Abstract
Background
Reactive oxygen species play critical roles in homeostasis and cell signaling. Dexmedetomidine, a specific agonist of the α2-adrenoceptor, has been commonly used for sedation, and it has been reported to have a protective effect against oxidative stress. In this study, we investigated whether dexmedetomidine has a protective effect against H2O2-induced oxidative stress and the mechanism of H2O2-induced cell death in normal human fetal osteoblast (hFOB) cells.
Methods
Cells were divided into three groups: control group—cells were incubated in normoxia without dexmedetomidine, hydrogen peroxide (H2O2) group—cells were exposed to H2O2 (200 µM) for 2 h, and Dex/H2O2 group—cells were pretreated with dexmedetomidine (5 µM) for 2 h then exposed to H2O2 (200 µM) for 2 h. Cell viability and apoptosis were evaluated. Osteoblast maturation was determined by assaying bone nodular mineralization. Expression levels of bone-related proteins were determined by western blot.
Results
Cell viability was significantly decreased in the H2O2 group compared with the control group, and this effect was improved by dexmedetomidine. The Hoechst 33342 and Annexin-V FITC/PI staining revealed that dexmedetomidine effectively decreased H2O2-induced hFOB cell apoptosis. Dexmedetomidine enhanced the mineralization of hFOB cells when compared to the H2O2 group. In western blot analysis, bone-related protein was increased in the Dex/H2O2 group.
Oxidative stress is related to an imbalance between the production of reactive oxygen species (ROS) and free radicals as well as inappropriate antioxidant function. The ROS, formed by an inadequate one-electron defect of oxygen, play critical roles in homeostasis and cell signaling. Hydrogen peroxide (H2O2), a highly reactive ROS, has been commonly used to mimic in vitro oxidative stress in many types of cells [1,2].
Bone is an essential structure to support the body and has its own homeostatic activity to maintain its integrity. This activity has been called a bone remodeling process regulated by the balanced action of osteoblasts and osteoclasts [34]. Osteoblasts, which produce and release matrix proteins, have a critical role in the process of bone formation. Intracellular accumulation of ROS in osteoblasts leads to the destruction of bone homeostasis [5].
Dexmedetomidine, a specific agonist of the α2-adrenoceptor, has been commonly used in operating rooms and intensive care units for analgesia and sedation [67]. Recently, dexmedetomidine has been reported to have a protective effect on the lung, intestine, liver, and kidney against the oxidative stress that is triggered by endotoxemia or ischemia reperfusion [891011].
Thus, we aimed to investigate whether dexmedetomidine has a protective effect against H2O2-induced oxidative stress and to elucidate the mechanism of H2O2-induced cell death in normal human fetal osteoblast (hFOB) cells.
3-[4,5-dimethylthiazol-2-yl]-2,5-diphenyl tetrazolium bromide (MTT) was purchased from Sigma (St. Louis, MO, USA). Antibodies against Collagen type I (Col I), bone morphogenetic protein-2 (BMP-2), osterix, and transforming growth factor-β (TGF-β) were purchased from Abcam (Cambridge, MA, USA). GAPDH, mouse anti-rabbit IgG antibody, and rabbit anti-mouse IgG antibodies were purchased from Santa Cruz Biotechnology (Santa Cruz, CA, USA). All other chemicals and reagents were purchased from Sigma unless otherwise specified.
Human fetal osteoblast cell line (hFOB 1.19) was purchased from ATCC (Rockville, MD, USA). Cells were cultured in Dulbecco's Modified Eagle Medium: Nutrient Mixture F-12 (DMEM/F-12) with 4 mM L-glutamine, 1.5 g/L sodium bicarbonate, 4.5 g/L glucose, and 1.0 mM sodium pyruvate supplemented with 10% fetal bovine serum (FBS) and 1% penicillin-streptomycin (GIBCO-BRL, Rockville, MD, USA). Cells were incubated at 37℃ in a humidified 5% CO2–95% air incubator.
