Abstract
The use of three-dimensional (3D) printing is becoming more common, including in the field of orthopaedic surgery. There are currently four primary clinical applications for 3D-printing in hip and pelvic surgeries: (i) 3D-printed anatomical models for planning and surgery simulation, (ii) patient-specific instruments (PSI), (iii) generation of prostheses with 3D-additive manufacturing, and (iv) custom 3D-printed prostheses. Simulation surgery using a 3D-printed bone model allows surgeons to develop better surgical approaches, test the feasibility of procedures and determine optimal location and size for a prosthesis. PSI will help inform accurate bone cuts and prosthesis placement during surgery. Using 3D-additive manufacturing, especially with a trabecular pattern, is possible to produce a prosthesis mechanically stable and biocompatible prosthesis capable of promoting osseointergration. Custom implants are useful in patients with massive acetabular bone loss or periacetabular malignant bone tumors as they may improve the fit between implants and patient-specific anatomy. 3D-printing technology can improve surgical efficiency, shorten operation times and reduce exposure to radiation. This technology also offers new potential for treating complex hip joint diseases. Orthopaedic surgeons should develop guidelines to outline the most effective uses of 3D-printing technology to maximize patient benefits.
Three-dimensional (3D) printing technology is additive manufacturing that produces 3D shapes by stacking twodimensional (2D) cross-sectional shapes with various materials. It is used for laminated manufacturing, such as rapid prototyping (RP). In the early days, there were a number of key limitations in printing materials, molding time, size, precision and strength of the moldings, which limited industrial uses. In recent years, however, printing materials have been diversified including metals, and printing equipment has been developed accordingly. The paradigm of industrial use is rapidly changing as the production of complex shapes and customized products become possible.
Today, 3D-printing technology can be used to create simulation models or medical implants, thus significantly aiding doctors and medical companies by optimizing the way a surgeon plans and executes a procedure1). The application of 3D-printing technology to clinical medicine has already become the fastest growing innovation in the medical field2).
The use of 3D-printing in the field of orthopaedic surgery is rapidly increasing. The ease of segmenting bone from computed tomography (CT) scans, and the variety of available 3D printers enables researchers, manufacturers, and surgeons to easily use 3D-printing technology. Over the last decade, there have been significant developments in the orthopedic surgery field, especially in hip and pelvic surgeries. Many scientific papers have been published regarding research in the field of 3D-printed hip prosthesis3).
Currently, the use of 3D-printing technology in hip and pelvic surgeries can be divided into four important categories. First is the development of anatomical models based on patient imaging; these bone models can facilitate an improved understanding of the patho-anatomy and surgeons can use it to simulate the surgery to potentially improve execution. Second is the synthesis of patient-specific instruments (PSI) that may increase the accuracy of a surgery; most PSIs are manufactured in the form of guides or jigs. Third is the production of arthroplasty implants; 3D-printed hip implants are advantageous because ingrowth surfaces can be modified to optimize bony ingrowth. Forth is the development of custom implants. Unlike standard sized implants, a custom implant created using patient-specific medical images can be a perfect match for the patient's unique anatomy. Importantly, the use of 3D-printed custom implants has recently been reported in not only pelvic tumor surgery but also in difficult revision hip arthroplasty with severe acetabular bone loss4).
In conventional orthopedic procedures, surgeons have to integrate all preoperative 2D images and formulate a 3D-surgical plan. 2D-CT images can be reconstructed into 3D-stereoscopic images, but eventually the information is received two-dimensionally by the surgeon via a computer monitor. For surgeries involving the hip and pelvis, preoperative planning is particularly difficult especially in cases of complex anatomy and severe deformity. Therefore, a variety of new preoperative planning methods are being developed, such as via digital radiographs5). Above all, however, 3D-printed anatomical models provide surgeons with an opportunity to better understand individual patient anatomy. Simulation surgery using 3D-printed bone models allows a surgeon to develop better surgical approaches, test the feasibility of procedures and determine the optimal location and size of implants.
