Abstract
Topical anesthetics are commonly used in oral & maxillofacial surgery to control pain in the oral cavity mucosa before local anesthetic injection. These anesthetic agents come in many forms, developed for different usages, to minimize adverse reactions, and for optimal anesthetic efficiency. Earlier studies have revealed that these agents may also limit the growth of microorganisms in the area of anesthetic application. Many topical anesthetic agents show different levels of antimicrobial activity against various bacterial strains and Candida. The dosage of local anesthetic agent used in some clinical preparations is too low to show a significant effect on microbial activity. Efficiency of antimicrobial activity depends on the local anesthetic agent's properties of diffusion within the bloodstream and binding efficiency with cytoplasmic membrane, which is followed by disruption of the bacterial cell membrane. The antimicrobial properties of these agents may extend their usage in patients to both control pain and infection. To develop the topical local anesthetic optimal usage and antimicrobial effect, a collaborating antiseptic agent may be used to benefit the local anesthetic. However, more research is required regarding minimum inhibitory concentration (MIC) and minimum bactericidal concentration (MBC) of topical local anesthetic agents with drug interaction between anesthetics and antiseptic agents.
Local anesthesia is applied topically or administered by injection to control pain in the body. Unlike general anesthesia, local anesthetics do not cause loss of consciousness. Topical anesthetics are used to numb mucosa of the nose, ear and throat, anus, and genital area. It is used adjunctively to relieve pain and to help patients feel more comfortable before surgery. In addition to their anesthetic properties, local anesthetics also possess antimicrobial effects. They have been shown to inhibit and kill a wide spectrum of bacteria and fungus [1]. In dentistry, topical anesthetics are typically used to anesthetize the mucosal surface area of the oral cavity prior to local anesthetic injection [2].
When administering local dental anesthesia, bacteria in the normal oral flora can pass into the tissue by needle injection through the mucous membrane. These bacteria can cause suppurated local infections or odontogenic bacteremia after dental interventions [345]. Therefore, it is possible that the antimicrobial properties of topical anesthetics may provide an additional benefit as an antiseptic to prevent post-operative infections.
We reviewed the effect of local anesthetics on antimicrobial activity with a focus on dentistry. These anesthetics were tested against a broad spectrum of microorganisms, including oral and skin microflora, opportunistic pathogenic bacteria, and fungi. The collective results of in vitro and in vivo studies conducted over many years have substantiated a supplemental role for local anesthetics in the probable prevention and treatment of interventional site infections.
The structure of an anesthetic molecule consists of three components: 1) the lipophilic aromatic ring, 2) an intermediate ester or amide linkage, and 3) the tertiary amine, which influences the clinical properties. The potency of a local anesthetic depends on lipid solubility of the aromatic rings and its substitute compound along with the tertiary amine [67]. For example, bupivacaine is more potent than articaine due to its greater lipid solubility. Lipid solubility also affects the onset of the anesthetic as it enables rapid diffusion of the drug through cell membranes. Thus, the potency of 0.5% bupivacaine is comparable to 4% articaine [8]. Conversely, concentration or the presence of other factors may have a greater effect on onset [7]. Epinephrine in the solution of the local anesthetic decreased its vascular absorption rate, thereby enhancing the deep numbness and long duration of local action [8].
Local anesthetics can be classified as ester-type or amide-type based on its intermediate chain. Drug metabolism is different for each type; esters are hydrolyzed in blood plasma by plasma esterases, whereas amides are metabolized in the liver. The incidence of allergic reaction, from skin rash to anaphylaxis, is highly associated with ester anesthetics due to the formation of p-aminobenzoic acid (PABA) [6], which may cross-react with similar compounds such as sulfa antibiotics or metabisulfite [9].
Cocaine was the first local anesthetic drug, introduced by Koller in 1884 [10]. For oral use, cocaine is available in liquid form mixed with adrenaline for topical anesthesia. It has a reported high risk of addiction and toxicity.
Topical benzocaine is an ester-type anesthetic agent composed of p-aminobenzoic acid (PABA) that can induce allergic reactions. Benzocaine is available in various preparations, such as gel, gel patch, spray, ointment, or solution. Concentrations used range from 6% to 20%, and has multiple uses. Topical use of 20% benzocaine provides anesthetic effect within 30 seconds but requires approximately 2 – 3 minutes to reach adequate depth. It effectively reduces pain caused by injection into the alveolar mucosa and tongue, but only slightly affects the palatal mucosa because of the tissue thickness and dense nerve supply [11]. The duration of action is approximately 5 – 15 minutes after onset. Benzocaine-associated methemoglobinemia has been regularly reported [12].
Tetracaine, also an ester derivative of PABA, is more effective than cocaine [13]. Tetracaine is available in spray and ointment forms of 0.2% to 2.0% concentration. It produces rapid onset within 10 – 20 seconds, with a short duration of action of 10 – 15 minutes. Tetracaine is quickly absorbed into the mucous membrane. Limiting use to 20 mg per session to reduce the risk of adverse reactions has been recommended [14].
Lidocaine is the only amide-type anesthetic that is available in both topical and injectable forms. In dentistry, many forms and concentrations of lidocaine are used, from 2 – 5% gel and solution, 5% ointment, 10% spray, and 20% lidocaine patch [15]. Lidocaine has a potency comparable to benzocaine. It requires three minutes after application to reach adequate anesthesia [16]. Lidocaine is highly effective on alveolar mucous but is less effective on palatal mucous membrane. It is used as an analgesic ingredient in Magic Mouth Wash and mouth rinse products for oral mucositis in patients receiving radiation and chemotherapy [17].
Prilocaine is also used in combination with other topical anesthetics, such as in Eutectic mixture of local anesthetics (EMLA) and Oraqix [18]. The maximum dose is 6 mg/kg in adults and should not exceed 400 mg. Prilocaine has low cardiac toxicity and is relatively safe in pregnant women [19].
EMLA, which contains 2.5% lidocaine and 2.5% prilocaine, is used in procedures or surgeries that cause minimal to considerable pain. EMLA is also commonly used as a topical skin analgesic cream to reduce pain, anxiety, and discomfort associated with venous cannulation in adults and children [20].
Common adverse effects of topical anesthetics are temporary altered sense of taste and allergy. The signs and symptoms of allergic reactions are itching, edema, nasal discharge, shortness of breath, wheezing, and headache [2324].
