Abstract
Bone health in prostate cancer patients represents a prerequisite for acceptable quality of life and optimal outcome of this disease. The major threat for bone health in prostate cancer displays cancer treatment induced bone loss as well as the development of bone metastases. In recent years, several new pharmaceuticals targeting bone metabolism such as denosumab or androgen pathway targeting drugs (abiraterone acetate and enzalutamide) have been approved for the treatment of progressive disease aiming to interrupt the vicious circle of bone metastasis and aberrant bone resorption. This development raised the awareness of the pivotal role of bone health in prostate cancer and introduced (symptomatic) skeletal related events as an important end point in recent clinical trials. Bone targeted drugs have become standard of care in patients with metastatic castration resistant prostate cancer, their role in metastatic hormone sensitive prostate cancer has been discussed controversely. In oligometastatic prostate cancer patients several promising approaches in metastasis directed therapy, including conventional surgery, stereotactic ablative radiation and image-guided single-fraction robotic stereotactic radiosurgery (CyberKnife®) were launched but are not in routine clinical use until now caused by sparse clinical evidence.
The maintenance of bone health in patients with prostate cancer (PC) is a well-known challenge especially in advanced stages of disease. Approximately 90% of patients with advanced PC will develop bone metastases (BM) [12]. BM as well as cancer treatment induced bone loss (CTIBL) can lead to impaired bone mineral density (BMD) and symptomatic skeletal related events (SRE), such as increased risk of pathological fractures, which are accompanied by pain, immobility and decreased quality of life (QoL) [3]. The occurrence of SREs are not only associated with a significantly impaired QoL and a substantial economic burden for the health system but also increased morbidity and especially mortality in patients with PC [456]. Therefore, current concepts in the treatment of PC focus on the prolongation of metastases-free survival and particularly on the prevention of SREs to provide optimal outcome for the patient [7].
This review aims to discuss the pathophysiology of bone complications induced by either CTIBL or BM and to discuss current concepts and trends in the maintenance of bone health in PC patients.
Bone health issues, especially decreased BMD, in PC patients are often linked to cancer treatment. A variety of mechanisms are involved in CTIBL. Chemotherapy as well as androgen deprivation therapy (ADT) and glucocorticoids can induce CTIBL [8]. In patients with metastatic castration resistant prostate cancer (mCRPC) taxan-based chemotherapy represents a major option for treatment [7]. Taxane chemotherapy agents are known to induce myelosuppression [910]. Quach et al [11] recently showed the impact of myelosuppressive agents on bone metabolism in mice. Myelosuppression induced a significant and persistent reduction in trabecular bone volume and a promotion of bone turnover favoring bone resorption. This led to increased expression of monocyte chemoattractant protein 1 (MCP1) and other inflammatory cytokines in mice. An increased expression of MCP1 was also detected in cancer patients with bone loss after chemotherapy [1112].
ADT is widely used in different settings and therapeutic regimens in PC patients. In total approximately 33% to 70% of all patients with PC will receive ADT in the course of their disease [13]. There are different pharmaceutical options including gonadotropin-releasing hormone agonists and antagonists, androgen receptor (AR) antagonists and 5α-reductase enzyme inhibitors to suppress androgen production or AR signaling in PC [1415]. ADT in PC patients causes a rapid loss of bone mass while testosterone itself reduces bone turnover [16]. Newer insights in the field of male osteoporosis indicate that bioavailable estradiol levels show a better correlation with male BMD and fracture risk than levels of testosterone [17]. Lowest levels of bioavailable estradiol and testosterone were associated with low BMD and increased fracture risk. Estradiol directly influences osteoclast and osteoblast activity via receptor-mediated pathways. One well known and therapeutically important pathway with regulatory involvement of estradiol is the receptor activator of nuclear factor-kappa B (RANK)/RANK ligand (RANKL)/osteoprotegerin (OPG) pathway which influences osteoclast activity [1516]. The pivotal role of estradiol on bone metabolism has been elucidated in estrogen deficiency where several osteoclastogenic cytokines (tumor necrosis factor [TNF], interleukin-6 [IL-6], IL-11, IL-17, and RANKL) showed upregulated expression while anti-osteoclastogenic factors, such as osteoprotegrin, were suppressed [16].
Glucocorticoids are substantial supportive drugs in taxane based chemotherapy in patients with PC. But glucocorticoids also account for severe side effects such as glucocorticoid induced osteoporosis. The predominant effect of glucocorticoids on bone metabolism is the impairment of bone formation by direct inhibition of osteoblast differentiation by suppressing Wnt protein signaling and inducing adipogenetic transcription factors (peroxisome proliferator-activated receptor γ) [18]. In addition, glucocorticoids also induce osteoblast as well as osteocyte apoptosis and impair their microenvironment [1418]. In contrast, glucocorticoids promote a prolonged lifespan of osteoclasts by increasing RANKL and decreasing osteoprotegrin expression in stromal cells as well as osteoblasts and herby lead to a resorption favoring bone metabolism [18]. Moreover, glucocorticoids show also indirect effects on bone metabolism by the reduced sex steroid production resulting in a hypogonadism which itself can induce increased bone resorption as mentioned above [1819].
Prior to initiation of any pharmaceutical cancer treatment each PC patient should be informed about the risk of CTIBL. To avoid bone loss after initiation of pharmaceutical cancer treatment including ADT in PC, patients should be evaluated with regard to fracture risk, BMD, serum vitamin D, and calcium levels before and during therapy (Fig. 1). Especially after long-term ADT, patients face a substantial risk to develop osteopenia and osteoporosis [7]. Lifestyle changes to avoid loss in BMD e.g., moderate physical exercise, avoidance of smoking and reduction of alcohol intake are recommended (Fig. 1). Unfortunately, evidence levels of the effects of these lifestyle changes are low and remain mainly unclear [2021]. Besides these lifestyle changes a daily supplementation of 1,000 to 1,200 mg/d calcium and 800 IU/d vitamin D is recommended for osteopenic diseases to reduce fracture risk (Fig. 1) [22]. It is reported that high daily calcium intake exceeding 2,000 mg/d for several years is associated with an increased incidence of PC as well as increased risk of advanced-stage and high-grade disease [23]. In contrast, there is no evidence that moderate supplementation of calcium and vitamin D as mentioned above promotes PC tumor progression [1524]. Currently, experts recommend the initiation of antiresorptive drugs in PC patients under ADT as soon as BMD T-score decreases under the threshold of −2.5 and one or more risk factors, or hip and vertebral fractures occur (Fig. 1) [7]. Antiresorptive drugs such as bisphosphonates and denosumab were most commonly evaluated in parallel with calcium and vitamin D supplementation [152425].
Bisphosphonates are an established pharmaceutical option to prevent or treat osteoporosis in PC patients receiving ADT. To clarify the role of bisphosphonates on fracture risk and BMD as well as the occurrence of side effects Serpa Neto et al [25] analyzed 15 studies with a total of 2.634 patients. They found a significantly reduced rate of fractures (risk ratio [RR], 0.80; p=0.005) and osteoporosis (RR, 0.39; p<0.00001) under bisphosphonate therapy. Intravenously administered zoledronic acid (ZA) revealed superior performance regarding fracture prevention (number needed to treat [NNT]=14.9) and development of osteoporosis (NNT=2.68) compared to orally administered pomidronate (NNT=38.4/NNT=3.06) and alendronate (NNT=41.6), respectively. The addition of bisphosphonates to ADT in PC patients and their above-mentioned benefits were reached without any major gastrointestinal or cardiovascular side effects. Osteonecrosis of the jaw (ONJ) occurred in a reduced rate compared to bisphosphonate treatment in mCRPC [1525].