Intertrochanteric fractures (ITF) are one of the most common fractures of the hip and primarily occur in elderly people with osteoporosis. Intramedullary fixation has become the most commonly used method of treating unstable ITF. Zheng et al.6) performed CT scanning and used proximal femur nail antirotation (PFNA) with a 3D RP model for a group of 19 patients and compared outcomes to another group (n=20) who underwent conventional PFNA operation. The 3D RP model was generated using the fused deposition modeling technique and the length and diameter of the main screw blade was measured during the simulation. It was demonstrated that the 3D RP model-assisted procedure resulted in more effective reduction of the neck shaft angle. Furthermore, patients undergoing surgery with the 3D RP model experienced a significant reduction in surgery duration (P<0.01), and reductions in intraoperative (P=0.02) and postoperative (P=0.03) blood loss, compared with those treated with conventional surgery. In this study, the 3D RP modelling technique was able to create an accurate model of the ITF, which facilitated surgical planning and fracture reduction, thus improving the efficiency of PFNA surgery for ITFs.
Treatments for acetabular fractures aim to restore the biomechanical property of the pelvis and acetabulum through reconstructing the articular surface and restoring anatomical structure. Because of the complex anatomy of the pelvis and nearby neurovascular structure, acetabular fracture surgery has always been challenging.
In 2015, Yu et al.7) published on the benefit of augmented stereoscopic 3D CT reconstructions to allow for an appreciation of the normal 3D anatomy of the pelvis on the fractured side and use of models for subsequent intraoperative contouring of pelvic reconstruction plates. In 2019, Chen et al.8) summarized the results of a retrospective analysis of 52 bicolumnar acetabular fracture cases which were divided into two groups for comparison; patients in group A (n=28 patients) underwent, computer-assisted virtual surgical procedures and 3D printing of patient-specific precontoured plates, while patients in group B (n=24) were treated with the conventional method. Fracture type, operative blood loss, surgical time, complications, radiographic quality of reduction, and hip function were compared between groups. The final surgical procedure conducted on all patients in group A was almost identical to the preoperative virtual operation. Operative time and intraoperative blood loss were significantly reduced in group A compared with group B (P<0.05); quality of postoperative fracture reduction and patient satisfaction with resultant hip function was also slightly higher in group A compared with group B (Fig. 1).
Total hip arthroplasty (THA) in dysplastic hips or sequelae of septic arthritis is technically demanding due to the distorted anatomy. Patient specific (CT-based), 3D RP models were used to plan for acetabular cup placement so that a surgeon could identify pelvic structures, assess the ideal extent of reaming and determine the size of cup after a reconstructive procedure.
In 2013, Won et al.9) published the results of a THA study using an RP model in 21 complex hips; all surgeries were completed successfully and the acetabular component used was within 2 mm of the predicted size in 17 hips (80.9%). All of the acetabular components and femoral stems had radiological evidence of bone ingrowth and stability at the final follow-up (mean, 35.5 months), without any detectable wear or peri-prosthetic osteolysis. Several similar studies have been reported in recent years1011).
Orthopaedic surgeons and engineers are constantly looking for methods to improve the surgical positioning of prostheses in accordance with the purpose of the operation. Navigations and robotics have been used to improve the accuracy of hip surgery but have had limited use because of high costs, increased operative times and other logistical issues. More recently, PSI has been developed to guide the positioning of a prosthesis during orthopaedic surgery. We reviewed the currently reported PSI techniques used for hip surgery.
Cam-type femoro-acetabular impingement (FAI) can be treated by performing an osteochondroplasty to remove the excess impinging bone from the head neck junction of femur. Verma et al.12) reported a case of Cam-type FAI in an 18 year-old male, who underwent surgical treatment by osteochondroplasty. CT-based virtual surgical planning was conducted to design the femoral head and neck jigs, which were 3D printed and used intra-operatively to guide for adequate and optimum excision of bone at the femoral head neck junction. They concluded that their customized jigs were accurate and useful for the surgery.
For the treatment of early osteonecrosis of femoral head, the conventional method of core decompression is usually performed under C-arm fluoroscopy. However, in 2018, Li et al.13) reported the clinical efficacy of a 3D-printing guide plate for core decompression instead of C-arm fluoroscopy. The 3D-printed guide plate could be tightly attached to the proximal part of the femur during the operation and one Kirschner wire could be inserted into the pinhole on the guide plate to obtain an appropriate decompression position. Compared with 20 patients who underwent conventional core decompression under C-arm, fluoroscopy time, and intraoperative blood loss in 20 patients treated with a 3D-printing guide plate was significantly less. There was also a significant difference between the groups in the last follow-up of Harris hip scores (HHS).
Recently, PSI has been developed to guide the positioning of components during hip arthroplasty. This technique uses imaging techniques such as CT and magnetic resonance imaging to plan surgery in a virtual 3D environment. The surgeon can plan orientation and position of the prosthesis relative to a chosen standard frame of reference and execute the plan using simple intraoperative patient-specific guides.