Allergic reactions are infrequent in amide-type anesthetics [25]. Methemoglobinemia is another adverse reaction caused by anesthetics that can lead to severe or fatal cyanosis and additional adverse effects [12]. Adverse effects from using prilocaine and EMLA creams have been reported [26]. More severe complications can occur, including central nervous system toxicity, cardiovascular toxicity, and methemoglobinemia [2728].
Topical anesthetics eliminate pain by inhibiting signal transmission of tiny nerve endings at the surface [29]. Local anesthetics interrupt neural conduction by inhibiting sodium ion influx through sodium channels within neuronal membranes during their activated and inactivated states. Sensitivity to anesthetics depends on the diameter of neural fibers. Differential sensitivity consequently affects the recovery of neural fibers in first the motor, then the sensory, and finally the autonomic fibers [30].
Various forms of topical anesthetics, such as sprays, solutions, gels, and ointments, and indicated uses have been developed to facilitate clinical use by dentists [213]. The effective duration of a topical anesthetic is 10 – 50 minutes depending on anesthetic usage. When used prior to local anesthetics injection, at least two minutes is required after application for significant anesthetic effect [25].
Numerous previous studies have reported antimicrobial activity of several local anesthetics since their first observations in 1909 (Table 1) [3132333435363738394045464748495051]. The bacteria investigated were all common pathogens. Noda et al. [36] indicated that bupivacaine had greater antibacterial activity than lidocaine. At equal concentrations, greater antibacterial activity was found when preservatives were added to the anesthetics, and the authors observed that while the preservatives alone were weakly bacteriostatic, they were not bactericidal. This points to the significance of bactericidal activity in the anesthetics.
From 1996 to 1998, publications by Sakuragi and colleagues [373839] also indicated that the rate and potency of antimicrobial activity were dose-dependent, time-dependent, and temperature-dependent. They also reported that 0.5% bupivacaine showed the greatest bactericidal activity on methicillin-resistant Staphylococcus aureus.
Aydin et al. [40] showed that a concentration of 1% prilocaine inhibited the growth of Escherichia coli, S. aureus, and Pseudomonas aeruginosa, whereas lidocaine inhibited only P. aeruginosa. While 0.25% bupivacaine was found to inhibit only P. aeruginosa, ropivacaine failed to inhibit the growth of any pathogens [40]. These findings were confirmed by many other studies [41424344]. In vitro, epinephrine appearing in clinical doses of local anesthetic agents has shown no antimicrobial effect. In 1999, Parr et al. [45] demonstrated this in Enterococcus faecalis and vancomycin-resistant enterococci, E. coli, P. aeruginosa, and S. aureus and methicillin-resistant S. aureus. Gram-negative organisms showed the highest sensitivity to lidocaine whereas S. aureus was the least sensitive. Time-kill studies were carried out on bacteria exposed to various clinical concentrations of lidocaine with and without epinephrine. Lidocaine demonstrated a dose-dependent inhibition of growth for all strains of bacteria tested. The addition of epinephrine to the local anesthetic did not affect the susceptibility of bacteria to lidocaine [45]. These results also suggested that lidocaine may have a role in the prophylaxis and treatment of surgical wound infections, particularly in methicillin- and vancomycin-resistant bacterial infections.
A comparison study of antimicrobial effects [46] of 1) articaine, 2) bupivacaine, 3) mepivacaine, 4) prilocaine, 5) lidocaine, 6) butanilicaine, and 7) procaine in combination with four preservatives and vasoconstrictive components showed different minimal inhibitory concentrations (MIC) and minimal bactericidal concentrations (MBC). Antimicrobial activity was proven to come from the anesthetic component, and not from the preservative or vasoconstrictor components. An in vitro study of 25 clinical isolates and ATCC (American Type Culture Collection) reference strains showed powerful antibacterial effects of EMLA [47]. Subsequently, Sedef et al. [48] showed dose-dependent antibacterial activity of EMLA and 5% lidocaine. Recently, Liu et al. [49] reported that 2% lidocaine shows strong antimicrobial activity against eight isolates of commonly encountered bacterial strains in periprosthetic joint infection.
In the case of Candida albicans, Rodrigues et al. [43] suggested that local anesthetics inhibited fungal germ tube formation secondary to a blockade of ionic channels. Pina-Vaz et al. [50] also found fungistatic activity at lower concentrations secondary to yeast metabolic impairment, and fungicidal activity at higher concentrations secondary to cytoplasmic membrane damage. Clinical doses of lidocaine and procaine have demonstrated inhibitory effects on growth of various species of bacteria and fungi.
Sporicidal activity of local anesthetics and their preservatives were tested by Abdelaziz and el-Nakeeb [51]. Inhibition of growth proved to be temperature-dependent for all agents. Higher temperatures were required to elicit sporicidal activity against Bacillus subtilis, compared to Aspergillus niger. Among the preservatives, chlorocresol/local anesthetic combinations also exhibited the highest sporicidal activity [51].
It has been suggested that the inhibitory effect of local anesthetics on bacterial growth results from the disruption of the bacterial cell wall or cytoplasmic membrane, leading to leakage of cellular components and subsequent cell lysis [52]. Table 2 [435053545556] summarizes the results of studies on local anesthetic action and antimicrobial mechanism. It has been reported that penetration of bacterial membranes results from the electrostatic binding of anesthetic molecules to polar groups relating to the hydrophobic character of the anesthetic on the surface of the membrane [54]. Anesthetic molecules inhibit membrane respiratory activity, and change membrane permeability and solubility, causing K+ to be leaked from the bacterial cytoplasm. Including anesthetic agents at high concentrations has shown antifungal activity, and at low concentrations has shown inhibition of germ tube formation in various Candida species [55]. Conversely, there are limited studies that attribute the mechanism of action of antimicrobial activity of local anesthetics.
This paper first reported that isolating and culturing pathogenic microorganisms in samples collected from an area that might be contaminated with the anesthetics can be problematic to the microbiological laboratory [47]. The antibacterial effect of topical anesthetics may lead to false-negative cultures from the specimens. Numerous studies have recommended using a low concentration of anesthetic to decrease the possibility of obtaining false-negative cultures. Table 3 lists the studies on antimicrobial effects in local anesthetics for infection control [31415758596061].
