Abstract
The γδ T cells are unconventional lymphocytes that function in both innate and adaptive immune responses against various intracellular and infectious stresses. The γδ T cells can be exploited as cancer-killing effector cells since γδ TCRs recognize MHC-like molecules and growth factor receptors that are upregulated in cancer cells, and γδ T cells can differentiate into cytotoxic effector cells. However, γδ T cells may also promote tumor progression by secreting IL-17 or other cytokines. Therefore, it is essential to understand how the differentiation and homeostasis of γδ T cells are regulated and whether distinct γδ T cell subsets have different functions. Human γδ T cells are classified into Vδ2 and non-Vδ2 γδ T cells. The majority of Vδ2 γδ T cells are Vγ9δ2 T cells that recognize pyrophosphorylated isoprenoids generated by the dysregulated mevalonate pathway. In contrast, Vδ1 T cells expand from initially diverse TCR repertoire in patients with infectious diseases and cancers. The ligands of Vδ1 T cells are diverse and include the growth factor receptors such as endothelial protein C receptor. Both Vδ1 and Vδ2 γδ T cells are implicated to have immunotherapeutic potentials for cancers, but the detailed elucidation of the distinct characteristics of 2 populations will be required to enhance the immunotherapeutic potential of γδ T cells. Here, we summarize recent progress regarding cancer immunology of human γδ T cells, including their development, heterogeneity, and plasticity, the putative mechanisms underlying ligand recognition and activation, and their dual effects on tumor progression in the tumor microenvironment.
References
1. Vantourout P, Hayday A. Six-of-the-best: unique contributions of γδ T cells to immunology. Nat Rev Immunol. 2013; 13:88–100.


2. Adams EJ, Gu S, Luoma AM. Human gamma delta T cells: evolution and ligand recognition. Cell Immunol. 2015; 296:31–40.


3. Chien YH, Meyer C, Bonneville M. γδ T cells: first line of defense and beyond. Annu Rev Immunol. 2014; 32:121–155.


4. Stolk D, van der Vliet HJ, de Gruijl TD, van Kooyk Y, Exley MA. Positive & negative roles of innate effector cells in controlling cancer progression. Front Immunol. 2018; 9:1990.
5. Girardi M, Oppenheim DE, Steele CR, Lewis JM, Glusac E, Filler R, Hobby P, Sutton B, Tigelaar RE, Hayday AC. Regulation of cutaneous malignancy by gammadelta T cells. Science. 2001; 294:605–609.
6. Mishra R, Chen AT, Welsh RM, Szomolanyi-Tsuda E. NK cells and gammadelta T cells mediate resistance to polyomavirus-induced tumors. PLoS Pathog. 2010; 6:e1000924.
7. Zhao Y, Niu C, Cui J. Gamma-delta (γδ) T cells: friend or foe in cancer development? J Transl Med. 2018; 16:3.
8. Barrow AD, Martin CJ, Colonna M. The natural cytotoxicity receptors in health and disease. Front Immunol. 2019; 10:909.


9. Wrobel P, Shojaei H, Schittek B, Gieseler F, Wollenberg B, Kalthoff H, Kabelitz D, Wesch D. Lysis of a broad range of epithelial tumour cells by human gamma delta T cells: involvement of NKG2D ligands and T-cell receptor- versus NKG2D-dependent recognition. Scand J Immunol. 2007; 66:320–328.


10. Braza MS, Klein B. Anti-tumour immunotherapy with Vγ9Vδ2 T lymphocytes: from the bench to the bedside. Br J Haematol. 2013; 160:123–132.


11. Hannani D, Ma Y, Yamazaki T, Déchanet-Merville J, Kroemer G, Zitvogel L. Harnessing γδ T cells in anticancer immunotherapy. Trends Immunol. 2012; 33:199–206.


12. Kunzmann V, Smetak M, Kimmel B, Weigang-Koehler K, Goebeler M, Birkmann J, Becker J, Schmidt-Wolf IG, Einsele H, Wilhelm M. Tumor-promoting versus tumor-antagonizing roles of γδ T cells in cancer immunotherapy: results from a prospective phase I/II trial. J Immunother. 2012; 35:205–213.