Acetabular guidance systems aim to optimize the cup size, implant medialization, anteversion, and inclination. Femoral guidance systems aim to optimize the stem size and alignment, offset, leg length (height of neck cut), and stem version. Several PSI systems (e.g., Signature Hip [Zimmer Biomet], OPS [Corin Group], Hip Plan [Symbios], and MyHip [Medacta]) are currently licensed for use in the United States and Europe14) (Fig. 2).
PSI THA guides have been shown to improve the accuracy of implant positioning and may play an important role in reconstructing complex anatomy. In a prospective randomized controlled trial, Small et al.15) compared 18 patients undergoing THA with conventional instrumentation and 18 patients undergoing THA with PSI. Results demonstrated a statistically significant difference in version of the acetabular component between standard instrumentation and PSI. However, the effects on long-term functional outcomes or survival of PSI THA guides are controversial. More clinical research is needed to better characterize the potential benefits of PSI in terms of surgical accuracy.
Most applications of 3D-printing technology in the orthopedic area have focused on high customization or patient-specific manufacturing. On the other hand, advances have been made in terms of materials that can be leveraged along with 3D-printing technology. In particular, the material called trabecular titanium, which has a structure similar to the trabecular bone of the human body, can be reproduced only with high-quality additive manufacturing technology. By applying trabecular titanium material to a hip prosthesis, it is possible to produce a prosthesis that is mechanically stability, biocompatible while promoting osseointergration.
Recently, the development of electron beam melting 3D-printing technology has led to orthopaedic manufacturing with integrated trabecular surfaces, melting thin layers of metal powder in finely tuned 3D constructs1617). This additive manufacturing provided a bulk cup with a constant porosity (65%), each pore sizing 700µm16). Such a pore size, which is not achievable through conventional manufacturing approaches was demonstrated to provide a high fixation capacity in the early weeks and a deep bone ingrowth in an in vivo study by Taniguchi et al.18). Asti et al.19) also reported the results of an in vitro study revealing that a trabecular titanium scaffold facilitated better osseointegration compared with other synthetic scaffolds.
Hip prostheses made from high porous trabecular titanium is already used worldwide. In 2019, Castagnini et al.20) reported eight years results comparing and evaluating survival rates and reason for revisions with trabecular titanium cups and conventional cementless cups. Trabecular titanium cups had a statistically higher survival rate compared with the control group and a statistically lower incidence of cup aseptic loosening (Fig. 3).
Arabnejad et al.21) reported that 3D-printed fully porous implants can reduce the amount of bone loss secondary to stress shielding by 75% compared to a conventional fully solid implant. This result also agrees with those of the in vitro quasi-physiological experimental model and the corresponding finite element model for both the optimized fully porous and fully solid implant. These studies demonstrate the merit and potential of modifying material architecture to achieve substantial reduction of bone resorption secondary to stress shielding (Fig. 4).
Custom implants may be indicated when: (i) patients' bony geometries fall outside the range of standard implants with respect to implant size- or disease-specific requirements, and (ii) improved surgical results are anticipated due to a better fit between implants and patients' anatomical needs22).
The management of acetabular bone loss is a challenging problem in revision THA2324). Multiple surgical reconstruction options have been described for severe acetabular defects including use of a jumbo acetabular cup, impaction bone grafting combined with a cemented cup, structural allograft, oblong acetabular cup, antiprotrusio cage, and cup-cage construct2526272829). Several studies have assessed a technique to analyze the defect in detail and to reconstruct the acetabulum using a custom-made trabecular titanium implant that matches the anatomy of the bone deficient acetabulum, taking into account the patient's bone quality to achieve primary implant stability293031). Custom-made triflanged acetabular components (CTAC) were developed to restore hip biomechanics and achieve primary implant stability, even in cases of extreme acetabular bone loss. Implant stability is favored by maximizing host bone contact and by three flanges that fit the iliac, ischial and pubic bone32333435). The design of CTACs implants is based on 3D models produced from CT scans. Patient-specific bony situation is evaluated and an implant proposal is formulated including a biomechanical assessment. The implant proposal also includes patient-specific screw positions based on bone quality and drill guides to achieve the planned position. Moreover, the medial side of CTACs implants can be developed with a porous defect-filling scaffold to promote osteointegration (Fig. 5).