Reynolds et al. [57] found that proparacaine had the lowest MICs, lower than that of povidone-iodine. All tested anesthetics and povidone-iodine inhibited bacterial growth at commercially available concentrations. For the diagnosis of pneumonia, Anding et al. [58] found significant bactericidal activity of the local anesthetic oxybuprocaine against Streptococcus pneumoniae and Haemophilus influenzae, even at the lowest concentration of 0.01%. At 1%, oxybuprocaine inhibited the growth of E. coli and P. aeruginosa. Collectively, growth inhibition by local anesthetics is likely a reason behind low sensitivity observed in microbiological culture. Therefore, it is recommended that clinicians use a low concentration of minimally inhibitory local anesthetics to optimize culture yields.
Antibacterial activity demonstrated over the past several decades indicates that local anesthetics might help eradicate microorganisms that are introduced into the tissues or blood vessels. In the case of methicillin and vancomycin-resistant bacteria, treatment of surgical wound infection has mandated a broader application for local anesthetics [45]. This suggests that the surgical benefit of local anesthesia may extend beyond its analgesic properties, as shown in Table 4 [46626364656667].
Stratford et al. [62] reported that lidocaine produced a 70% reduction in bacterial count in vivo, however adding epinephrine resulted in a 20-fold increase in bacterial count compared to controls. This suggested that hypoxia resulting from vasoconstriction may directly increase the risk of surgical site infection [62]. Lu et al. [63] used a mouse model to demonstrate that infusion of 2% lidocaine in a S. aureus-infected wound showed a nearly 10-fold reduction in bacteria count.
In the process of dental anesthetic injection, oral bacteria can enter the tissue following perforation of the mucous membrane. Dental local anesthesia in compromised patients may, therefore, lead to suppurate local infections or odontogenic bacteremia, as has been shown to occur in other dental surgery interventions. These circumstances have raised the issue of routine antibiotic prophylaxis in oral surgery [6465].
Pelz et al. [46] studied antimicrobial activity of commonly used local anesthetics in dentistry, occurring at the concentrations of the seven commercially available local anesthetics. The tested microorganisms were oral flora, including Streptococcus viridans and anaerobic bacteria, skin and intestinal flora, opportunistic pathogenic bacteria, and C. albicans strains.
The antimicrobial activity of methyl-4-hydroxybenzoate, sodium bisulfite, adrenaline hydrogen tartrate, and adrenaline (preservative and vasoconstrictive components in anesthetics) were tested. Ultracaine D-S and its active substance, articaine hydrochloride, similarly showed the most prominent antimicrobial activity with regards to both MIC and MBC. The routinely applied concentration of Ultracaine D-S and Hostacaine was roughly four-times higher and two-times higher, respectively, than the MBC values for the tested bacteria, whereas MBC values were not reached or exceeded with the concentrations of other anesthetics used. Novocaine showed the lowest antimicrobial activity and did not inhibit 35 species tested. Due to the results obtained with nosocomial strains of E. coli, S. aureus, and P. aeruginosa, disinfection of the mucous membranes should be performed routinely in immunocompromised patients, regardless of the anesthetic used.
A solution of 1:100,000 epinephrine and 2% Xylocaine®, was shown to inhibit the growth of specific organisms and to be bactericidal to others. Its bactericidal activity was significantly less than 2% Xylocaine® without epinephrine.
Therefore, dentists can apply the potential use of local anesthetics as antiseptics in a variety of dental procedures to eliminate bacteria on the surface areas of treatment. During dental treatment, local anesthetic injection techniques, such as buccal infiltration and conventional and modified intraligamental injection, have been found to significantly increase the rate of bacteremia [4]. It was shown that elevated levels of bacteria in the bloodstream that increased immediately after local anesthetic injection returned to normal within one hour [5].
Local anesthetics can inhibit the growth of bacteria that have inoculated into the soft tissue during the injection process. For dental treatments such as tooth extraction and root scaling, antibiotic prophylaxis is recommended prior to the dental procedure to decrease bacteremia rates, especially in immune-compromised patients or patients under immunosuppressive drugs [36667].
Alternatively, infection may occur after dentoalveolar surgery such as third molar removal and dental implant placement [6869]. This can occur from contamination during the surgical process, in suturing, in the environment, as well as from patient hygiene [70]. Prophylaxis antibiotics should be prescribed prior to the operation to reduce the rate of postoperative infection [6871].
For dental implant surgery, the use of chlorhexidine mouth rinse significantly reduces plaque collection and the microorganisms that relate to postoperative infection. Among several antibacterial agents, chlorhexidine is recommended as the gold standard for surgical site infection control due to its broad spectrum and antiseptic efficacy [72].
In patients with oral mucositis who have received radiotherapy, symptoms can arise from acute inflammation of oral mucosa, tongue, and pharynx. Agents are required to reduce pain and prevent infection. In general, the local anesthetics diphenhydramine, xylocaine, and lidocaine are the drugs of choice to relieve the pain, and non-alcohol-containing chlorhexidine is recommended to prevent the infection [7374].
Local anesthetics not only serve as agents for pain control but possess antimicrobial activity as well. Caution should be exercised when administering local anesthetics prior to diagnostic procedures in which culture specimens are to be obtained, as the antimicrobial activity of the local anesthetic could lead to false-negative results. In such cases, it is recommended that the lowest concentration possible of a mildly antimicrobial agent be used to optimize culture yields.
However, local anesthetics can be considered as an adjunct to traditional antimicrobial use in the clinical or laboratory setting. Greater concentration, longer exposure, and higher temperature each correlate with a proportional increase in microbial growth inhibition. Multiple local anesthetics at concentrations typically used in the clinical setting, such as 0.125% – 0.75% bupivacaine and 1% – 3% lidocaine, inhibit the growth of numerous bacteria and fungi. Bupivacaine and lidocaine inhibit growth to a significantly higher degree than ropivacaine.
Minimal information is available on the antimicrobial efficacy of anesthetic agents in dental clinical settings. The rate of onset of antimicrobial activity of local anesthetics as well as whether the activity is bactericidal or bacteriostatic is still unknown. Similarly, antimicrobial activity of local anesthetics with preservatives has not been studied. A statement on the possible prophylaxis use of topical anesthetics in dentistry cannot currently be made unless more clinical studies have confirmed.
ACKNOWLEDGEMENTS
The authors would like to thank the staff and dental assistants, and include our colleagues at the Department of Oral and Maxillofacial Surgery, Faculty of Dentistry, Mahidol University.
References
1. Johnson SM, Saint John BE, Dine AP. Local anesthetics as antimicrobial agents: a review. Surg Infect (Larchmt). 2008; 9:205–213. PMID: 18426354.