The placebo controlled HALT trial investigated the efficacy of denosumab, a fully humanized monoclonal antibody that specifically binds to the receptor activator of nuclear factor-κB ligand, a key mediator of osteoclast formation, function, and survival in 1,468 PC patients (734 in the denosumab group and 734 in the placebo group) receiving ADT [24]. Primary end point of this study was the change in BMD at the lumbar spine after 4 applications of 60 mg denosumab subcutaneously, each administration after 6 months. Secondary end points included the incidence of new vertebral fractures. After 24 months BMD at the lumbar spine increased by 5.6% after administration of denosumab while placebo treated PC patients lost 1% of BMD at the lumbar spine. BMD increased in each target location including total hip and distal radius in PC patients after the application of denosumab and sustained after 36 months. The incidence of new vertebral fractures in the denosumab treated group (1.5%) were significantly reduced compared to the placebo group (3.9%) after 36 months (RR, 0.38; 95% confidence interval [CI], 0.19–0.78; p=0.006) [24]. Of note, adverse events were similar between both groups [1524]. The study results led to the approval of denosumab for the treatment of ADT-induced bone loss in patients at increased risk for fractures.
The application of ADT in PC patients also decreases the levels of bioavailable estradiol and herby influences and promotes bone loss, as mentioned above. Therefore, selective estrogen receptor modulators such as Toremifene were evaluated in a placebo-controlled phase III study. Primary end point was the occurrence of new vertebral fractures, secondary endpoints included changes of BMD. PC patients receiving ADT were assigned either to 80 mg toremifene daily or placebo. The authors found a significant risk reduction of 50% (95% CI, −1.5–75.0; p=0.05) in the toremifene group for the incidence of vertebral fractures and a significantly increased BMD at the lumbar spine, hip and femoral neck. Unfortunately, adverse events, in particular venous thromboembolic events, occurred more frequently in the toremifene group (2.6% versus 1.1% in placebo group) [26]. Therefore, no approval was granted for this drug for the management of CTIBL.
While several of the above mentioned antiresorptive agents, especially bisphosphonates and densoumab, are in clinical use to prevent and treat cancer induced bone loss, anabolic agents promoting bone formation are currently only available in clinical trials for several malignant diseases [1527]. Main objective of these therapeutic approaches is the maintenance of Wnt signaling to sustain bone formation including osteoblast differentiation. Antibody based approaches inhibiting sclerostin and Dickkopf homolog 1 (DKK1), functioning as inhibitors of Wnt signaling and osteoblast differentiation themselves, lead to increased bone formation [2728]. Sclerostin seems to represent a very interesting target in PC patients as it is described to show significantly elevated serum levels in PC patients especially when receiving ADT. In the phase 3 FRAME trial, remosozumab, a monoclonal antibody that binds sclerostin, achieved a significant risk reduction of 73% in postmenpausal women with osteoporosis to develop vertebral fractures compared to placebo [29]. The change on BMD under remosozumab in men with a T-score of ≤−2.5 or ≤−1.5 was evaluated in the phase III randomized BRIDGE trial and showed significantly increased BMD in the treatment group [30]. Manipulation in Wnt signaling has to be evaluated carefully in malignant diseases especially taking into consideration that increased Wnt signaling is known to be associated with several solid tumors such as colorectal cancer and others [31]. Moreover, anabolic approaches to increase bone formation by intermittent application of human parathyroid hormone 1-34 in murine prostate cancer models led to enhanced formation of osteoblastic lesions [1532].
The variety of above mentioned approaches in maintaining bone health in PC patients displays the relevance of bone turnover in this disease. A study by Fizazi et al [33] were PC patients with low concentrations of several bone-related parameters such as alkaline phosphatase, bone-specific alkaline phosphatase, corrected urinary N-telopeptide and others showed extended time to first bone metastasis and improved overall survival. Of note, Hahn et al [34] reported earlier that a failure of first-line ADT to suppress markers of bone-turnover was associated with significantly reduced time to first SRE.
These findings underscore the crucial importance of bone metabolism and its prognostic value in PC.
PC in a localized stage with a very good 5-year overall-survival is rarely lethal. In advanced stage PC nearly 90% of the patients develop BM with a dramatically decreased 5-year survival of 29.8% [1]. Within 2 years, nearly 42% of all PC patients with BM suffer from an SRE with above mentioned consequences [35]. While many approaches were launched and evaluated in order to prevent PC from spreading, pathophysiology of tumor dissemination in PC remains insufficiently understood. Several models to describe the metastatic cascade in PC are discussed [36]. Presumably, malignant PC cells represent a major component in this process exhibiting altered phenotypical properties such as decreased adhesiveness to their surroundings, increased migratory capacity and invasiveness. The most famous theory of epithelial-to-mesenchymal transformation (EMT) seems to be driven by altered cytoskeletal proteins such as decreased expression of E-catherin and increased N-catherin promoting impaired cell-cell adhesion and invasion [363738]. Homing of the circulating PC cells is putatively mediated by the expressed CXC-chemokine receptor 4, which accounts for chemotaxis towards bone marrow in hematopoietic stem cells [3639]. After extravasation into bone marrow, αVβ3 or α2β1 integrins convey the adhesion of PC cells to endothelial cells and other extracellular matrixes [364041]. Tumor cells reaching the bone microenvironment can either proliferate or remain in a state of dormancy which seems to be mediated by RANKL, TNF, and others [36]. Activated PC cells in bone microenvironment secret several osteoblast-activating factors, such as IL-6 and parathyroid hormone-related peptide. These activated osteoblasts themselves secrete RANKL which is also, among others, secreted by activated tumor cells, resulting in an accelerated differentiation and activation of osteoclasts. The activated osteoclasts release, among their boosted bone resorption, growths factors such as transforming growth factor β which promote further tumor growth and herby complete the viscous circle in PC [36]. As metastases in PC are predominantly osteoblastic in nature with impaired stability of aberrant produced bone, a molecular switch has to occur towards osteoblastic-dominated response in PC metastases. The mechanism of this switch is currently unknown but involvement of DKK1 is suggested, as expression is increased during early phase of BM [3642].
Besides the well-known antibody-based approach to target and inhibit RANKL, no further targeted therapy to burst the viscous circle of tumor dissemination, tumor proliferation and changes in bone metabolism in PC patients has yet been translated into approved clinical trials despite of promising preclinical and early clinical data.
Nearly every other patient with metastatic PC develops the first SRE within two years after diagnosis [35]. Despite antiresorptive treatment in metastatic PC patients 15% to 20% develop fractures in a period of 2 to 3 years [43]. To reduce the risk of SRE development in these patients therapeutic approaches using bone targeting therapies such as bisphosphonates, denosumab, or radium-223-dichloride but also systemically acting agents such as abiraterone, enzalutamide or the cytotoxic agents docetaxel and cabacitaxel were launched and evaluated with distinct efficacy profiles and side effects.