De Martino et al.36) published a systematic review of 17 articles assessing the clinical results of CTACs; a total of 579 CTACs were implanted. The all-cause revision-free survivorship was 82.7%. The overall complication rate was 29%. Dislocation and infection were the most common complications observed with an incidence of 11% and 6.2%, respectively. This could be due to the extensive approach and the poor quality of the soft tissues in multi-operated patients.
According to current data, CTACs have a high incidence of complications. However, since most cases have been used in patients with significant acetabular bone loss or pelvic discontinuity, CTACs remain an efficacious treatment option and have potential for development. Despite a high complication rate, the use of such implants seems justified in carefully selected patients with massive uncontained acetabular bone defects.
Historically, the most common method of managing periacetabular malignant bone tumors was hindquarter amputation or external hemipelvectomy37). Prosthetic reconstruction may be a promising treatment for peri-acetabular malignant bone tumors with advances in radiotherapy and chemotherapy38394041). However, this approach is associated with a high complication rate. Therefore, research on prosthesis design is needed, and 3D-printing technology is emerging as an alternative because of acceptable cosmesis, immediate stability, and the possibility of rapid recovery, as well as early weight-bearing activity. Recently, 3D-printed prostheses have been used for hemipelvic reconstruction and have obtained good short-term functional results4243).
Liang et al.44) reported the results of 35 patients who underwent resection of a pelvic tumor and reconstruction using a 3D-printed prosthesis. Three patients underwent reconstruction using an iliac prosthesis, 12 using a standard hemipelvic prosthesis and 20 using a screw-rod connected hemipelvic prosthesis. After a mean follow-up of 20.5 months, 25 patients survived without evidence of disease, five were alive with disease and five had died from metastatic disease. For the surviving patients, the mean clinical score (Musculoskeletal Tumor Society 93 score) was improved.
Wang et al.45) reported 11 patients with periacetabular malignant bone tumors treated by personalized 3D-printed hemipelvic prostheses after en bloc resection. During an average follow-up of 15.5 months, patients have shown acceptable functional results without severe complications. Hip dislocation was detected in two patients, while delayed wound healing occurred in one patient. Local tumor recurrence was not observed. They concluded that reconstruction arthroplasty using 3D-printed pelvic prostheses can facilitate the precise matching and osseointegration between implants and the host bone. Reconstruction arthroplasty using 3D-printed pelvic prosthesis provides a promising alternative for those patients with peri-acetabular malignant bone tumors.
An increase in research and publications involving 3D-printing applications in orthopedic surgery and related fields was observed, especially in recent ten years. Overall, most papers were published from China (75 papers), followed by United States (69 papers), United Kingdom (17 papers), Australia (15 papers), and India (11 papers). The number of studies published in Korea (8 papers) on orthopaedic applications of 3D-printing technology is relatively low compared to that of the USA, China, and advanced European countries (Fig. 6)46).
3D-printing technology has many advantages when applied to orthopaedic surgeries, especially in the hip and pelvis, however, it is not without its limitations. Firstly, the accuracy and resolution remain to be further proven. Soft tissue and cartilage are usually excluded during the processing of CT-based radiological images. Depending on the purpose of technology, the inclusion of such information may be affected. When constructing a 3D-printed model or PSI, structures such as blood vessels, nerves and tendons are not taken into account, which may lead to unexpected problems during surgery. Moreover, the 3D printing process can be influenced by artificial factors. Additionally, the cost of 3D printing is relatively expensive. At present, the cost for a 3D model of the hip and hemipelvis can vary from $200 to $1,000 depending upon factors, such as the materials used, the size of the print, and the type of printer used47).
A few years ago, only plastics could be 3D-printed into anatomic models and PSIs. Then, metal powders, such as titanium or cobalt-chrome, were used to fabricate custom implants by 3D printing48). The advances in biomimetic materials for 3D printing will be one major direction for future development in 3D-printing technology applied to the development of patient-specific orthopedics. The material should be biocompatible and sterilizable for intraoperative use49).
Surgeons might not easily master the steps in the working process such as image postprocessing, creating 3D images that highlight regions of interest, planning a surgery with various reconstructive options, and even preoperative biomechanical evaluation of the implant design or a reconstructive method. To increase the popularity of 3D-printing technology among orthopedic surgeons, an integrated all-in-one computer platform should be developed to allow for easy planning and seamless communication among different care providers (e.g., radiologists, orthopaedic surgeons, engineers, implant companies)50)