2. Ogle OE, Mahjoubi G. Local anesthesia: agents, techniques, and complications. Dent Clin North Am. 2012; 56:133–148. ixPMID: 22117947.


3. Baltch AL, Schaffer C, Hammer MC, Sutphen NT, Smith RP, Conroy J, et al. Bacteremia following dental cleaning in patients with and without penicillin prophylaxis. Am Heart J. 1982; 104:1335–1339. PMID: 7148652.


4. Roberts GJ, Simmons NB, Longhurst P, Hewitt PB. Bacteraemia following local anaesthetic injections in children. Br Dent J. 1998; 185:295–298. PMID: 9803037.


5. Barbosa M, Carmona IT, Amaral B, Limeres J, Alvarez M, Cerqueira C, et al. General anesthesia increases the risk of bacteremia following dental extractions. Oral Surg Oral Med Oral Pathol Oral Radiol Endod. 2010; 110:706–712. PMID: 20580576.


6. Covino BG. Pharmacology of local anaesthetic agents. Br J Anaesth. 1986; 58:701–716. PMID: 2425835.


7. Becker DE, Reed KL. Local anesthetics: review of pharmacological considerations. Anesth Prog. 2012; 59:90–101. PMID: 22822998.


8. Petrikas AZH, Egorova VA, Ermilova KV. Articaine and adrenaline combinations efficacy for dental anesthesia. Stomatologiia (Mosk). 2009; 88:24–26.
9. Eggleston ST, Lush LW. Understanding allergic reactions to local anesthetics. Ann Pharmacother. 1996; 30:851–857. PMID: 8826570.


10. Leonard M. Carl Koller: mankind's greatest benefactor? The story of local anesthesia. J Dent Res. 1998; 77:535–538. PMID: 9539455.


11. Keller BJ. Comparison of the effectiveness of two topical anesthetics and a placebo in reducing injection pain. Hawaii Dent J. 1985; 16:10–11.
12. Cash C, Arnold DH. Extreme Methemoglobinemia After Topical Benzocaine: Recognition by Pulse Oximetry. J Pediatr. 2017; 181:319. PMID: 27881239.


13. Lee HS. Recent advances in topical anesthesia. J Dent Anesth Pain Med. 2016; 16:237–244. PMID: 28879311.


14. Davis KL, Channick CL. Safety of topical tetracaine in patients undergoing flexible bronchoscopy. J Bronchology Interv Pulmonol. 2009; 16:95–98. PMID: 23168506.


15. Bagesund M, Tabrizi P. Lidocaine 20% patch vs lidocaine 5% gel for topical anaesthesia of oral mucosa. Int J Paediatr Dent. 2008; 18:452–460. PMID: 18341564.
16. Roghani S, Duperon DF, Barcohana N. Evaluating the efficacy of commonly used topical anesthetics. Pediatr Dent. 1999; 21:197–200. PMID: 10355012.
17. Kirk LM, Brown SD, Luu Y, Ogle A, Huffman J, Lewis PO. Beyond-use dating of lidocaine alone and in two “magic mouthwash” preparations. Am J Health Syst Pharm. 2017; 74:e202–e210. PMID: 28438825.


18. Mayor-Subirana G, Yague-Garcia J, Valmaseda-Castellon E, Arnabat-Dominguez J, Berini-Aytes L, Gay-Escoda C. Anesthetic efficacy of Oraqix(R) versus Hurricaine(R) and placebo for pain control during non-surgical periodontal treatment. Med Oral Patol Oral Cir Bucal. 2014; 19:e192–e201. PMID: 24316699.
19. Guay J. Adverse events associated with intravenous regional anesthesia (Bier block): a systematic review of complications. J Clin Anesth. 2009; 21:585–594. PMID: 20122591.