The first bone targeting agent in use on a regular base in PC patients is ZA which acts by inhibition of mevalonate pathway and herby mediating osteoclast apoptosis [44]. Approval of ZA was based on a placebo-controlled randomized phase 3 trial, where 4 mg and 8/4 mg intravenously administered ZA every 3 weeks significantly reduced the incidence of SREs in patients with mCRPC compared to placebo (44.2% versus 33.2%; 38.5% versus 33.2%). Additionally, time to first SRE was significantly prolonged under ZA (488 days versus 321 days; p=0.011) [1545]. In the same trial pain and analgesic scores showed a significant improvement in the treatment group while QoL and performance status did not differ between ZA and placebo. In contrast, ZA could not prolong time to first SRE in the ALLIANCE and STAMPEDE trial in hormone sensitive PC patients (31.9 months versus 29.8 months) [464748]. In an exploratory subgroup analysis of the ALLIANCE trial, ZA showed a trend towards prolonged time to first on study SRE in patients who suffered from an SRE in advance to study entry (hazard ratio [HR], 0.56). These findings need to be confirmed in further prospective trials for a final recommendation [48]. Overall survival could not be improved by intravenously administered ZA in metastatic PC irrespective of hormone status [45]. A further clinical context that has been discussed critically in the past is the potential prevention of BM by bisphosphonates. Wirth et al [49] observed the efficacy of intravenously administered ZA every 3 months in localized high-risk PC patient (prostate-specific antigen [PSA] ≥20 ng/mL, node-positive disease, or Gleason score 8–10) in terms of BM prevention in the ZEUS trial. After a median follow-up of 4.8 years 14.7% of the PC patients in the ZA group and only 13.2% of PC patients in the control group developed BM (log-rank: p=0.65). Herby ZA could not hamper tumor spread to bone in high-risk PC patients (Table 1).
Severe adverse effects of intravenously administered ZA are uncommon, however typically self-limiting acute phase reaction including pyrexia and associated symptoms occure in 10% to 30% of patients. Other side effects such as hypocalcemia and others have to be taken into account too [50]. A more frequent severe complication linked to the administration of ZA is ONJ which occurs in approximately 2% of the patients on a monthly ZA regime. Reduction of administration frequency to a 12 weekly application regimen of ZA resulted in a decreased ONJ-incidence by 1% at equal SRE prevention outcome after 2 years [51]. Of note, ZA showed direct anticancer effect in in vitro experiments on hormone refractory PC cell lines and showed synergistic cytotoxic effects with other agents such as docetaxel in further experiments [5253]. These findings have been translated into the STAMPEDE trial were results showed no evidence of efficacy of ZA with regard to SREs and overall survival and herby could not validate the in vitro findings of direct anticancer effect [47].
For several years ZA displayed the standard of care for CRPC patients regarding SRE prevention until Fizazi et al [54] showed the superior performance of denosumab with regards to SRE prevention (Table 1). Denosumab, a fully humanized immunoglobulin G2-anti-RANKL antibody, was evaluated in 1,904 CRPC patients compared to standard of care ZA regarding time to first on study SRE. Administration of 120 mg denosumab subcutaneously was performed every 4 weeks. RANKL inhibition by denosumab prolonged time to first on study SRE by almost 4 months compared to intravenously administered ZA (20.7 months versus 17.1 months; HR, 0.82). In a subgroup analysis focusing on symptomatic skeletal events (SSEs), denosumab also prolonged time to first and subsequent SSEs [4655]. Furthermore, denosumab prevented pain worsening and delayed use of potent analgetics [56]. Severe adverse events of denosumab are in general comparable to those of ZA. mCRPC patients developed ONJ in 2.3% in the denosumab group and 1.3% in the ZA group in a pivotal trial [4657]. Hypocalcemia mostly occurred in the first month of denosmumab administration, therefore supplementation of calcium and vitamin D is recommended [4658].
As RANK/RANKL/OPG pathway is likely to be involved in tumor progression an development of metastases by initiation of EMT, the preventive effect of densomuab in terms of metastasis development was investigated in a multicenter Phase III trial in 1,432 CRPC patients [5759]. A total of 716 non-metastatic CRPC (nmCRPC) patients at high risk for tumor spread to bone (PSA ≥8.0 ng/mL and/or PSA doubling time ≤10.0 months) received monthly subcutaneous densomub 120 mg and showed significantly prolonged bone metastasis free survival by 4.2 months compared to the placebo group (29.5 months versus 25.2 months; HR, 0.85; p=0.028). Of note, overall survival was similar in both groups. Both groups showed generally similar rates of adverse events and severe adverse events with the exception of ONJ. With a 5% rate of ONJ in the densumab group compared to no case in placebo group Food and Drug Administration (FDA) rejected denosumab for metastasis prevention presumably due to risk-benefit profile [57].
Another bone targeting agent with a completely different mechanism of action has been approved for the treatment of mCRPC in 2013. Intravenously administered radium-223-dichloride shows calcium mimetic features and therefore binds to hydroxyapatite especially in sites with increased bone turnover, locally emitting alpha radiation and subsequently leading to double-strand DNA breaks with potent local cytotoxic effect [4660]. The placebo-controlled phase III ALSYMPCA trial evaluated the efficacy of 6 applications intravenously administered radium-223-dichloride or placebo in bone metastatic PC patients after docetaxel or in PC patients unfit for docetaxel. Irrespective of taxane-based chemotherapy pretreatment, radium-223-dichloride improved overall survival by nearly 3 months compared to placebo (14.0 months versus 11.2 months; HR, 0.70). Moreover, radium-223-dichloride statistically delayed first and concomitant on-study SREs with a median occurrence at 15.6 and 9.8 months, respectively (p<0.001), and also improved time to first SSE [4660] (Table 1). Of note, adverse events occurred in comparable incidence in intervention and control group with anemia, nausea, fatigue, weight-loss, and edema [6061]. In a subgroup analysis of the ALSYMPCA trial, against all concern, combination with antiresorptive agents, such as ZA or denosumab did not result in an impaired efficacy of radium-223-dichloride. In contrast, patients receiving antiresortives in addition to radium-223-dichloride showed prolonged time to first SSE [62]. In a phase 1/2 trial (NCT01106352) the combination of radium-223-dichloride with low dose docetaxel lead to a greater percent decline in total alkaline phosphatase and in formation markers such as procollagen type 1 N propeptide and bone-specific alkaline phosphatase. This effect needs to be evaluated in a larger cohort and needs to be correlated to clinical data [63]. A progressive disease after at least two previous treatments for metastatic PC displays the prerequisite for the use of radium-223-dichloride since a study on metastatic PC patients with mild symptoms showed increased fracture risk and mortality in combination with abiraterone [64]. Exclusion criteria for the use of radium-223-dichloride in PC patients are visceral metastasis [46]. The combination of radium-223-dichloride and enzalutamide is currently evaluated in a clinical trial (NCT02194842). Due to an increased fracture risk (26.0% versus 8.1%) and mortality (34.4% versus 28.2%) for the combination of radium-223-dichloride and abiraterone, combination therapy of radium-223-dichloride with other systemically acting agents besides ADT is currently not recommended by manufacturer [6164].
Systemically acting agents in use for the treatment of metastatic PC are cytotoxic taxanes such as docetaxel and cabacitaxel as well as pharmaceuticals influencing the androgen pathway, such as abiraterone and enzalutamide.
Docetaxel has been approved for the treatment of patients with mCRPC and herby prolonged overall survival compared to mitoxantrone. In progressive post docetaxel metastatic PC patients cabacitaxel has been approved as second-line chemotherapy showing again prolonged overall survival and reduced risk of death compared to mitoxantrone. Evidence on beneficial effects on bone health are rare due to the lack of end points concerning SREs or SSEs in most trials so that no evidence based conclusion could be drawn from the existing data [9616566].
In 2011 abiraterone acetate have been approved for the treatment of mCRPC after taxane based chemotherapy. Abiraterone acetate selectively inhibits androgen biosynthesis by potently and irreversibly blocking CYP17 and herby influences testosterone and estrogen biosynthesis. In the COU-AA-301 trial, which led to the approval of abiraterone acetate, 797 mCRPC patients were randomly assigned to receive either orally administered abiraterone acetate or placebo plus prednisone. Abiraterone acetate not only significantly increased overall survival (15.8 months versus 11.2 months; p<0.0001) but also prolonged time to first SRE (9.9 months versus 4.9 months; p=0.0001) and showed beneficial effect on pain levels compared to baseline [676869]. A second Phase III study (COU-AA-302) has led to the expanded approval of abiraterone acetate for the treatment of chemotherapy-naïve mCRPC patients by enhanced performance of abiraterone acetate over prednisone alone with respect to overall survival (34.7 months versus 30.3 months; HR, 0.81; p=0.0033), radiographic progression-free survival (16.5 months versus 8.3 months; HR, 0.53; p<0.001), time to opiate use for cancer-related pain and longer time to decline in performance status [467071] (Table 1).