In order to actively apply 3D-printing technology to the hip surgery field and expand the market, there is a need to improve the medical insurance cost. Let's take a look at the situation in countries that are leaders in 3D-printing technology. The US FDA applies an exception that exempts the approval process for cases where 3D-printing related medical technologies are not based on FDA guidelines, but where their effectiveness and safety are demonstrated. In Japan, national medical insurance have been applied to 3D-printing simulation modeling techniques since 2016. Currently, preoperative planning and simulation surgery using 3D-printing technology is used to perform various orthopaedic surgeries, including hip and pelvic surgery.
In Korea, some surgeons have combined 3D-printing technology with orthopaedic surgery and consistently appreciate its usefulness and potential, however, the government's policies have not yet reached the level of advanced countries. In particular, in orthopaedic surgery, despite the fact that 3D-printing technology has many possible applications and may be the best option in certain cases, more effort to gain government support is required. Also, the Korean FDA should be more proactive to ensure that the standards and guidance for 3D applications can guarantee the safe and effective use of 3D-printed orthopaedic devices.
For the hip and pelvis, simple plastic bone models are very useful in planning some difficult cases of fracture and arthroplasty for deformed hips. PSI may have limited additional benefits for routine hip arthroplasty, but maybe much useful in complex pelvic tumor resection and complex reconstruction. 3D-printed metallic implants are increasingly used for the reconstruction of critically sized acetabular defects in complex revision hip replacement surgery and tumor endoprosthetic reconstruction in many countries. We look forward to seeing a larger series of clinical studies, but the guidelines are still lacking. In the near future, access to 3D-printing technology will gradually increase in the medical field. Orthopaedic surgeons should be aware of the various degrees of regulatory requirements imposed by different countries and implement necessary validation and quality assurance steps when using customized tools and implants. Orthopaedic surgeons should also develop guidelines that increase the benefit of patients through discussion and collaboration with governments and engineering groups.
Figures and Tables
Fig. 1
(A) The real sized models of the fractured hemiplevis and mirrored healthy hemipelvis were printed using 3-dimensional printing. (B) Then preoperative contouring of the plates on the mirrored hemipelvis model was performed while being consistent with virtual surgical procedures. (C) Postoperative radiograph showing the iliac oblique view of pelvis. Data from the article of Chen et al. (Orthop Traumatol Surg Res. 2019;105:877-84)8) published by Elsevier Masson SAS. All rights reserved.
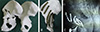
Fig. 2
Images showing the MyHip™ patient-specific instruments system. (A) The acetabular guide is seated into the acetabulum, and two pins are inserted through attached drill sleeves. The guide is removed, leaving the two pins to act as a guide to reaming and component placement. (B) The femoral guide has a contoured fit to the femoral neck and is kept in place for the neck cut by two intraosseous pins. Data from Medacta, Chicago, IL, USA.
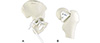
Fig. 3
Highly porous cups in total hip arthroplasty were eveloped in order to furtherly improve the osseointegration, enhance the long-term durability, and reduce the rate of aseptic loosening. Data from Lima Corporate, Udine, Italy.
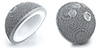
Fig. 4
Regions prone to bone resorption in Gruen zones 1–7 for (A) fully solid implant and for (B) fully porous implant with tailored relative density distribution. The fully porous implant with an optimized material micro-structure can reduce the amount of bone loss secondary to stress shielding by 75% compared to a fully solid implant. Data from the article of Arabnejad et al. (Int Orthop. J Orthop Res. 2017;35:1774-83)21).
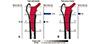
Fig. 5
(A) Preoperative radiograph after three revision surgeries with massive acetabular bone loss. (B) The design of custom-made triflanged acetabular components (CTAC) is based on 3-dimensional models produced from computed tomography scans taking into account the patient's anatomical geometry. (C) The medial side of CTAC can be provided with a porous defect-filling scaffold to favor osteointegration. (D) Postoperative radiograph.
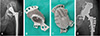
References
1. Hegde A. Healthcare 3D printing market 2018 prominent players - Aprecia pharmaceuticals, Aspect Biosystems, Bio 3D Technologies, BioBots, Cyfuse Biomedical, Digilab, 3Dynamics Systems, Envision TEC, Luxexcel, Materialise NV, Nano3D Biosciences, Oceanz, Organovo Ho [Internet]. Cleveland (OH): Global Market Insights, Inc.;2018. 08. 03. cited 2019 Oct 18. Available from: https://www.openpr.com/news/1160692/healthcare-3d-printing-market-2018-prominent-players-aprecia-pharmaceuticals-aspect-biosystemsbio-3d-technologies-biobots-cyfuse-biomedical-digilab-3dynamics-systems-envision-tec-luxexcel-materialise-nvnano3d-biosciences-oceanz-organovo-ho.html.
2. Ventola CL. Medical applications for 3D printing: current and projected uses. Pharm Ther. 2014; 39:704–711.
3. Xia RZ, Zhai ZJ, Chang YY, Li HW. Clinical applications of 3-dimensional printing technology in hip joint. Orthop Surg. 2019; 11:533–544.



