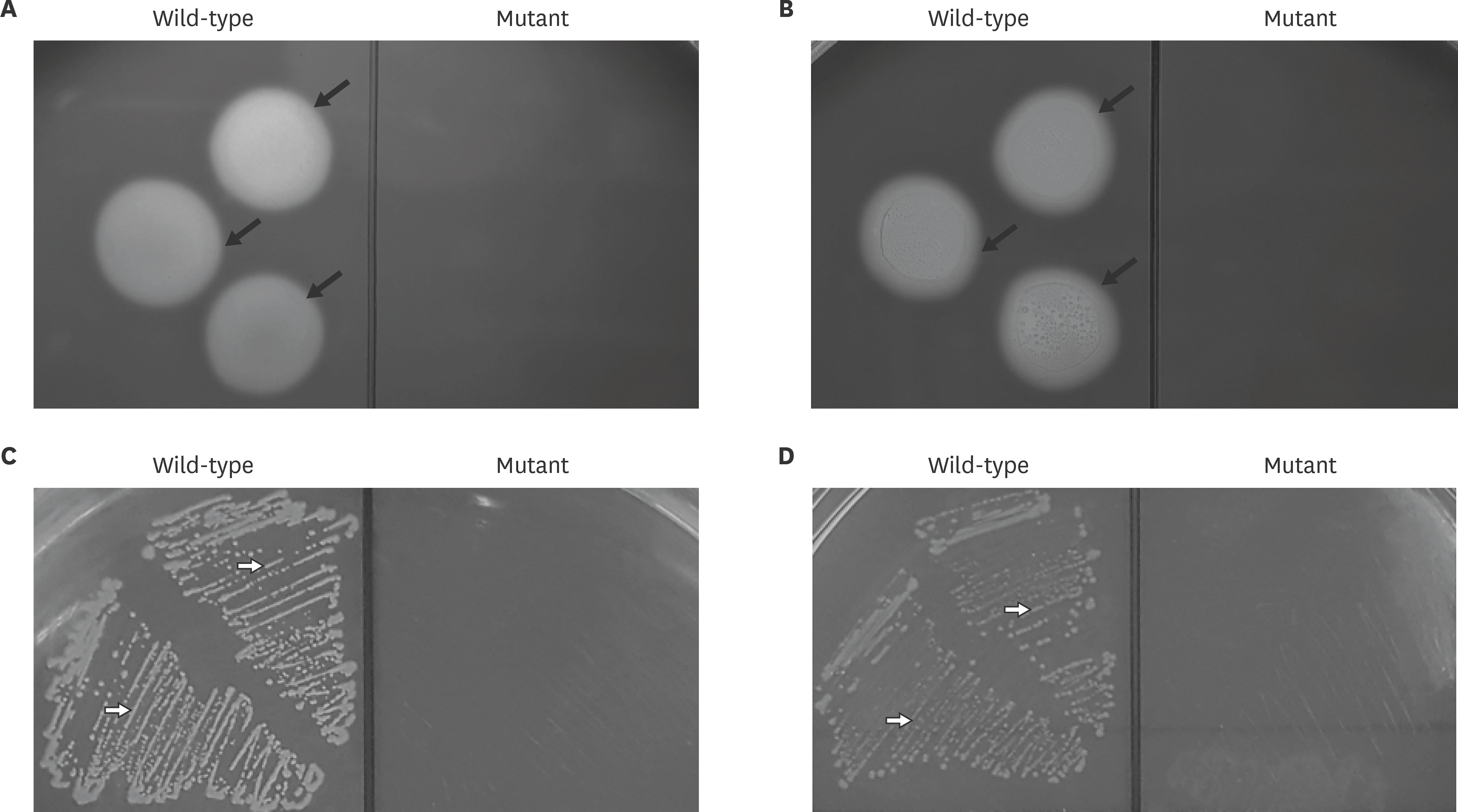
Fig. 3.