The stock of dexmedetomidine (490 µM) was kept frozen at -20℃ until use and diluted to the designated concentration with DMEM/F-12 when needed. Prior to dexmedetomidine treatment, cells were grown to about 75% confluence and then exposed to dexmedetomidine at various concentrations (0, 0.1, 1, 5, and 10 µM) for 2 h to determine the most appropriate concentration. The cells were divided into the following three groups: control group—the cells were incubated at 37℃ in a humidified atmosphere with 5% CO2 without dexmedetomidine treatment, H2O2 group—the cells were exposed to 200 µM of H2O2 for 2 h, and Dex/H2O2 group—the cells were pretreated with 5 µM of dexmedetomidine for 2 h and then exposed to 200 µM of H2O2 for 2 h.
The viability of hFOB cells was determined using the MTT assay. Cells were cultured in 96-well plates (4 × 103 cells/well). The cells were then treated with the indicated concentrations of drug. At the endpoint of treatment, 100 µl of MTT (500 mg/ml) was added to each well. The cells were incubated for 4 h at 37℃. The formazan crystals, which were formed, were then solubilized in DMSO (200 µl/well) by constant shaking for 15 min. The cell viability was measured by an ELISA reader (Tecan, Männedorf, Switzerland) at 620 nm excitatory emission wavelength.
Cells were harvested and cytocentrifuged onto a clean, glass slide. Cells were stained in 1 µg/ml Hoechst 33342 for 15 min at 37℃ in the dark and washed in phosphate buffered saline (PBS). The slides were mounted with glycerol. The samples were observed and photographed under an epifluorescence microscope (Carl Zeiss, Goettingen, Germany).
Quantification of apoptotic cells was determined by Annexin V-FITC/PI staining. The adherent cells were collected by centrifugation and then resuspended in 500 µl of 1X binding buffer. Then, they were stained with 5 µl Annexin V-FITC and propidium iodide (PI) (50 µg/ml) and incubated at room temperature for 5 min in the dark. The cells were analyzed with a CYTOMICS FC 500 flow cytometer system (Beckman Coulter, CA, USA ). The results were shown as quadrant dot plots with intact cells (Annexin V-/PI-), early apoptotic cells (Annexin V+/PI-), late apoptotic cells (Annexin V+/PI+), and necrotic cells (Annexin V-/PI+).
Mineralization of hFOB cells was determined in 24-well plates using Alizarin red S (Sigma, St. Louis, MO, USA) staining. The cells were washed once with distilled water after fixation with 4% paraformaldehyde and then stained with 2% alizarin red solution according to the manufacturer's protocol.
The cells (1.5 × 106) were washed twice in ice-cold PBS, resuspended in 200 µl ice-cold solubilizing buffer (300 mM NaCl, 50 mM Tris-Cl (pH 7.6), 0.5 % Triton X-100, 2 mM PMSF, 2 µl/ml aprotinin, and 2 µl/ml leupeptin), and incubated at 4℃ for 1 h. The lysates were centrifuged at 13,200 rpm for 30 min at 4℃. Protein concentrations of cell lysates were determined using a Bradford protein assay (Bio-Rad, Richmond, CA, USA), and 20 µg of protein was resolved by a 10% SDS/PAGE gel. The gel was transferred to polyvinylidene fluoride (PVDF) membranes (Millipore, Billerica, MA, USA). After blocking, the membranes were reacted with appropriate primary antibodies. Immunostaining with secondary antibodies was detected using SuperSignal West Femto substrate (Pierce, Rockford, IL, USA).
The effect of dexmedetomidine on hFOB cells was demonstrated over various concentrations (0, 0.1, 1, 5, and 10 µM). The viability of hFOB cells pretreated with dexmedetomidine was not changed compared to the control. Dexmedetomidine did not show any cytotoxic effect on the hFOB cells (Fig. 1A). In contrast, after treatment with a wide range of H2O2 concentrations (0, 25, 50, 100, 200, and 400 µM) for 2 h, the cell viability was significantly decreased in a dose-dependent manner (Fig. 1B). All subsequent experiments were performed with 5 µM of dexmedetomidine and 200 µM of H2O2. To compare the cell viability among the three groups, we performed another MTT assay. We pretreated with 5 µM of dexmedetomidine for 2 h and then exposed hFOB cells to 200 µM H2O2 for 2 h. As shown in Fig. 2, cell viability was markedly decreased in the H2O2 group compared to the control, while viability was significantly improved by dexmedetomidine pretreatment. As a result, we discovered that dexmedetomidine pretreatment significantly protected the hFOB cells from H2O2-induced cell death.