4. Baauw M, van Hellemondt GG, van Hooff ML, Spruit M. The accuracy of positioning of a custom-made implant within a large acetabular defect at revision arthroplasty of the hip. Bone Joint J. 2015; 97:780–785.


5. Shin JK, Son SM, Kim TW, Shin WC, Lee JS, Suh KT. Accuracy and reliability of preoperative on-screen templating using digital radiographs for total hip arthroplasty. Hip Pelvis. 2016; 28:201–207.



6. Zheng SN, Yao QQ, Mao FY, et al. Application of 3D printing rapid prototyping-assisted percutaneous fixation in the treatment of intertrochanteric fracture. Exp Ther Med. 2017; 14:3644–3650.



7. Yu AW, Duncan JM, Daurka JS, Lewis A, Cobb J. A feasibility study into the use of three-dimensional printer modelling in acetabular fracture surgery. Adv Orthop. 2015; 2015:617046.


8. Chen K, Yang F, Yao S, et al. Application of computer-assisted virtual surgical procedures and three-dimensional printing of patient-specific pre-contoured plates in bicolumnar acetabular fracture fixation. Orthop Traumatol Surg Res. 2019; 105:877–884.


9. Won SH, Lee YK, Ha YC, Suh YS, Koo KH. Improving pre-operative planning for complex total hip replacement with a Rapid Prototype model enabling surgical simulation. Bone Joint J. 2013; 95:1458–1463.


10. Xu J, Li D, Ma RF, Barden B, Ding Y. Application of rapid prototyping pelvic model for patients with DDH to facilitate arthroplasty planning: a pilot study. J Arthroplasty. 2015; 30:1963–1970.


11. Faur C, Crainic N, Sticlaru C, Oancea C. Rapid prototyping technique in the preoperative planning for total hip arthroplasty with custom femoral components. Wien Klin Wochenschr. 2013; 125:144–149.


12. Verma T, Mishra A, Agarwal G, Maini L. Application of three dimensional printing in surgery for cam type of femoro-acetabular impingement. J Clin Orthop Trauma. 2018; 9:241–246.