Growth curves of wild-type and mutants of APP1 (A) and APP5 (B). Symbols: ο, wild-type APP; ▲, mutant APP Δ apxIBDΔ pnp. APP, Actinobacillus pleuropneumoniae; OD600, optical density at 600 nm.
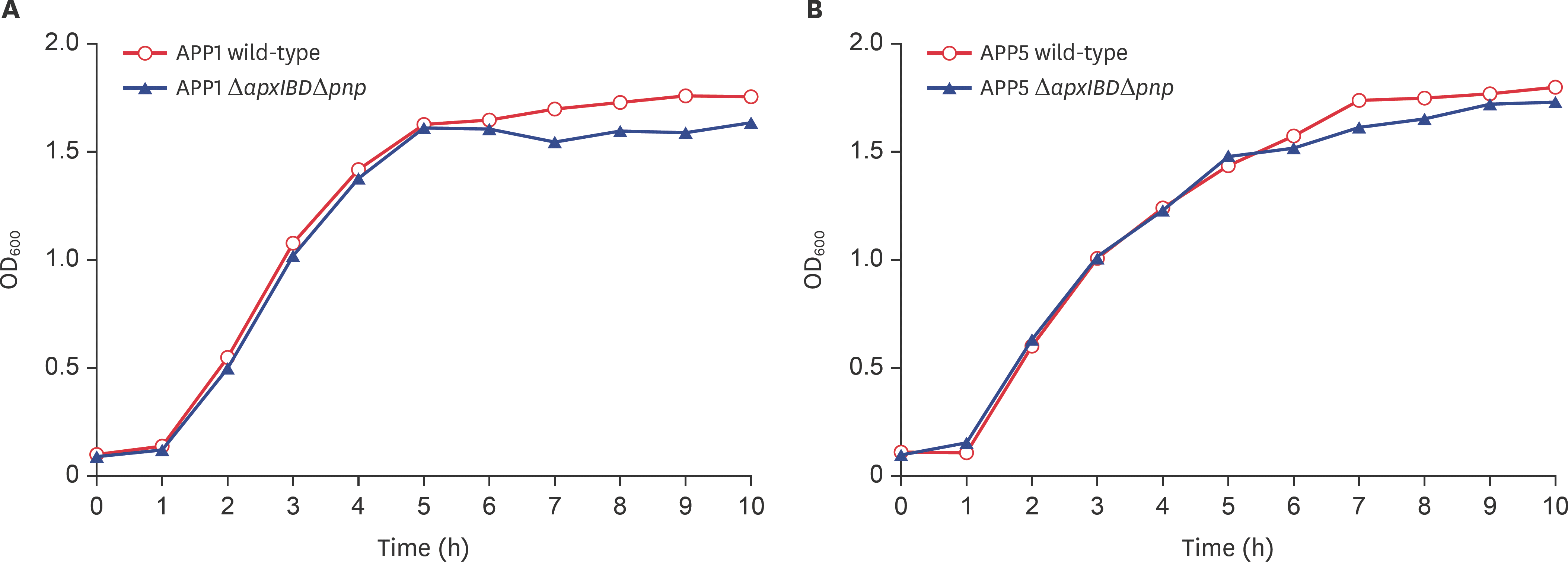
Fig. 4.
Sodium dodecyl sulfate-polyacrylamide gel electrophoresis of Apx toxins in wild-type and the mutants of APP1 (A) and APP5 (B). Lane M, Xpert Prestained Protein marker; lane 1, culture supernatant of the wild-type APP before precipitating; lane 2, culture supernatant of the mutants before precipitating; lane 3, RTX toxins of wild-type after precipitating with ammonium sulfate; lane 4, RTX toxins of the mutants after precipitating with ammonium sulfate. APP, Actinobacillus pleuropneumoniae; RTX, repeats in toxin.
