Abstract
Purpose
Materials and Methods
Results
Conclusion
Figures and Tables
Fig. 1
hsa-let-7g levels in lung cancer. (A) The expression of hsa-let-7g in 20 paired normal-lung tumor samples. (B) Correlation of hsa-let-7g expression levels at different tumor stages. (C) HOXB1 expression in 20 paired normal-tumor lung samples. *p<0.05. HOXB1, homeobox B1, mRNA: messenger RNA.
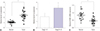
Fig. 2
Promotion of lung cancer by hsa-let-7g. (A) Expression of hsa-let-7g in A549 cells with a negative control lentivirus (LV-NC) or hsa-let-7g LV-inhibitor. (B) Proliferation in A549 cells after inhibition of has-let-7g expression. (C) Cell apoptosis in A549 cells after inhibition of has-let-7g expression. (D) Expression of hsa-let-7g in A549 cells transfected with LV-NC or hsa-let-7g LV-inhibitor. (E) Expression of hsa-let-7g in H1944 cells with a negative control lentivirus (LV-NC) or hsa-let-7g LV-inhibitor. (F) Proliferation in H1944 cells after inhibition of has-let-7g expression. (G) Cell apoptosis in H1944 cells after inhibition of has-let-7g expression. (H) Expression of hsa-let-7g in H1944 cells transfected with LV-NC or hsa-let-7g LV-inhibitor. *p<0.05.
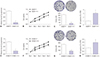
Fig. 3
Modulation of HOXB1 by hsa-let-7g. (A, B) Direct targeting of hsa-let-7g on HOXB1 was confirmed by luciferase activity assay. (C, D) Expression level of HOXB1 mRNA in A549 and H1944 cells post-transfection with hsa-let-7g mimics or mimic-NC and LV-inhibitor or negative congtrol lentivirus (LV-NC). (E, F) HOXB1 protein expression level in A549 and H1944 cells transfected with LV-NC or LV-inhibitor. *p<0.05. HOXB1, homeobox B1, mRNA: messenger RNA.
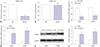
Fig. 4
Reversion in A549 cells. (A, B) The expression level of HOXB1 was significantly downregulated in A549 cells after co-transfection with LV-sh-HOXB1 and has-let-7g inhibitor, compared to cells transfected with has-let-7g inhibitor or negative congtrol lentivirus (LV-NC). (C) Proliferation increased in A549 cells after co-transfection with LV-sh-HOXB1 and LV-inhibitor, compared to LV-inhibitor. (D) Apoptosis decreased in A549 cells after co-transfection with LV-sh-HOXB1 and LV-inhibitor, compared to LV-inhibitor alone. *p<0.05. HOXB1, homeobox B1.
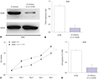
Fig. 5
Reversion in H1944 cells. (A, B) The expression level of HOXB1 was significantly downregulated in H1944 cells after co-transfection with LV-sh-HOXB1 and has-let-7g inhibitor, compared to cells transfected with has-let-7g inhibitor or negative congtrol lentivirus (LV-NC). (C) Proliferation increased in H1944 cells after co-transfection with LV-sh-HOXB1 and LV-inhibitor, compared to LV-inhibitor alone. (D) Apoptosis decreased in H1944 cells after co-transfection with LV-sh-HOXB1 and LV-inhibitor, compared to LV-inhibitor alone. *p<0.05. HOXB1, homeobox B1.
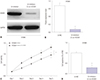
Notes
AUTHOR CONTRIBUTIONS
Conceptualization: Shengwei Ma.
Data curation: Qian Zhou and Fenghe Cui.
Formal analysis: Fenghe Cui.
Funding acquisition: Shengwei Ma.
Investigation: Kuang Xiao.
Methodology: Fenghe Cui.
Project administration: Shengwei Ma.
Resources: Kuang Xiao.
Software: Kuang Xiao.
Supervision: Shengwei Ma.
Validation: Qian Zhou.
Visualization: Qian Zhou.
Writing—original draft: Fenghe Cui.
Writing—review & editing: Shengwei Ma.
Approval of final manuscript: All authors.
References




















































