Abstract
Purpose
Materials and Methods
Results
Figures and Tables
Fig. 1
Morphologic characteristics in patient-derived three-dimensional (3D) organoid culture of renal cell carcinoma. (A) Clear cell renal cell carcinoma morphology in primary tissue by hematoxylin and eosin (H&E) stain. (B) 3D organoid culture under light microscopy shows diverse colonies. (C) Paraffin blocked samples of 3D organoid culture show morphology similar to that of clear cell renal cell carcinoma in primary tissue. All scale bars, 20 µm.
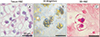
Fig. 2
Biomarker comparison in two-dimensional (2D) culture, patient-derived three-dimensional (3D) organoid culture, and primary tissue. (A) Immunofluorescence analysis of carbonic anhydrase 9 (CA9) (green), keratin (red), vimentin (green), and 4′,6-diamidino-2-phenylindole (DAPI) (blue) expression in 2D cultured cancer and normal cells. Scale bars, 10 µm. (B) CA9 and keratin immunofluorescence in 3D organoid culture of cancer and normal cells. Scale bars, 10 µm. (C) Immunohistochemistry (IHC) staining of CA9 and vimentin in primary tissue from normal and renal cancer patients. (D) IHC scores of renal cancer tissue in 5 patients. (E) Real-time polymerase chain reaction fold induction of CA9 and vimentin in primary tissue of renal cancer patients relative to normal tissue.
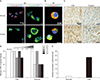
Fig. 3
Patient-derived three-dimensional (3D) organoid culture of renal cancer cells exhibits increased expression of carbonic anhydrase 9 (CA9) and vimentin. (A) Expression of CA9 and vimentin in 3D organoid culture (passage 4) and two-dimensional (2D) culture by an immunofluorescence assay. Scale bars, 20 µm. (B) Real-time polymerase chain reaction (RT-PCR) relative mean intensity scores of CA9 and vimentin in 3D organoid culture compared to 2D culture (p<0.05). Error bar represents standard error of mean. (C) RT-PCR fold induction of CA9 and vimentin in 3D culture relative to 2D culture. (D) Western blot of CA9 in 3D organoid culture and 2D culture. GAPDH, glyceraldehyde-3-phosphate dehydrogenase.
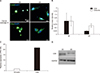
ACKNOWLEDGMENTS
Notes
AUTHORS' CONTRIBUTIONS
Research conception and design: Woong Kyu Han.
Data acquisition: Sook Young Kim.
Statistical analysis: Joon Chae Na and Sook Young Kim.
Data analysis and interpretation: Joon Chae Na.
Drafting of the manuscript: Joon Chae Na and Jee-Hoon Kim.
Critical revision of the manuscript: Woong Kyu Han.
Obtaining funding: Woong Kyu Han.
Administrative, technical, or material support: Young-Ran Gu, Dae-Young Jun, Hyung Ho Lee, Young Eun Yoon, and Kyung Hwa Choi.
Supervision: Sung Joon Hong.
Approval of the final manuscript: Woong Kyu Han.
References









































SUPPLEMENTARY MATERIALS
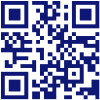