20. Speirs AF, Taylor KH, Joanes DN, Girdler NM. A randomised, double-blind, placebo-controlled, comparative study of topical skin analgesics and the anxiety and discomfort associated with venous cannulation. Br Dent J. 2001; 190:444–449. PMID: 11352393.


21. McMillan AS, Walshaw D, Meechan JG. The efficacy of Emla and 5% lignocaine gel for anaesthesia of human gingival mucosa. Br J Oral Maxillofac Surg. 2000; 38:58–61. PMID: 10783450.


22. Munshi AK, Hegde AM, Latha R. Use of EMLA: is it an injection free alternative? J Clin Pediatr Dent. 2001; 25:215–219. PMID: 12049081.


23. Jackson D, Chen AH, Bennett CR. Identifying true lidocaine allergy. J Am Dent Assoc. 1994; 125:1362–1366. PMID: 7844301.
24. Wilson AW, Deacock S, Downie IP, Zaki G. Allergy to local anaesthetic: the importance of thorough investigation. Br Dent J. 2000; 188:120–122. PMID: 10717997.


25. Meechan JG. Effective topical anesthetic agents and techniques. Dent Clin North Am. 2002; 46:759–766. PMID: 12436830.


26. Parker JF, Vats A, Bauer G. EMLA toxicity after application for allergy skin testing. Pediatrics. 2004; 113:410–411. PMID: 14754960.


27. Tran AN, Koo JY. Risk of systemic toxicity with topical lidocaine/prilocaine: a review. J Drugs Dermatol. 2014; 13:1118–1122. PMID: 25226014.
28. Larson A, Stidham T, Banerji S, Kaufman J. Seizures and methemoglobinemia in an infant after excessive EMLA application. Pediatr Emerg Care. 2013; 29:377–379. PMID: 23462397.


29. Strichartz G. Molecular mechanisms of nerve block by local anesthetics. Anesthesiology. 1976; 45:421–441. PMID: 9844.


30. Myers MI, Peltier AC. Uses of skin biopsy for sensory and autonomic nerve assessment. Curr Neurol Neurosci Rep. 2013; 13:323. PMID: 23250768.


31. Schmidt RM, Rosenkranz HS. Antimicrobial activity of local anesthetics: lidocaine and procaine. J Infect Dis. 1970; 121:597–607. PMID: 4393033.


32. James FM, George RH, Naiem H, White GJ. Bacteriologic aspects of epidural analgesia. Anesth Analg. 1976; 55:187–190. PMID: 943977.


33. Rosenberg PH, Renkonen OV. Antimicrobial activity of bupivacaine and morphine. Anesthesiology. 1985; 62:178–179. PMID: 3918482.


34. Hodson M, Gajraj R, Scott NB. A comparison of the antibacterial activity of levobupivacaine vs. bupivacaine: an in vitro study with bacteria implicated in epidural infection. Anaesthesia. 1999; 54:699–702. PMID: 10417467.
35. Grimmond TR, Brownridge P. Antimicrobial activity of bupivacaine and pethidine. Anaesth Intensive Care. 1986; 14:418–420. PMID: 3105346.


36. Noda H, Saionji K, Miyazaki T. Antibacterial activity of local anesthetics. Masui. 1990; 39:994–1001. PMID: 2232143.


37. Sakuragi T, Ishino H, Dan K. Bactericidal activity of clinically used local anesthetics on Staphylococcus aureus. Reg Anesth. 1996; 21:239–242. PMID: 8744667.
38. Sakuragi T, Ishino H, Dan K. Bactericidal activity of 0.5% bupivacaine with preservatives on microorganisms in the human skin flora. Reg Anesth. 1997; 22:178–184. PMID: 9089861.


39. Sakuragi T, Ishino H, Dan K. Bactericidal activity of preservative-free bupivacaine on microorganisms in the human skin flora. Acta Anaesthesiol Scand. 1998; 42:1096–1099. PMID: 9809095.


40. Aydin ON, Eyigor M, Aydin N. Antimicrobial activity of ropivacaine and other local anaesthetics. Eur J Anaesthesiol. 2001; 18:687–694. PMID: 11553246.


41. Mullin GS, Rubinfeld RS. The antibacterial activity of topical anesthetics. Cornea. 1997; 16:662–665. PMID: 9395876.


42. Pere P, Lindgren L, Vaara M. Poor antibacterial effect of ropivacaine: comparison with bupivacaine. Anesthesiology. 1999; 91:884–886. PMID: 10485806.
43. Rodrigues AA, Pina-Vaz C, Mardh PA, Martinez-de-Oliveira J, Freitas-da-Fonseca A. Inhibition of germ tube formation by Candida albicans by local anesthetics: an effect related to ionic channel blockade. Curr Microbiol. 2000; 40:145–148. PMID: 10679044.
44. Batai I, Kerenyi M, Falvai J, Szabo G. Bacterial growth in ropivacaine hydrochloride. Anesth Analg. 2002; 94:729–731. PMID: 11867406.
45. Parr AM, Zoutman DE, Davidson JS. Antimicrobial activity of lidocaine against bacteria associated with nosocomial wound infection. Ann Plast Surg. 1999; 43:239–245. PMID: 10490173.


46. Pelz K, Wiedmann-Al-Ahmad M, Bogdan C, Otten JE. Analysis of the antimicrobial activity of local anaesthetics used for dental analgesia. J Med Microbiol. 2008; 57:88–94. PMID: 18065672.