In 2018 received approval of abiraterone acetate in metastatic high-risk castration sensitive PC based on the results of the LATITUDE trial. In this trial 1,199 patients were randomly assigned to abiraterone acetate plus prednisone or placebo. Median overall survival was not achieved in the abiraterone acetate arm and 34.7 months and placebos arms, respectively (HR, 0.621; p<0.0001). Time to next SRE was not reached in intervention and control arm after a median follow-up of 30.4 months [72]. Typical adverse events in patients receiving abiraterone acetate that occured in at least 5% in the LATITUDE trial are hypertension, hot flush, hypokalemia, increased alanine aminotransferase or aspartate aminotransferase, headache, urinary tract infection, upper respiratory tract infection, and cough [72]. The STAMPEDE trial investigates the potential benefit of the addition of abiraterone acetate to ADT in newly diagnosed and metastatic, node-positive or high-risk locally advanced PC patients or in relapsing PC patients after radical surgery or radiotherapy with high risk features. After 3 years of follow-up the addition of abiraterone acetate to ADT caused not only a beneficial effect on survival rate (83% versus 76%; HR, 0.63; p<0.001) in the combination arm but also dramatically reduced the risk of an SRE (12% versus 22%; HR, 0.46; p<0.001). The spectrum of adverse events are mostly comparable to those in the LATITUDE trial [73].
The second-generation nonsteroidal antiandrogen enzalutamide had been approved for the treatment of mCRPC in 2012. Enzalutamide competitively binds the AR, inhibits AR translocation to cell nucleus and herby suppresses AR signaling [46]. The approval of enzalutamide was based on the results of the AFFIRM trial where mCRPC patients after treatment-failure of docetaxel were included to receive enzalutamide orally once a day. This trial was stopped after an interim analysis showing significantly superior overall survival in the enzalutamide group compared to the placebo group (18.4 months versus 13.6 months; HR, 0.63; p<0.001). Of note, enzalutamide significantly prolonged time to the first skeletal-related event (16.7 months versus 13.3 months; HR, 0.69; p<0.001) in mCRPC patients [74]. The PREVAIL trial investigated the effect of enzalutamide in metastatic PC patients before chemotherapy. Enzalutamide significantly reduced the risk of death by 29% in metastatic PC patients before docetaxel (HR, 0.71; p<0.001) and prolonged time to first SRE [75]. Most recently, enzalutamide has been approved for patients with nmCRPC and high risk of developing metastases based on the results of the PROSPER trial. In this trial enzalutamide showed statistically significant improvement of metastasis-free survival compared to placebo (36.6 months versus 14.7 months; HR, 0.29; p<0.001) (Table 1). Most common adverse events in the enzalutamide group of this trial that occurred more frequent than in the placebo group were asthenia or fatigue, hypertension, nausea, hot flush, dizziness, and falls [76].
In the double-blind, placebo-controlled, phase 3 SPARTAN trial involving men with nmCRPC at high risk (PSA doubling time ≤10 months) apalutamide, a AR inhibitor, showed dramatic effect on median metastasis-free survival (40.5 months versus 16.2 months in the placebo group (HR for metastasis or death, 0.28; p<0.001). Adverse events leading to discontinuation of the trial regimen occurred more frequent in the apalutamide group (10.6% versus 7.05%), especially rash (23.8% versus 5.5%), hypothyroidism (8.1% versus 2.0%) and fracture (11.7% versus 6.5%) occurred more often under apalutamide treatment [77]. FDA approved apalutamide for patients with nmCRPC in 2018 while the approval of the European Medicines Agency has been granted in early 2019.
Recently, darolutamide, a structurally unique AR antagonist, showed significantly improved metastasis-free survival (40.4 months versus 18.4 months; HR, 0.41; p<0.001), overall survival, time to SSE and time to pain progression in nmCRPC patients with a PSA doubling time of 10 months or less in ARAMIS trial. Severe adverse events were similar in darolutamide and placebo group [78]. The rolling submission to the FDA for the approval of darolutamide in nmCRPC has been completed in February 2019.
The results of the PROSPER, SPARTAN, and ARAMIS trials suggest that AR-targeting agents may show stronger effects on prevention of metastases in nmCRPC patients compared to bone targeting agents. To further improve metastases prevention in PC, investigations on combination therapy of these systemically acting agents and bone targeting agents in prospective, radomized trials are of high interest. Both in SPARTAN and prosper approximately 10% of patients received bone-targeting agents. This limits the conclusions that can be made based on these trials regarding the potential synergisms of AR-targeting agents and bone targeting agents for prevention of metastases formation.
Besides bone pain, impending pathological fractures or neurological symptoms e.g., caused by compression of the spinal cord can require external beam radiotherapie (ERBT) of the PC-BMs [4661]. More than 50% to 80% of metastatic PC patients report decreasing pain after palliative ERBT of BMs. Of note, this effect was irrespective of single- or multi-dose ERBT regimen. Therefore, current recommendations suggest single-dose of 8 Gy or multi-dose ERBT of 10 times 3 Gy for pain reduction although multi-dose ERBT seems to reduce the rate of re-radiation of the same site in long survivors and induce a superior remineralization of the bone [6179]. After ERBT, indicated by bone pain, more than 50% of the patients report decreasing pain after 1 to 2 weeks lasting for more than 6 months [618081]. Pathological fractures or spinal cord compression with subsequent acute neurological symptoms caused by BMs of PC often require surgical interventions such as surgical stabilization e.g., by internal fixation [61].
A more recent approach in local control of disease in oligometastatic PC patients displays the metastasis directed therapy (MDT) which suggested an improved progression-free survival in several retrospective investigations [82]. Methods in use for MDT are image-guided single-fraction robotic stereotactic radiosurgery (CyberKnife®) and stereotactic body radiotherapy as well as conventional surgery. A trial comparing CyberKnife® versus stereotactic ablative radiation therapy showed local control rates of 70% in the 51 included PC patients [4683]. In a prospective, multicenter, randomized, phase II trial 62 asymptomatic PC patients suffering biochemical recurrence after primary PC treatment with curative intend and no more than three extracranial metastatic lesions were randomized in surveillance or MDT group. MDT included surgery if possible or stereotactic body radiotherapy. After a median follow-up of three years PC patients after MDT showed distinctly prolonged ADT-free survival (primary endpoint) compared to active surveillance (21 months versus 13 months; HR, 0.60; p=0.11). Of note, MDT- and surveillance-arm showed equal QoL and adverse events [82]. MDT in oligometastatic PC patients seems to be promising, although further investigation defining the optimal patients' characteristics are needed in advance of implementation in guidelines and daily clinical use.
Bone health in PC patient is crucial for an acceptable QoL and for the optimal outcome for the patient. Therefore, several pharmaceuticals targeting bone metabolism and androgen pathway had been introduced especially in advanced stage PC patients. Emphasis on the introduction of denosumab in combination with calcium and vitamin D in metastatic PC patients with bone-involvement is given to reduce CTIBL and subsequent SREs. Beneficial effects of systemically acting agents such as abiraterone acetate and enzalutamide with regard to overall survival and extended time to first SRE were observed in CRPC patients and support the use of these agents for treatment of BM.
Notes
Disclosure: AS served as advisor for Ipsen Pharma, Janssen, Alere, Bristol-Myers Squibb, Stebabiotech, Synergo and Ferring. TT served in advisory boards of Amgen and Bayer. AS and TT received speakers' bureau honoraria of Astellas and Janssen; AS of Ipsen Pharma, Sanofi Aventis and Curevac and TT of Amgen. Other authors have no potential conflicts of interest to disclose.
References
1. Gandaglia G, Karakiewicz PI, Briganti A, Passoni NM, Schiffmann J, Trudeau V, et al. Impact of the site of metastases on survival in patients with metastatic prostate cancer. Eur Urol. 2015; 68:325–334. PMID: 25108577.