The mechanism of H2O2-induced cell death and protective effect of dexmedetomidine on hFOB cell were investigated by Hoechst 33342 and Annexin-V FITC/PI staining. Cells were observed under a fluorescence microscope (× 400) (Fig. 3). In the control and Dex/H2O2 groups, nuclei were lightly stained by the dye and morphological changes were not detected. In contrast, the nuclei of H2O2 treated cells presented typical apoptotic features, which are fragmented and deeply stained nuclei. We next investigated whether H2O2-induced cell death through an apoptotic mechanism. Annexin-V FITC/PI staining was used for the detection of apoptotic stages. Compared with the control group, in the H2O2 group the portion of Annexin-V(+)/PI(+) cells increased from 1.2% to 25%. However, pretreatment with dexmedetomidine 2 h prior to H2O2 treatment significantly attenuated the percentage of Annexin-V(+)/PI(+) cells to 10.0 % (P < 0.05), demonstrating the anti-apoptotic effect of dexmedetomidine (Fig. 4).
Mineralization of hFOB cells was investigated using Alizarin red S staining. This staining clearly showed that dexmedetomidine pretreatment enhanced the staining intensity of hFOB cells when compared to that of the H2O2 group (Fig. 5).
We examined the activation of bone-related protein in hFOB cells by western blotting analysis. The recruitment of osterix to the membrane occurs via a Col I-dependent mechanism; thus, Col I is essential for bone formation in vivo. The levels of Col I, BMP-2, osterix, and TGF-β were increased in the Dex/H2O2 group compared to the H2O2 group (Fig. 6).
Various external stimuli can trigger oxidative stress by toxins, ischemia, or mechanical stress. Oxidative stress has previously been regarded as an inducer of a variety of bone diseases including osteoblastic apoptosis and osteoporosis [1213]. However, the biological mechanism through which oxidative stress affects osteoblast death is still not fully understood.
The goals of this study were to determine the protective effect of dexmedetomidine on hFOB cells that have undergone H2O2-induced oxidative stress and to investigate the regulation of bone homeostasis. Our findings showed that dexmedetomidine treatment did not cause any cytotoxic effects in hFOB cells (Fig. 1A). We also utilized the cell viability assay to demonstrate the toxicity of H2O2. As a result, H2O2 treatment induced cell death in a dose-dependent manner. In particular, a concentration of more than 100 µM caused oxidative stress in hFOB cells (Fig. 1B). To investigate the effect of dexmedetomidine against H2O2-induced cell death, we made a comparison among the three distributed groups: control, H2O2, and Dex/H2O2. The viability of the H2O2 treatment group was decreased compared to that of the control. However, pretreatment with dexmedetomidine restored the cell viability that had been decreased by H2O2.
Cell staining with Hoechst confirmed that pretreatment with dexmedetomidine reduced H2O2-induced apoptosis (Fig. 3). Hoechst staining can distinguish dead cells from live cells by indicating fragmentation and condensation of chromatin, which causes the cells to brightly fluorescence [14]. We explored this process in more detail using Annexin V-FITC/PI double staining. One event in the earlier stage of apoptosis includes translocation of phosphatidylserine (PS) from the inside of the cell membrane to the surface. Annexin V has high affinity for PS, and fluorochrome-labeled Annexin V can be used for the detection of PS translocation using flow cytometry. PI is frequently used as a nuclear staining dye. The ability of PI to enter a cell is dependent on the membrane permeability; PI cannot get into live or early apoptotic cells because of the intact cell membrane. In late-stage apoptosis and necrotic cells, the intact part of plasma and nuclear membranes deteriorates, allowing PI to enter the membranes and display fluorescence [151617181920]. In the H2O2 group, the portion of Annexin-V(+)/PI(+) cells was increased. This suggested that H2O2 affects the late stage of apoptosis and dexmedetomidine has a protective effect against H2O2-induced apoptosis in hFOB cells (Fig. 4).