13. Li B, Lei P, Liu H, et al. Clinical value of 3D printing guide plate in core decompression plus porous bioceramics rod placement for the treatment of early osteonecrosis of the femoral head. J Orthop Surg Res. 2018; 13:130.



14. Henckel J, Holme TJ, Radford W, Skinner JA, Hart AJ. 3D-printed patient-specific guides for hip arthroplasty. J Am Acad Orthop Surg. 2018; 26:e342–e348.


15. Small T, Krebs V, Molloy R, Bryan J, Klika AK, Barsoum WK. Comparison of acetabular shell position using patient specific instruments vs. standard surgical instruments: a randomized clinical trial. J Arthroplasty. 2014; 29:1030–1037.


16. Ohldin P. Series production of CE-certified orthopedic implants with integrated porous structures for improved bone ingrowth [Internet]. Vienna: Annals of DAAAM & Proceedings;2010. cited 2019 Sep 27. Available from: http://connection.ebscohost.com/c/articles/55675250/series-production-cecertified-orthopedic-implants-integrated-porous-structuresimproved-bone-ingrowth.
17. Regis M, Marin E, Fedrizzi L, Pressacco M. Additive manufacturing of Trabecular Titanium orthopedic implants. MRS Bull. 2015; 40:137–144.


18. Taniguchi N, Fujibayashi S, Takemoto M, et al. Effect of pore size on bone ingrowth into porous titanium implants fabricated by additive manufacturing: an in vivo experiment. Mater Sci Eng C Mater Biol Appl. 2016; 59:690–701.


19. Asti A, Gastaldi G, Dorati R, et al. Stem cells grown in osteogenic medium on PLGA, PLGA/HA, and titanium scaffolds for surgical applications. Bioinorg Chem Appl. 2010; 2010:831031.


20. Castagnini F, Bordini B, Stea S, Calderoni PP, Masetti C, Busanelli L. Highly porous titanium cup in cementless total hip arthroplasty: registry results at eight years. Int Orthop. 2019; 43:1815–1821.


21. Arabnejad S, Johnston B, Tanzer M, Pasini D. Fully porous 3D printed titanium femoral stem to reduce stress-shielding following total hip arthroplasty. J Orthop Res. 2017; 35:1774–1783.


22. Rengier F, Mehndiratta A, von Tengg-Kobligk H, et al. 3D printing based on imaging data: review of medical applications. Int J Comput Assist Radiol Surg. 2010; 5:335–341.


23. Paprosky WG, Perona PG, Lawrence JM. Acetabular defect classification and surgical reconstruction in revision arthroplasty. A 6-year follow-up evaluation. J Arthroplasty. 1994; 9:33–44.


24. Berry DJ, Lewallen DG, Hanssen AD, Cabanela ME. Pelvic discontinuity in revision total hip arthroplasty. J Bone Joint Surg Am. 1999; 81:1692–1702.


25. Abolghasemian M, Tangsataporn S, Sternheim A, Backstein D, Safir O, Gross AE. Combined trabecular metal acetabular shell and augment for acetabular revision with substantial bone loss: a mid-term review. Bone Joint J. 2013; 95:166–172.

26. Banerjee S, Issa K, Kapadia BH, Pivec R, Khanuja HS, Mont MA. Systematic review on outcomes of acetabular revisions with highly-porous metals. Int Orthop. 2014; 38:689–702.


27. Beckmann NA, Weiss S, Klotz MC, Gondan M, Jaeger S, Bitsch RG. Loosening after acetabular revision: comparison of trabecular metal and reinforcement rings. A systematic review. J Arthroplasty. 2014; 29:229–235.


28. Sculco PK, Ledford CK, Hanssen AD, Abdel MP, Lewallen DG. The evolution of the cup-cage technique for major acetabular defects: full and half cup-cage reconstruction. J Bone Joint Surg Am. 2017; 99:1104–1110.

29. Barlow BT, Oi KK, Lee YY, Carli AV, Choi DS, Bostrom MP. Outcomes of custom flange acetabular components in revision total hip arthroplasty and predictors of failure. J Arthroplasty. 2016; 31:1057–1064.