47. Berg JO, Mossner BK, Skov MN, Lauridsen J, Gottrup F, Kolmos HJ. Antibacterial properties of EMLA and lidocaine in wound tissue biopsies for culturing. Wound Repair Regen. 2006; 14:581–585. PMID: 17014670.
48. Sedef Gocmen J, Buyukkocak U, Caglayan O, Aksoy A. In vitro antibacterial effects of topical local anesthetics. J Dermatolog Treat. 2008; 19:351–353. PMID: 18608709.
49. Liu K, Ye L, Sun W, Hao L, Luo Y, Chen J. Does Use of Lidocaine Affect Culture of Synovial Fluid Obtained to Diagnose Periprosthetic Joint Infection (PJI)? An In Vitro Study. Med Sci Monit. 2018; 24:448–452. PMID: 29360804.
50. Pina-Vaz C, Rodrigues AG, Sansonetty F, Martinez-De-Oliveira J, Fonseca AF, Mardh PA. Antifungal activity of local anesthetics against Candida species. Infect Dis Obstet Gynecol. 2000; 8:124–137. PMID: 10968594.


51. Abdelaziz AA, el-Nakeeb MA. Sporicidal activity of local anaesthetics and their binary combinations with preservatives. J Basic Microbiol. 1987; 27:403–410. PMID: 3134539.


52. Silva MT, Sousa JC, Polonia JJ, Macedo PM. Effects of local anesthetics on bacterial cells. J Bacteriol. 1979; 137:461–468. PMID: 104970.


53. Leung YW, Rawal BD. Mechanism of action of tetracaine hydrochloride against Pseudomonas aeruginosa. J Infect Dis. 1977; 136:679–683. PMID: 410897.
54. Fazly Bazaz BS, Salt WG. Local anaesthetics as antibacterial agents: effects on cellular respiration and the leakage of cytoplasmic constituents. Microbios. 1983; 37:139–149. PMID: 6353168.
55. Rodrigues A, Vaz CP, Fonseca AF, de Oliveira JM, Barros H. In vitro effect of local anesthetics on candida albicans germ tube formation. Infect Dis Obstet Gynecol. 1994; 1:193–197. PMID: 18475344.
56. Schmidt RM, Rosenkranz HS, Ryan JF. Inhibition of DNA synthesis by lidocaine and procaine. Experientia. 1971; 27:261–262. PMID: 5546633.


57. Reynolds MM, Greenwood-Quaintance KE, Patel R, Pulido JS. Selected Antimicrobial Activity of Topical Ophthalmic Anesthetics. Transl Vis Sci Technol. 2016; 5:2.


58. Anding K, Albrecht P, Heilmann C, Daschner F. The bactericidal effect of oxybuprocaine. A possible cause of false negative results in bronchoalveolar lavage. Anaesthesist. 1993; 42:619–622. PMID: 8214534.
59. Olsen KM, Peddicord TE, Campbell GD, Rupp ME. Antimicrobial effects of lidocaine in bronchoalveolar lavage fluid. J Antimicrob Chemother. 2000; 45:217–219. PMID: 10660504.


60. Chandan SS, Faoagali J, Wainwright CE. Sensitivity of respiratory bacteria to lignocaine. Pathology. 2005; 37:305–307. PMID: 16194830.


61. Aldous WK, Jensen R, Sieck BM. Cocaine and lidocaine with phenylephrine as topical anesthetics: antimicrobial activity against common nasal pathogens. Ear Nose Throat J. 1998; 77:554–557. PMID: 9693473.


62. Stratford AF, Zoutman DE, Davidson JS. Effect of lidocaine and epinephrine on Staphylococcus aureus in a guinea pig model of surgical wound infection. Plast Reconstr Surg. 2002; 110:1275–1279. PMID: 12360067.
63. Lu CW, Lin TY, Shieh JS, Wang MJ, Chiu KM. Antimicrobial effect of continuous lidocaine infusion in a Staphylococcus aureus-induced wound infection in a mouse model. Ann Plast Surg. 2014; 73:598–601. PMID: 25310128.
64. Rahn R. Endocarditis risk from dental procedures. Zahnarztl Prax. 1989; 40:48–52. PMID: 2623985.
65. Roberts MC. Antibiotic resistance in oral/respiratory bacteria. Crit Rev Oral Biol Med. 1998; 9:522–540. PMID: 9825225.


66. Baltch AL, Pressman HL, Hammer MC, Sutphen NC, Smith RP, Shayegani M. Bacteremia following dental extractions in patients with and without penicillin prophylaxis. Am J Med Sci. 1982; 283:129–140. PMID: 7044125.


67. Diz Dios P, Tomas Carmona I, Limeres Posse J, Medina Henriquez J, Fernandez Feijoo J, Alvarez Fernandez M. Comparative efficacies of amoxicillin, clindamycin, and moxifloxacin in prevention of bacteremia following dental extractions. Antimicrob Agents Chemother. 2006; 50:2996–3002. PMID: 16940094.


68. Ramos E, Santamaria J, Santamaria G, Barbier L, Arteagoitia I. Do systemic antibiotics prevent dry socket and infection after third molar extraction? A systematic review and meta-analysis. Oral Surg Oral Med Oral Pathol Oral Radiol. 2016; 122:403–425. PMID: 27499028.


69. Reiland MD, Ettinger KS, Lohse CM, Viozzi CF. Does Administration of Oral Versus Intravenous Antibiotics for Third Molar Removal Have an Effect on the Incidence of Alveolar Osteitis or Postoperative Surgical Site Infections? J Oral Maxillofac Surg. 2017; 75:1801–1808. PMID: 28438595.