Osteoblasts, derived from mesenchymal stem cells, are responsible for the formation of bone extracellular matrix and are able to mineralize into bone matrix [212223]. Alizarin red S staining suggested that the bone mineralization matrix was weak in H2O2 groups. As expected, dexmedetomidine pretreatment enhanced the mineralization staining (Fig. 5). Next, we examined the recovery effect of dexmedetomidine on the expression levels of osteoblastic differentiation markers (Col I, BMP-2, osterix, and TGF-β) in hFOB cells by western blot analysis. Expression of all osteoblastic markers was decreased in the H2O2 group while dexmedetomidine enhanced their expression (Fig. 6).
In conclusion, our results suggest that H2O2-induced cell death via apoptotic signaling in hFOB cells and pretreatment with dexmedetomidine can prevent H2O2-induced oxidative stress. In addition, dexmedetomidine enhanced the activity of osteoblastic differentiation in response to oxidative injury. We propose the potential therapeutic value of dexmedetomidine in treating oxidative stress via inhibiting apoptosis and enhancing osteoblast activity. Moreover, the current investigation could be further evidence to support the antioxidant potential of dexmedetomidine in vitro.
Figures and Tables
Fig. 1
Measurement of cell viability in human fetal osteoblast (hFOB) cells. Cell viability was analyzed using the MTT assay. (A) Dexmedetomidine did not show any cytotoxic effect on the hFOB cells. (B) After a wide range of concentrations of H2O2 (0, 25, 50, 100, 200, and 400 µM) treatment for 2 h, the viability was significantly decreased in a dose-dependent manner.
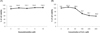
Fig. 2
Human fetal osteoblast (hFOB) cells were pretreated with dexmedetomidine (Dex, 5 µM) for 2 h and then treated with 200 µM of H2O2 for 2 h. Cell viability was analyzed using the MTT assay. The data were calculated as a percentage of the control group and expressed as the mean of at least three experiments. Cell viability was markedly decreased in the H2O2 treatment group compared to the control, but viability was significantly improved by dexmedetomidine. *P < 0.05 compared with the control group, †P < 0.05 compared with the H2O2 group.
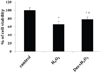
Fig. 3
Morphological changes of human fetal osteoblast (hFOB) cells in H2O2-induced apoptosis. The group preconditioned with dexmedetomidine (Dex, 5 µM) demonstrated weak nuclear condensation. Nuclei of normal control cells and Dex/H2O2 groups were lightly stained by the dye and morphological changes were not detected, while the nuclei of H2O2 treated cells presented with typical apoptotic features.
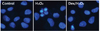
Fig. 4
Detection of apoptosis and necrosis with Annexin-V-FITC and propidium iodide (PI) staining. Every group of cells with Annexin-V and PI staining were measured by flow cytometry. Compared with the control group, the portion of Annexin-V(+)/PI(+) cells in the H2O2 group increased from 1.2% to 25%. However, pretreatment with dexmedetomidine 2 h prior to H2O2 treatment significantly attenuated the percentage of Annexin-V(+)/PI(+) cells to 10% (P < 0.05).
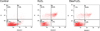
Fig. 5
Measurement of human fetal osteoblast (hFOB) cell mineralization using Alizarin red S staining. The Dex/H2O2 group showed enhanced staining compared to the H2O2 group. Dex = Dexmedetomidine.
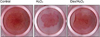
Fig. 6
Detection of protein levels using western blot analysis. Expression of type I collagen (Col I), bone morphogenetic protein (BMP)-2, osterix, and transforming growth factor-beta (TGF-β) in human fetal osteoblast (hFOB) cells. The levels of Col I, BMP-2, osterix, and TGF-β were increased in the dexmedetomidine pretreatment group compared to the H2O2 group.
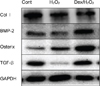
References
1. Lee D, Kook SH, Ji H, Lee SA, Choi KC, Lee KY, et al. N-acetyl cysteine inhibits H2O2-mediated reduction in the mineralization of MC3T3-E1 cell by down-regulating Nrf2/HO-1 pathway. BMB Rep. 2015; 48:636–641.