30. Moore KD, McClenny MD, Wills BW. Custom triflange acetabular components for large acetabular defects: minimum 10-year follow-up. Orthopedics. 2018; 41:e316–e320.


31. Myncke I, van Schaik D, Scheerlinck T. Custom-made triflanged acetabular components in the treatment of major acetabular defects. Short-term results and clinical experience. Acta Orthop Belg. 2017; 83:341–350.

32. Colen S, Harake R, De Haan J, Mulier M. A modified custommade triflanged acetabular reconstruction ring (MCTARR) for revision hip arthroplasty with severe acetabular defects. Acta Orthop Belg. 2013; 79:71–75.

33. DeBoer DK, Christie MJ, Brinson MF, Morrison JC. Revision total hip arthroplasty for pelvic discontinuity. J Bone Joint Surg Am. 2007; 89:835–840.


34. Wind MA Jr, Swank ML, Sorger JI. Short-term results of a custom triflange acetabular component for massive acetabular bone loss in revision THA. Orthopedics. 2013; 36:e260–e265.


35. Taunton MJ, Fehring TK, Edwards P, Bernasek T, Holt GE, Christie MJ. Pelvic discontinuity treated with custom triflange component: a reliable option. Clin Orthop Relat Res. 2012; 470:428–434.


36. De Martino I, Strigelli V, Cacciola G, Gu A, Bostrom MP, Sculco PK. Survivorship and clinical outcomes of custom triflange acetabular components in revision total hip arthroplasty: a systematic review. J Arthroplasty. 2019; 34:2511–2518.


37. Carter SR, Eastwood DM, Grimer RJ, Sneath RS. Hindquarter amputation for tumours of the musculoskeletal system. J Bone Joint Surg Br. 1990; 72:490–493.


38. Ayvaz M, Bekmez S, Mermerkaya MU, Caglar O, Acaroglu E, Tokgozoglu AM. Long-term results of reconstruction with pelvic allografts after wide resection of pelvic sarcomas. ScientificWorldJournal. 2014; 2014:605019.


39. Puri A, Gulia A, Pruthi M. Outcome of surgical resection of pelvic osteosarcoma. Indian J Orthop. 2014; 48:273–278.



40. Zang J, Guo W, Yang Y, Xie L. Reconstruction of the hemipelvis with a modular prosthesis after resection of a primary malignant peri-acetabular tumour involving the sacroiliac joint. Bone Joint J. 2014; 96:399–405.


41. Sun W, Li J, Li Q, Li G, Cai Z. Clinical effectiveness of hemipelvic reconstruction using computer-aided custom-made prostheses after resection of malignant pelvic tumors. J Arthroplasty. 2011; 26:1508–1513.


42. Chen X, Xu L, Wang Y, Hao Y, Wang L. Image-guided installation of 3D-printed patient-specific implant and its application in pelvic tumor resection and reconstruction surgery. Comput Methods Programs Biomed. 2016; 125:66–78.


43. Wong KC, Kumta SM, Geel NV, Demol J. One-step reconstruction with a 3D-printed, biomechanically evaluated custom implant after complex pelvic tumor resection. Comput Aided Surg. 2015; 20:14–23.


44. Liang H, Ji T, Zhang Y, Wang Y, Guo W. Reconstruction with 3D-printed pelvic endoprostheses after resection of a pelvic tumour. Bone Joint J. 2017; 99:267–275.


45. Wang B, Hao Y, Pu F, Jiang W, Shao Z. Computer-aided designed, three dimensional-printed hemipelvic prosthesis for peri-acetabular malignant bone tumour. Int Orthop. 2018; 42:687–694.


46. Hughes AJ, DeBuitleir C, Soden P, et al. 3D printing aids acetabular reconstruction in complex revision hip arthroplasty. Adv Orthop. 2017; 2017:8925050.


47. Li CS, Vannabouathong C, Sprague S, Bhandari M. The use of carbon-fiber-reinforced (CFR) PEEK material in orthopedic implants: a systematic review. Clin Med Insights Arthritis Musculoskelet Disord. 2015; 8:33–45.