2. Bubendorf L, Schöpfer A, Wagner U, Sauter G, Moch H, Willi N, et al. Metastatic patterns of prostate cancer: an autopsy study of 1,589 patients. Hum Pathol. 2000; 31:578–583. PMID: 10836297.


3. Ibrahim A, Scher N, Williams G, Sridhara R, Li N, Chen G, et al. Approval summary for zoledronic acid for treatment of multiple myeloma and cancer bone metastases. Clin Cancer Res. 2003; 9:2394–2399. PMID: 12855610.
4. Howard LE, De Hoedt AM, Aronson WJ, Kane CJ, Amling CL, Cooperberg MR, et al. Do skeletal-related events predict overall survival in men with metastatic castration-resistant prostate cancer? Prostate Cancer Prostatic Dis. 2016; 19:380–384. PMID: 27377207.


5. Krupski TL, Foley KA, Baser O, Long S, Macarios D, Litwin MS. Health care cost associated with prostate cancer, androgen deprivation therapy and bone complications. J Urol. 2007; 178:1423–1428. PMID: 17706711.


6. Weinfurt KP, Li Y, Castel LD, Saad F, Timbie JW, Glendenning GA, et al. The significance of skeletal-related events for the health-related quality of life of patients with metastatic prostate cancer. Ann Oncol. 2005; 16:579–584. PMID: 15734776.


7. Heidenreich A, Bastian PJ, Bellmunt J, Bolla M, Joniau S, van der Kwast T, et al. EAU guidelines on prostate cancer. Part II: treatment of advanced, relapsing, and castration-resistant prostate cancer. Eur Urol. 2014; 65:467–479. PMID: 24321502.


8. Taxel P, Faircloth E, Idrees S, Van Poznak C. Cancer treatment-induced bone loss in women with breast cancer and men with prostate cancer. J Endocr Soc. 2018; 2:574–588. PMID: 29942922.


9. de Bono JS, Oudard S, Ozguroglu M, Hansen S, Machiels JP, Kocak I, et al. Prednisone plus cabazitaxel or mitoxantrone for metastatic castration-resistant prostate cancer progressing after docetaxel treatment: a randomised open-label trial. Lancet. 2010; 376:1147–1154. PMID: 20888992.


10. Tannock IF, de Wit R, Berry WR, Horti J, Pluzanska A, Chi KN, et al. Docetaxel plus prednisone or mitoxantrone plus prednisone for advanced prostate cancer. N Engl J Med. 2004; 351:1502–1512. PMID: 15470213.


11. Quach JM, Askmyr M, Jovic T, Baker EK, Walsh NC, Harrison SJ, et al. Myelosuppressive therapies significantly increase pro-inflammatory cytokines and directly cause bone loss. J Bone Miner Res. 2015; 30:886–897. PMID: 25418357.


12. Wissing MD. Chemotherapy- and irradiation-induced bone loss in adults with solid tumors. Curr Osteoporos Rep. 2015; 13:140–145. PMID: 25712619.


13. Msaouel P, Diamanti E, Tzanela M, Koutsilieris M. Luteinising hormone-releasing hormone antagonists in prostate cancer therapy. Expert Opin Emerg Drugs. 2007; 12:285–299. PMID: 17604502.


14. Hofbauer LC, Rachner TD, Coleman RE, Jakob F. Endocrine aspects of bone metastases. Lancet Diabetes Endocrinol. 2014; 2:500–512. PMID: 24880565.


15. Todenhöfer T, Stenzl A, Hofbauer LC, Rachner TD. Targeting bone metabolism in patients with advanced prostate cancer: current options and controversies. Int J Endocrinol. 2015; 2015:838202. PMID: 25802521.


16. Grossmann M, Cheung AS, Zajac JD. Androgens and prostate cancer; pathogenesis and deprivation therapy. Best Pract Res Clin Endocrinol Metab. 2013; 27:603–616. PMID: 24054933.


17. Drake MT, Khosla S. Male osteoporosis. Endocrinol Metab Clin North Am. 2012; 41:629–641. PMID: 22877433.


18. Briot K, Roux C. Glucocorticoid-induced osteoporosis. RMD Open. 2015; 1:e000014. PMID: 26509049.


19. Canalis E, Mazziotti G, Giustina A, Bilezikian JP. Glucocorticoid-induced osteoporosis: pathophysiology and therapy. Osteoporos Int. 2007; 18:1319–1328. PMID: 17566815.


20. Cheung AS, Zajac JD, Grossmann M. Muscle and bone effects of androgen deprivation therapy: current and emerging therapies. Endocr Relat Cancer. 2014; 21:R371–R394. PMID: 25056176.