2. Song Q, Gou WL, Zhang R. FAM3A Protects HT22 Cells Against Hydrogen Peroxide-Induced Oxidative Stress Through Activation of PI3K/Akt but not MEK/ERK Pathway. Cell Physiol Biochem. 2015; 37:1431–1441.


4. Hapidin H, Rozelan D, Abdullah H, Wan Hanaffi WN, Soelaiman IN. Quercus infectoria gall extract enhanced the proliferation and activity of human fetal osteoblast cell line (hFOB 1.19). Malays J Med Sci. 2015; 22:12–22.
5. Wang F, Yin P, Lu Y, Zhou Z, Jiang C, Liu Y, et al. Cordycepin prevents oxidative stress-induced inhibition of osteogenesis. Oncotarget. 2015; 6:35496–35508.


6. Cui J, Zhao H, Yi B, Zeng J, Lu K, Ma D. Dexmedetomidine attenuates bilirubin-induced lung alveolar epithelial cell death in vitro and in vivo. Crit Care Med. 2015; 43:e356–e368.


7. Gertler R, Brown HC, Mitchell DH, Silvius EN. Dexmedetomidine: a novel sedative-analgesic agent. Proc (Bayl Univ Med Cent). 2001; 14:13–21.


8. Kip G, Celik A, Bilge M, Alkan M, Kiraz HA, Ozer A, et al. Dexmedetomidine protects from post myocardial ischaemia reperfusion lung damage in diabetic rats. Libyan J Med. 2015; 10:27828.


9. Zhang XK, Zhou XP, Zhang Q, Zhu F. The preventive effects of dexmedetomidine against intestinal ischemia-reperfusion injury in Wistar rats. Iran J Basic Med Sci. 2015; 18:604–609.
10. Sezer A, Memis D, Usta U, Sut N. The effect of dexmedetomidine on liver histopathology in a rat sepsis model: an experimental pilot study. Ulus Travma Acil Cerrahi Derg. 2010; 16:108–112.
11. Kocoglu H, Ozturk H, Ozturk H, Yilmaz F, Gulcu N. Effect of dexmedetomidine on ischemia-reperfusion injury in rat kidney: a histopathologic study. Ren Fail. 2009; 31:70–74.


12. Huang CX, Lv B, Wang Y. Protein phosphatase 2A mediates oxidative stress induced apoptosis in osteoblasts. Mediators Inflamm. 2015; 2015:804260.


13. Wang L, Zhang YG, Wang XM, Ma LF, Zhang YM. Naringin protects human adipose-derived mesenchymal stem cells against hydrogen peroxide-induced inhibition of osteogenic differentiation. Chem Biol Interact. 2015; 242:255–261.


14. Wang G, Liu C, Liu J, Liu B, Li P, Qin G, et al. Exopolysaccharide from trichoderma pseudokoningii induces the apoptosis of MCF-7 cells through an intrinsic mitochondrial pathway. Carbohydr Polym. 2016; 136:1065–1073.


15. Jung IL, Lee JH, Kang SC. A potential oral anticancer drug candidate, leaf extract, induces the apoptosis of human hepatocellular carcinoma cells. Oncol Lett. 2015; 10:1597–1604.


16. Rieger AM, Nelson KL, Konowalchuk JD, Barreda DR. Modified annexin V/propidium iodide apoptosis assay for accurate assessment of cell death. J Vis Exp. 2011; 50:e2597.


17. van Engeland M, Nieland LJ, Ramaekers FC, Schutte B, Reutelinqsperger CP. Annexin V-affinity assay: a review on an apoptosis detection system based on phosphatidylserine exposure. Cytometry. 1998; 31:1–9.


18. Wilkins RC, Kutzner BC, Truong M, Sanchez-Dardon J, McLean JR. Analysis of radiation-induced apoptosis in human lymphocytes: Flow cytometry using Annexin V and propidium iodide versus the neutral comet assay. Cytometry. 2002; 48:14–19.


19. Sawai H, Domae N. Discrimination between primary necrosis and apoptosis by necrostatin-1 in Annexin V positive/propidium iodide-negative cells. Biochem Biophys Res Commun. 2011; 411:569–573.


20. Span LF, Pennings AH, Vierwinden G, Boezeman JB, Raymakers RA, de Witte T. The dynamic process of apoptosis analyzed by flow cytometry using Annexin-V/propidium iodide and a modified in situ end labeling technique. Cytometry. 2002; 47:24–31.


21. Ren H, Ren H, Li X, Yu D, Mu S, Chen Z, et al. Effects of intermedin on proliferation, apoptosis and the expression of OPG/RANKL/M-CSF in the MC3T3-E1 osteoblast cell line. Mol Med Rep. 2015; 12:6711–6717.