70. Otten JE, Wiedmann-Al-Ahmad M, Jahnke H, Pelz K. Bacterial colonization on different suture materials--a potential risk for intraoral dentoalveolar surgery. J Biomed Mater Res B Appl Biomater. 2005; 74:627–635. PMID: 15889436.


71. Pineiro A, Tomas I, Blanco J, Alvarez M, Seoane J, Diz P. Bacteraemia following dental implants' placement. Clin Oral Implants Res. 2010; 21:913–918. PMID: 20701619.
72. Genovesi A, Barone A, Toti P, Covani U. The efficacy of 0.12% chlorhexidine versus 0.12% chlorhexidine plus hyaluronic acid mouthwash on healing of submerged single implant insertion areas: a short-term randomized controlled clinical trial. Int J Dent Hyg. 2017; 15:65–72. PMID: 26084554.


73. Chaveli-López B, Bagán-Sebastián JV. Treatment of oral mucositis due to chemotherapy. J Clin Exp Dent. 2016; 8:e201–e209. PMID: 27034762.


74. Maria OM, Eliopoulos N, Muanza T. Radiation-Induced Oral Mucositis. Front Oncol. 2017; 7:89. PMID: 28589080.


Table 1
Previous Studies of Local Anesthetics (LA) and Antimicrobial Action
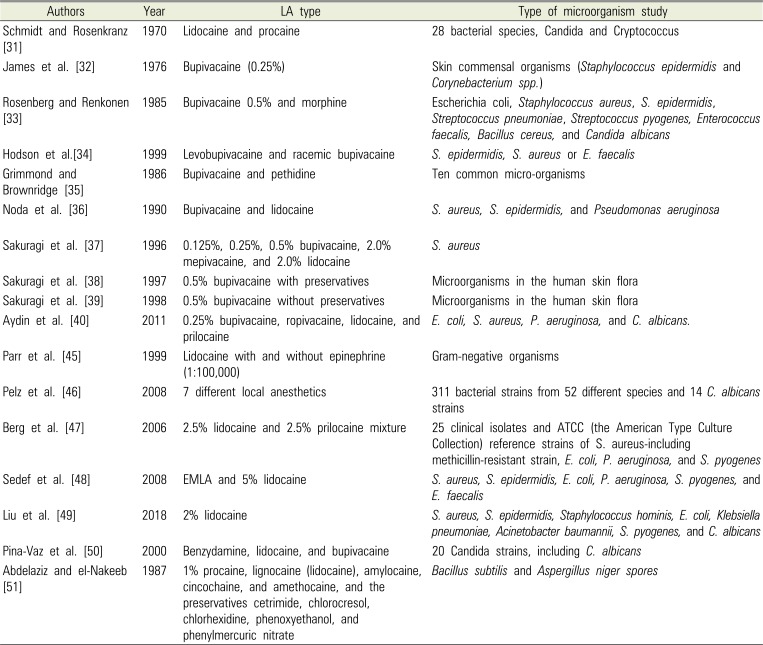
Authors | Year | LA type | Type of microorganism study |
---|---|---|---|
Schmidt and Rosenkranz [31] | 1970 | Lidocaine and procaine | 28 bacterial species, Candida and Cryptococcus |
James et al. [32] | 1976 | Bupivacaine (0.25%) | Skin commensal organisms (Staphylococcus epidermidis and Corynebacterium spp.) |
Rosenberg and Renkonen [33] | 1985 | Bupivacaine 0.5% and morphine | Escherichia coli, Staphylococcus aureus, S. epidermidis, Streptococcus pneumoniae, Streptococcus pyogenes, Enterococcus faecalis, Bacillus cereus, and Candida albicans |
Hodson et al.[34] | 1999 | Levobupivacaine and racemic bupivacaine | S. epidermidis, S. aureus or E. faecalis |
Grimmond and Brownridge [35] | 1986 | Bupivacaine and pethidine | Ten common micro-organisms |
Noda et al. [36] | 1990 | Bupivacaine and lidocaine | S. aureus, S. epidermidis, and Pseudomonas aeruginosa |
Sakuragi et al. [37] | 1996 | 0.125%, 0.25%, 0.5% bupivacaine, 2.0% mepivacaine, and 2.0% lidocaine | S. aureus |
Sakuragi et al. [38] | 1997 | 0.5% bupivacaine with preservatives | Microorganisms in the human skin flora |
Sakuragi et al. [39] | 1998 | 0.5% bupivacaine without preservatives | Microorganisms in the human skin flora |
Aydin et al. [40] | 2011 | 0.25% bupivacaine, ropivacaine, lidocaine, and prilocaine | E. coli, S. aureus, P. aeruginosa, and C. albicans. |
Parr et al. [45] | 1999 | Lidocaine with and without epinephrine (1:100,000) | Gram-negative organisms |
Pelz et al. [46] | 2008 | 7 different local anesthetics | 311 bacterial strains from 52 different species and 14 C. albicans strains |
Berg et al. [47] | 2006 | 2.5% lidocaine and 2.5% prilocaine mixture | 25 clinical isolates and ATCC (the American Type Culture Collection) reference strains of S. aureus-including methicillin-resistant strain, E. coli, P. aeruginosa, and S. pyogenes |
Sedef et al. [48] | 2008 | EMLA and 5% lidocaine | S. aureus, S. epidermidis, E. coli, P. aeruginosa, S. pyogenes, and E. faecalis |
Liu et al. [49] | 2018 | 2% lidocaine | S. aureus, S. epidermidis, Staphylococcus hominis, E. coli, Klebsiella pneumoniae, Acinetobacter baumannii, S. pyogenes, and C. albicans |
Pina-Vaz et al. [50] | 2000 | Benzydamine, lidocaine, and bupivacaine | 20 Candida strains, including C. albicans |