21. Saad F, Adachi JD, Brown JP, Canning LA, Gelmon KA, Josse RG, et al. Cancer treatment-induced bone loss in breast and prostate cancer. J Clin Oncol. 2008; 26:5465–5476. PMID: 18955443.


23. Wilson KM, Shui IM, Mucci LA, Giovannucci E. Calcium and phosphorus intake and prostate cancer risk: a 24-y follow-up study. Am J Clin Nutr. 2015; 101:173–183. PMID: 25527761.


24. Smith MR, Egerdie B, Hernández Toriz N, Feldman R, Tammela TL, Saad F, et al. Denosumab in men receiving androgen-deprivation therapy for prostate cancer. N Engl J Med. 2009; 361:745–755. PMID: 19671656.


25. Serpa Neto A, Tobias-Machado M, Esteves MA, Senra MD, Wroclawski ML, Fonseca FL, et al. Bisphosphonate therapy in patients under androgen deprivation therapy for prostate cancer: a systematic review and meta-analysis. Prostate Cancer Prostatic Dis. 2012; 15:36–44. PMID: 21894175.


26. Smith MR, Morton RA, Barnette KG, Sieber PR, Malkowicz SB, Rodriguez D, et al. Toremifene to reduce fracture risk in men receiving androgen deprivation therapy for prostate cancer. J Urol. 2010; 184:1316–1321. PMID: 20723926.


27. Rachner TD, Göbel A, Benad-Mehner P, Hofbauer LC, Rauner M. Dickkopf-1 as a mediator and novel target in malignant bone disease. Cancer Lett. 2014; 346:172–177. PMID: 24462802.


28. García-Fontana B, Morales-Santana S, Varsavsky M, García-Martín A, García-Salcedo JA, Reyes-García R, et al. Sclerostin serum levels in prostate cancer patients and their relationship with sex steroids. Osteoporos Int. 2014; 25:645–651. PMID: 23903956.


29. Cosman F, Crittenden DB, Adachi JD, Binkley N, Czerwinski E, Ferrari S, et al. Romosozumab treatment in postmenopausal women with osteoporosis. N Engl J Med. 2016; 375:1532–1543. PMID: 27641143.


30. Lewiecki EM, Blicharski T, Goemaere S, Lippuner K, Meisner PD, Miller PD, et al. A phase III randomized placebo-controlled trial to evaluate efficacy and safety of romosozumab in men with osteoporosis. J Clin Endocrinol Metab. 2018; 103:3183–3193. PMID: 29931216.


31. Giles RH, van Es JH, Clevers H. Caught up in a Wnt storm: Wnt signaling in cancer. Biochim Biophys Acta. 2003; 1653:1–24. PMID: 12781368.


32. Gomes RR Jr, Buttke P, Paul EM, Sikes RA. Osteosclerotic prostate cancer metastasis to murine bone are enhanced with increased bone formation. Clin Exp Metastasis. 2009; 26:641–651. PMID: 19421879.


33. Fizazi K, Massard C, Smith M, Rader M, Brown J, Milecki P, et al. Bone-related parameters are the main prognostic factors for overall survival in men with bone metastases from castration-resistant prostate cancer. Eur Urol. 2015; 68:42–50. PMID: 25449207.


34. Hahn NM, Yiannoutsos CT, Kirkpatrick K, Sharma J, Sweeney CJ. Failure to suppress markers of bone turnover on first-line hormone therapy for metastatic prostate cancer is associated with shorter time to skeletal-related event. Clin Genitourin Cancer. 2014; 12:33–40.e4. PMID: 24126237.


35. Oster G, Lamerato L, Glass AG, Richert-Boe KE, Lopez A, Chung K, et al. Natural history of skeletal-related events in patients with breast, lung, or prostate cancer and metastases to bone: a 15-year study in two large US health systems. Support Care Cancer. 2013; 21:3279–3286. PMID: 23884473.


36. Berish RB, Ali AN, Telmer PG, Ronald JA, Leong HS. Translational models of prostate cancer bone metastasis. Nat Rev Urol. 2018; 15:403–421. PMID: 29769644.


37. Nagle RB, Cress AE. Metastasis update: human prostate carcinoma invasion via tubulogenesis. Prostate Cancer. 2011; 2011:249290. PMID: 21949592.


38. Smith BN, Odero-Marah VA. The role of snail in prostate cancer. Cell Adh Migr. 2012; 6:433–441. PMID: 23076049.


39. Taichman RS, Cooper C, Keller ET, Pienta KJ, Taichman NS, McCauley LK. Use of the stromal cell-derived factor-1/CXCR4 pathway in prostate cancer metastasis to bone. Cancer Res. 2002; 62:1832–1837. PMID: 11912162.
40. Engl T, Relja B, Marian D, Blumenberg C, Müller I, Beecken WD, et al. CXCR4 chemokine receptor mediates prostate tumor cell adhesion through alpha5 and beta3 integrins. Neoplasia. 2006; 8:290–301. PMID: 16756721.
41. McCabe NP, De S, Vasanji A, Brainard J, Byzova TV. Prostate cancer specific integrin alphavbeta3 modulates bone metastatic growth and tissue remodeling. Oncogene. 2007; 26:6238–6243. PMID: 17369840.
42. Hall CL, Daignault SD, Shah RB, Pienta KJ, Keller ET. Dickkopf-1 expression increases early in prostate cancer development and decreases during progression from primary tumor to metastasis. Prostate. 2008; 68:1396–1404. PMID: 18561248.


43. Saad F, Gleason DM, Murray R, Tchekmedyian S, Venner P, Lacombe L, et al. Long-term efficacy of zoledronic acid for the prevention of skeletal complications in patients with metastatic hormone-refractory prostate cancer. J Natl Cancer Inst. 2004; 96:879–882. PMID: 15173273.


44. Prentice A. Diet, nutrition and the prevention of osteoporosis. Public Health Nutr. 2004; 7:227–243. PMID: 14972062.


45. Saad F, Gleason DM, Murray R, Tchekmedyian S, Venner P, Lacombe L, et al. A randomized, placebo-controlled trial of zoledronic acid in patients with hormone-refractory metastatic prostate carcinoma. J Natl Cancer Inst. 2002; 94:1458–1468. PMID: 12359855.


46. Hegemann M, Maas M, Rausch S, Walz S, Bedke J, Stenzl A, et al. Current concepts and trends in the treatment of bone metastases in patients with advanced prostate cancer. Asian J Androl. 2019; 21:12–18.


47. James ND, Sydes MR, Clarke NW, Mason MD, Dearnaley DP, Spears MR, et al. STAMPEDE investigators. Addition of docetaxel, zoledronic acid, or both to first-line long-term hormone therapy in prostate cancer (STAMPEDE): survival results from an adaptive, multiarm, multistage, platform randomised controlled trial. Lancet. 2016; 387:1163–1177. PMID: 26719232.
48. Smith MR, Halabi S, Ryan CJ, Hussain A, Vogelzang N, Stadler W, et al. Randomized controlled trial of early zoledronic acid in men with castration-sensitive prostate cancer and bone metastases: results of CALGB 90202 (alliance). J Clin Oncol. 2014; 32:1143–1150. PMID: 24590644.