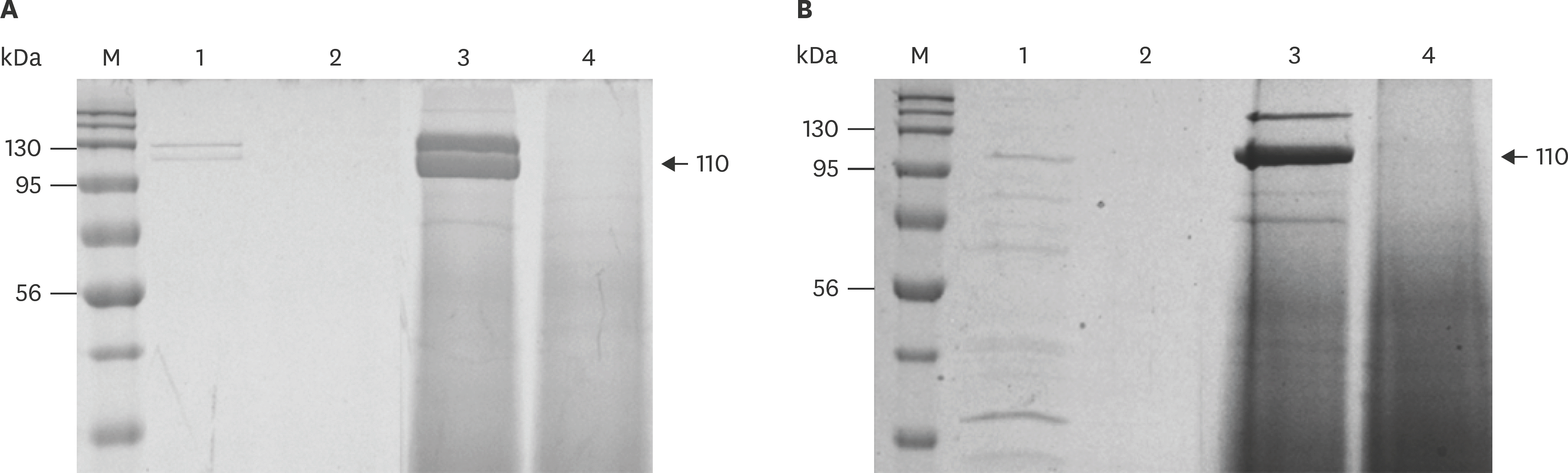
Fig. 5.
Evaluation of the stability deletion gene in the genome of APP1Δ apxIBDΔ pnp and APP5Δ apxIBDΔ pnp over 10 passages by PCR using primer pairs P5–P6 and P11–P12 for amplifying apxIBD and pnp genes, respectively. Amplified apxIBD gene (A) and pnp gene (B) from APP1Δ apxIBDΔ pnp. Amplified apxIBD gene (C) and pnp gene (D) from APP5Δ apxIBDΔ pnp. Lane M, DNA Ladder 1kb; lane 1, negative control; lane 2, target gene amplified from genomic DNA of wild-type APP, lanes 3–12, deletion gene amplified from genome DNA of mutant through 10 continuous passages. APP, Actinobacillus pleuropneumoniae; PCR, polymerase chain reaction.