Abdelaziz and el-Nakeeb [51] | 1987 | 1% procaine, lignocaine (lidocaine), amylocaine, cincochaine, and amethocaine, and the preservatives cetrimide, chlorocresol, chlorhexidine, phenoxyethanol, and phenylmercuric nitrate | Bacillus subtilis and Aspergillus niger spores |
Table 2
Studies of Antimicrobial Mechanism of Action of Local Anesthetics (LA)
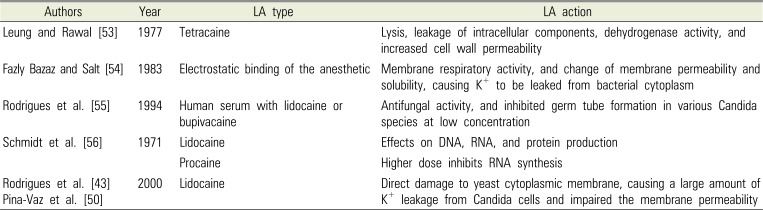
Authors | Year | LA type | LA action |
---|---|---|---|
Leung and Rawal [53] | 1977 | Tetracaine | Lysis, leakage of intracellular components, dehydrogenase activity, and increased cell wall permeability |
Fazly Bazaz and Salt [54] | 1983 | Electrostatic binding of the anesthetic | Membrane respiratory activity, and change of membrane permeability and solubility, causing K+ to be leaked from bacterial cytoplasm |
Rodrigues et al. [55] | 1994 | Human serum with lidocaine or bupivacaine | Antifungal activity, and inhibited germ tube formation in various Candida species at low concentration |
Schmidt et al. [56] | 1971 | Lidocaine | Effects on DNA, RNA, and protein production |
Procaine | Higher dose inhibits RNA synthesis | ||
Rodrigues et al. [43] | 2000 | Lidocaine | Direct damage to yeast cytoplasmic membrane, causing a large amount of K+ leakage from Candida cells and impaired the membrane permeability |
Pina-Vaz et al. [50] |
Table 3
Studies of Antimicrobial Effects of Local Anesthetics (LA) for Infection Control
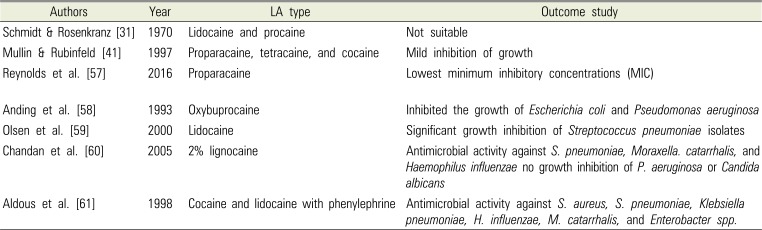
Authors | Year | LA type | Outcome study |
---|---|---|---|
Schmidt & Rosenkranz [31] | 1970 | Lidocaine and procaine | Not suitable |
Mullin & Rubinfeld [41] | 1997 | Proparacaine, tetracaine, and cocaine | Mild inhibition of growth |
Reynolds et al. [57] | 2016 | Proparacaine | Lowest minimum inhibitory concentrations (MIC) |
Anding et al. [58] | 1993 | Oxybuprocaine | Inhibited the growth of Escherichia coli and Pseudomonas aeruginosa |
Olsen et al. [59] | 2000 | Lidocaine | Significant growth inhibition of Streptococcus pneumoniae isolates |
Chandan et al. [60] | 2005 | 2% lignocaine | Antimicrobial activity against S. pneumoniae, Moraxella. catarrhalis, and Haemophilus influenzae no growth inhibition of P. aeruginosa or Candida albicans |
Aldous et al. [61] | 1998 | Cocaine and lidocaine with phenylephrine | Antimicrobial activity against S. aureus, S. pneumoniae, Klebsiella pneumoniae, H. influenzae, M. catarrhalis, and Enterobacter spp. |
Table 4
Studies of Antimicrobial Effects of Local Anesthetics (LA) in Dentistry
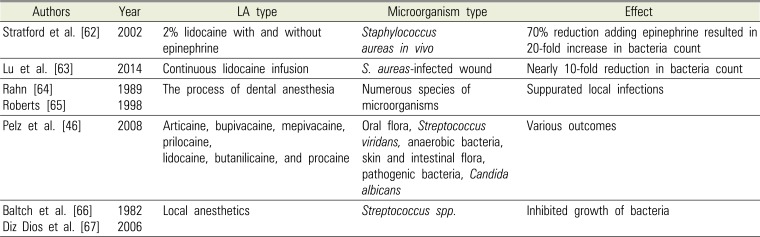
Authors | Year | LA type | Microorganism type | Effect |
---|---|---|---|---|
Stratford et al. [62] | 2002 | 2% lidocaine with and without epinephrine | Staphylococcus aureas in vivo | 70% reduction adding epinephrine resulted in 20-fold increase in bacteria count |
Lu et al. [63] | 2014 | Continuous lidocaine infusion | S. aureas-infected wound | Nearly 10-fold reduction in bacteria count |
Rahn [64] | 1989 | The process of dental anesthesia | Numerous species of microorganisms | Suppurated local infections |
Roberts [65] | 1998 | |||
Pelz et al. [46] | 2008 | Articaine, bupivacaine, mepivacaine, prilocaine, lidocaine, butanilicaine, and procaine | Oral flora, Streptococcus viridans, anaerobic bacteria, skin and intestinal flora, pathogenic bacteria, Candida albicans | Various outcomes |
Baltch et al. [66] | 1982 | Local anesthetics | Streptococcus spp. | Inhibited growth of bacteria |
Diz Dios et al. [67] | 2006 |