49. Wirth M, Tammela T, Cicalese V, Gomez Veiga F, Delaere K, Miller K, et al. Prevention of bone metastases in patients with high-risk nonmetastatic prostate cancer treated with zoledronic acid: efficacy and safety results of the Zometa European Study (ZEUS). Eur Urol. 2015; 67:482–491. PMID: 24630685.


50. Serefoglu EC, Tandogdu Z. Efficacy and safety of zoledronic acid in the treatment of glucocorticoid-induced osteoporosis. Ther Clin Risk Manag. 2010; 6:219–223. PMID: 20526439.


51. Himelstein AL, Foster JC, Khatcheressian JL, Roberts JD, Seisler DK, Novotny PJ, et al. Effect of longer-interval vs standard dosing of zoledronic acid on skeletal events in patients with bone metastases: a randomized clinical trial. JAMA. 2017; 317:48–58. PMID: 28030702.
52. Cirak Y, Varol U, Atmaca H, Kisim A, Sezgin C, Karabulut B, et al. Zoledronic acid in combination with serine/threonine phosphatase inhibitors induces enhanced cytotoxicity and apoptosis in hormone-refractory prostate cancer cell lines by decreasing the activities of PP1 and PP2A. BJU Int. 2012; 110:E1147–E1154. PMID: 22882676.


53. Brubaker KD, Brown LG, Vessella RL, Corey E. Administration of zoledronic acid enhances the effects of docetaxel on growth of prostate cancer in the bone environment. BMC Cancer. 2006; 6:15. PMID: 16417633.


54. Fizazi K, Carducci M, Smith M, Damião R, Brown J, Karsh L, et al. Denosumab versus zoledronic acid for treatment of bone metastases in men with castration-resistant prostate cancer: a randomised, double-blind study. Lancet. 2011; 377:813–822. PMID: 21353695.


55. Smith MR, Coleman RE, Klotz L, Pittman K, Milecki P, Ng S, et al. Denosumab for the prevention of skeletal complications in metastatic castration-resistant prostate cancer: comparison of skeletal-related events and symptomatic skeletal events. Ann Oncol. 2015; 26:368–374. PMID: 25425475.


56. Patrick DL, Cleeland CS, von Moos R, Fallowfield L, Wei R, Öhrling K, et al. Pain outcomes in patients with bone metastases from advanced cancer: assessment and management with bone-targeting agents. Support Care Cancer. 2015; 23:1157–1168. PMID: 25533578.


57. Smith MR, Saad F, Coleman R, Shore N, Fizazi K, Tombal B, et al. Denosumab and bone-metastasis-free survival in men with castration-resistant prostate cancer: results of a phase 3, randomised, placebo-controlled trial. Lancet. 2012; 379:39–46. PMID: 22093187.


58. Body JJ, von Moos R, Niepel D, Tombal B. Hypocalcaemia in patients with prostate cancer treated with a bisphosphonate or denosumab: prevention supports treatment completion. BMC Urol. 2018; 18:81. PMID: 30236112.


59. Palafox M, Ferrer I, Pellegrini P, Vila S, Hernandez-Ortega S, Urruticoechea A, et al. RANK induces epithelial-mesenchymal transition and stemness in human mammary epithelial cells and promotes tumorigenesis and metastasis. Cancer Res. 2012; 72:2879–2888. PMID: 22496457.


60. Parker C, Nilsson S, Heinrich D, Helle SI, O'Sullivan JM, Fosså SD, et al. Alpha emitter radium-223 and survival in metastatic prostate cancer. N Engl J Med. 2013; 369:213–223. PMID: 23863050.


61. Walz S, Stenzl A, Todenhöfer T. Management skelettaler und nicht-skelettaler komplikationen beim prostatakarzinom. J Onkol. 2018; 11/2018:22–28.
62. Coleman R, Fossa SD, Chodacki A, Wedel S, Bruland O, Staudacher K, et al. Time to first skeletal-related event (SRE) with radium-223 dichloride (Ra-223) in patients with castration-resistant prostate cancer (CRPC) and bone metastases: ALSYMPCA trial stratification factors analysis. Paper presented at: European Cancer Congress. 2013 Sep 27-Oct 1; Amsterdam, Netherlands. 2013. abstract #2876.
63. Morris MJ, Loriot Y, Fizazi K, Sweeney C, Ryan CJ, Shevrin DH, et al. Effects of radium-223 (Ra-223) with docetaxel versus docetaxel alone on bone biomarkers in patients with bone-metastatic castration-resistant prostate cancer (CRPC): a phase I/IIa clinical trial. J Clin Oncol. 2017; 6(suppl):154.


64. Gourd E. EMA guidance on radium-223 dichloride in prostate cancer. Lancet Oncol. 2018; 19:e190. PMID: 29551361.


65. Berthold DR, Pond GR, Soban F, de Wit R, Eisenberger M, Tannock IF. Docetaxel plus prednisone or mitoxantrone plus prednisone for advanced prostate cancer: updated survival in the TAX 327 study. J Clin Oncol. 2008; 26:242–245. PMID: 18182665.


66. Petrylak DP, Tangen CM, Hussain MH, Lara PN Jr, Jones JA, Taplin ME, et al. Docetaxel and estramustine compared with mitoxantrone and prednisone for advanced refractory prostate cancer. N Engl J Med. 2004; 351:1513–1520. PMID: 15470214.


67. de Bono JS, Logothetis CJ, Molina A, Fizazi K, North S, Chu L, et al. Abiraterone and increased survival in metastatic prostate cancer. N Engl J Med. 2011; 364:1995–2005. PMID: 21612468.


68. Fizazi K, Scher HI, Molina A, Logothetis CJ, Chi KN, Jones RJ, et al. COU-AA-301 Investigators. Abiraterone acetate for treatment of metastatic castration-resistant prostate cancer: final overall survival analysis of the COU-AA-301 randomised, double-blind, placebo-controlled phase 3 study. Lancet Oncol. 2012; 13:983–992. PMID: 22995653.


69. Logothetis CJ, Basch E, Molina A, Fizazi K, North SA, Chi KN, et al. Effect of abiraterone acetate and prednisone compared with placebo and prednisone on pain control and skeletal-related events in patients with metastatic castration-resistant prostate cancer: exploratory analysis of data from the COU-AA-301 randomised trial. Lancet Oncol. 2012; 13:1210–1217. PMID: 23142059.


70. Ryan CJ, Smith MR, de Bono JS, Molina A, Logothetis CJ, de Souza P, et al. COU-AA-302 Investigators. Abiraterone in metastatic prostate cancer without previous chemotherapy. N Engl J Med. 2013; 368:138–148. PMID: 23228172.


71. Ryan CJ, Smith MR, Fizazi K, Saad F, Mulders PF, Sternberg CN, et al. Abiraterone acetate plus prednisone versus placebo plus prednisone in chemotherapy-naive men with metastatic castration-resistant prostate cancer (COU-AA-302): final overall survival analysis of a randomised, double-blind, placebo-controlled phase 3 study. Lancet Oncol. 2015; 16:152–160. PMID: 25601341.


72. Fizazi K, Tran N, Fein L, Matsubara N, Rodriguez-Antolin A, Alekseev BY, et al. Abiraterone plus prednisone in metastatic, castration-sensitive prostate cancer. N Engl J Med. 2017; 377:352–360. PMID: 28578607.