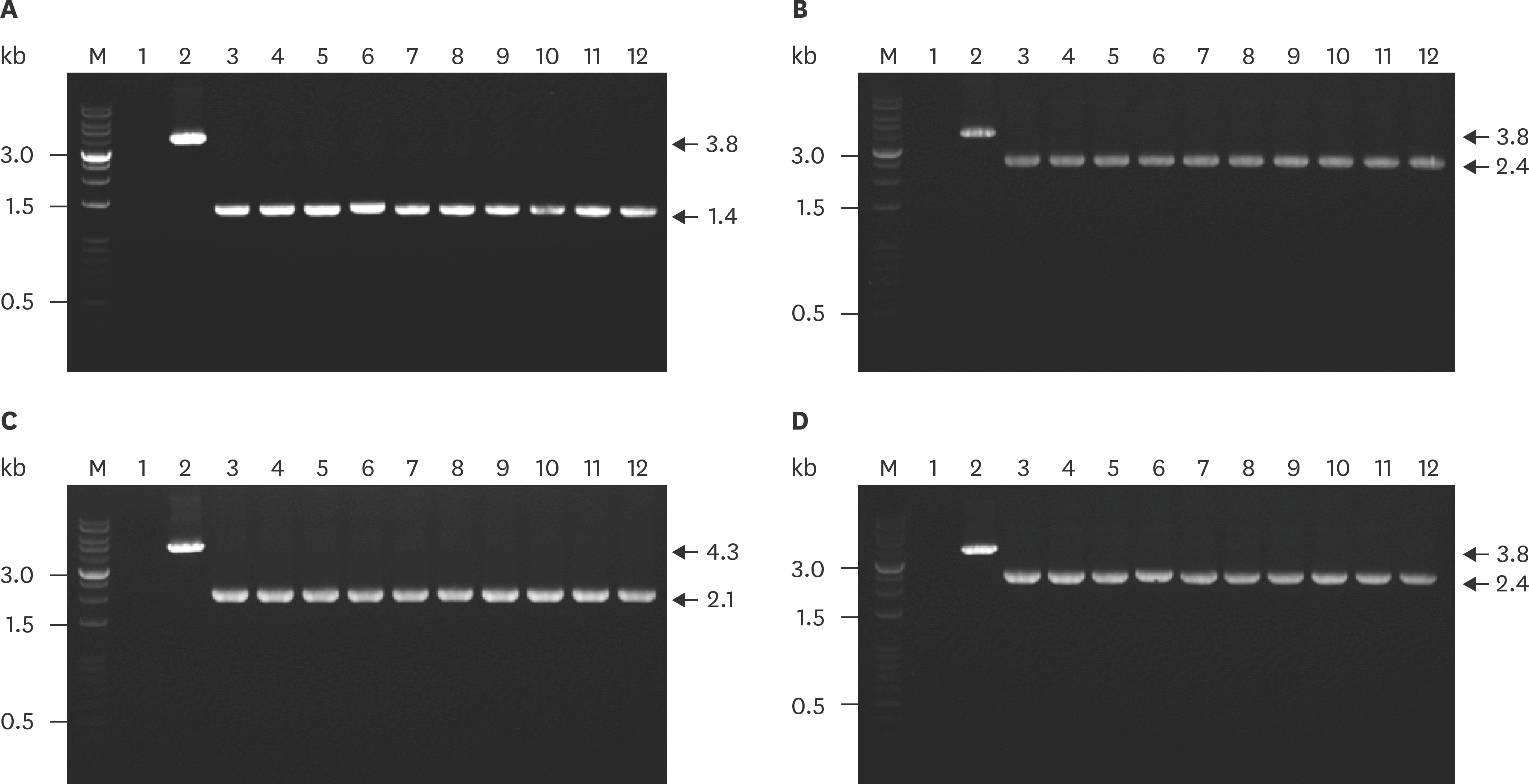
Table 1.
Bacterial strains used in this study
Table 2.
Plasmid and primers used in this study
Table 3.
Virulence of the wild-type and mutant of APP in mice*
Strain tested | LD50† (CFU) | Fold attenuation‡ |
---|---|---|
APP1 wild-type | 1.6 × 106 | 1 |
APP1Δ apxIBD | 6.3 × 107 | 39 |
APP1Δ pnp | 4.4 × 106 | 3 |
APP1Δ apxIBDΔ pnp | 1.5 × 108 | 93 |
APP5 wild-type | 6.5 × 106 | 1 |
APP5Δ apxIBD | 1.4 × 108 | 21 |
APP5Δ pnp | 1.6 × 107 | 2 |
APP5Δ apxIBDΔ pnp | 3.1 × 108 | 48 |
Table 4.
Protection of mice immunized with APP1 and APP5 mutants challenged with homologous and heterologous serotypes of APP*