73. James ND, de Bono JS, Spears MR, Clarke NW, Mason MD, Dearnaley DP, et al. Abiraterone for prostate cancer not previously treated with hormone therapy. N Engl J Med. 2017; 377:338–351. PMID: 28578639.
74. Scher HI, Fizazi K, Saad F, Taplin ME, Sternberg CN, Miller K, et al. Increased survival with enzalutamide in prostate cancer after chemotherapy. N Engl J Med. 2012; 367:1187–1197. PMID: 22894553.


75. Beer TM, Armstrong AJ, Rathkopf DE, Loriot Y, Sternberg CN, Higano CS, et al. Enzalutamide in metastatic prostate cancer before chemotherapy. N Engl J Med. 2014; 371:424–433. PMID: 24881730.


76. Hussain M, Fizazi K, Saad F, Rathenborg P, Shore N, Ferreira U, et al. Enzalutamide in men with nonmetastatic, castration-resistant prostate cancer. N Engl J Med. 2018; 378:2465–2474. PMID: 29949494.


77. Smith MR, Saad F, Chowdhury S, Oudard S, Hadaschik BA, Graff JN, et al. Apalutamide treatment and metastasis-free survival in prostate cancer. N Engl J Med. 2018; 378:1408–1418. PMID: 29420164.


78. Fizazi K, Shore N, Tammela TL, Ulys A, Vjaters E, Polyakov S, et al. Darolutamide in nonmetastatic, castration-resistant prostate cancer. N Engl J Med. 2019; 380:1235–1246. PMID: 30763142.


79. Lutz S, Chow E. A review of recently published radiotherapy treatment guidelines for bone metastases: contrasts or convergence? J Bone Oncol. 2012; 1:18–23. PMID: 26909250.


80. Coleman R, Body JJ, Aapro M, Hadji P, Herrstedt J. ESMO Guidelines Working Group. Bone health in cancer patients: ESMO Clinical Practice Guidelines. Ann Oncol. 2014; 25 Suppl 3:iii124–iii137. PMID: 24782453.


81. Weiss RJ, Forsberg JA, Wedin R. Surgery of skeletal metastases in 306 patients with prostate cancer. Acta Orthop. 2012; 83:74–79. PMID: 22206449.


82. Ost P, Reynders D, Decaestecker K, Fonteyne V, Lumen N, De Bruycker A, et al. Surveillance or metastasis-directed therapy for oligometastatic prostate cancer recurrence: a prospective, randomized, multicenter phase II trial. J Clin Oncol. 2018; 36:446–453. PMID: 29240541.


83. Napieralska A, Miszczyk L, Stapor-Fudzinska M. CyberKnife stereotactic radiosurgery and stereotactic ablative radiation therapy of patients with prostate cancer bone metastases. Neoplasma. 2016; 63:304–312. PMID: 26774153.


Fig. 1
Cancer treatment induced bone loss management in prostate cancer (PC). PC patients receiving androgen deprivation therapy (ADT) or cytotoxic agents should be monitored according to the flowchart mentioned above after initial evaluation. BMI: body mass index, BMD: bone mineral density, CRPC: castration resistant prostate cancer. Modified from article of Coleman et al (Ann Oncol 2014;25 Suppl 3:iii124-37) [80] with original copyright holder's permission.
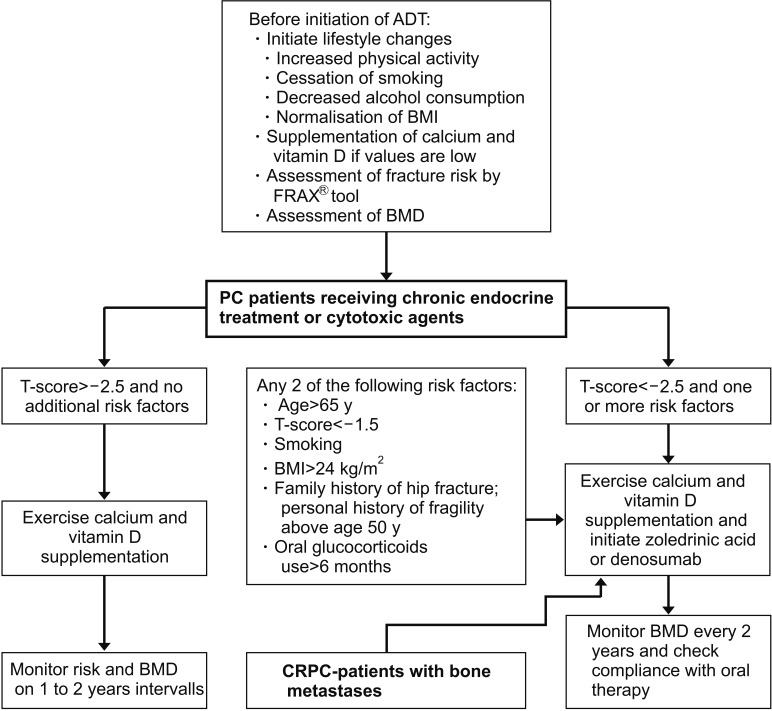
Table 1
Agents in PC treatment and their effect on time to next SRE
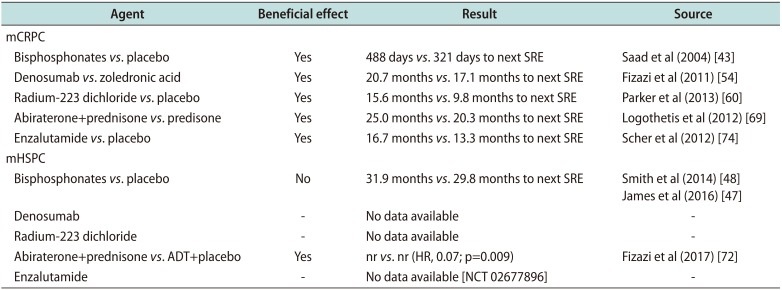
Agent | Beneficial effect | Result | Source |
---|---|---|---|
mCRPC | |||
Bisphosphonates vs. placebo | Yes | 488 days vs. 321 days to next SRE | Saad et al (2004) [43] |
Denosumab vs. zoledronic acid | Yes | 20.7 months vs. 17.1 months to next SRE | Fizazi et al (2011) [54] |
Radium-223 dichloride vs. placebo | Yes | 15.6 months vs. 9.8 months to next SRE | Parker et al (2013) [60] |
Abiraterone+prednisone vs. predisone | Yes | 25.0 months vs. 20.3 months to next SRE | Logothetis et al (2012) [69] |
Enzalutamide vs. placebo | Yes | 16.7 months vs. 13.3 months to next SRE | Scher et al (2012) [74] |
mHSPC | |||
Bisphosphonates vs. placebo | No | 31.9 months vs. 29.8 months to next SRE | Smith et al (2014) [48] |
James et al (2016) [47] | |||
Denosumab | - | No data available | - |
Radium-223 dichloride | - | No data available | - |
Abiraterone+prednisone vs. ADT+placebo | Yes | nr vs. nr (HR, 0.07; p=0.009) | Fizazi et al (2017) [72] |
Enzalutamide | - | No data available [NCT 02677896] | - |