13. Nicol AJ, Tokuyama H, Mattarollo SR, Hagi T, Suzuki K, Yokokawa K, Nieda M. Clinical evaluation of autologous gamma delta T cell-based immunotherapy for metastatic solid tumours. Br J Cancer. 2011; 105:778–786.


14. Rei M, Pennington DJ, Silva-Santos B. The emerging protumor role of γδ T lymphocytes: implications for cancer immunotherapy. Cancer Res. 2015; 75:798–802.


15. Fournié JJ, Sicard H, Poupot M, Bezombes C, Blanc A, Romagné F, Ysebaert L, Laurent G. What lessons can be learned from γδ T cell-based cancer immunotherapy trials? Cell Mol Immunol. 2013; 10:35–41.
16. Lo Presti E, Pizzolato G, Gulotta E, Cocorullo G, Gulotta G, Dieli F, Meraviglia S. Current advances in γδ T cell-based tumor immunotherapy. Front Immunol. 2017; 8:1401.


17. Xiang Z, Tu W. Dual face of Vγ9Vδ2-T cells in tumor immunology: anti- versus pro-tumoral activities. Front Immunol. 2017; 8:1041.


18. Turchinovich G, Pennington DJ. T cell receptor signalling in γδ cell development: strength isn't everything. Trends Immunol. 2011; 32:567–573.


19. Baker JE, Cado D, Raulet DH. Developmentally programmed rearrangement of T cell receptor Vgamma genes is controlled by sequences immediately upstream of the Vgamma genes. Immunity. 1998; 9:159–168.


20. Chodaczek G, Papanna V, Zal MA, Zal T. Body-barrier surveillance by epidermal γδ TCRs. Nat Immunol. 2012; 13:272–282.


21. Boyden LM, Lewis JM, Barbee SD, Bas A, Girardi M, Hayday AC, Tigelaar RE, Lifton RP. Skint1, the prototype of a newly identified immunoglobulin superfamily gene cluster, positively selects epidermal gammadelta T cells. Nat Genet. 2008; 40:656–662.


22. Bonneville M, O'Brien RL, Born WK. Gammadelta T cell effector functions: a blend of innate programming and acquired plasticity. Nat Rev Immunol. 2010; 10:467–478.


23. O'Brien RL, Born WK. Gammadelta T cell subsets: a link between TCR and function? Semin Immunol. 2010; 22:193–198.
24. Vermijlen D, Prinz I. Ontogeny of innate T lymphocytes – some innate lymphocytes are more innate than others. Front Immunol. 2014; 5:486.


25. Lanier LL, Weiss A. Presence of Ti (WT31) negative T lymphocytes in normal blood and thymus. Nature. 1986; 324:268–270.


26. Morita CT, Parker CM, Brenner MB, Band H. TCR usage and functional capabilities of human gamma delta T cells at birth. J Immunol. 1994; 153:3979–3988.
27. Vermijlen D, Brouwer M, Donner C, Liesnard C, Tackoen M, Van Rysselberge M, Twité N, Goldman M, Marchant A, Willems F. Human cytomegalovirus elicits fetal gammadelta T cell responses in utero. J Exp Med. 2010; 207:807–821.
28. Parker CM, Groh V, Band H, Porcelli SA, Morita C, Fabbi M, Glass D, Strominger JL, Brenner MB. Evidence for extrathymic changes in the T cell receptor gamma/delta repertoire. J Exp Med. 1990; 171:1597–1612.


29. Cairo C, Mancino G, Cappelli G, Pauza CD, Galli E, Brunetti E, Colizzi V. Vdelta2 T-lymphocyte responses in cord blood samples from Italy and Côte d'Ivoire. Immunology. 2008; 124:380–387.


30. Moens E, Brouwer M, Dimova T, Goldman M, Willems F, Vermijlen D. IL-23R and TCR signaling drives the generation of neonatal Vgamma9Vdelta2 T cells expressing high levels of cytotoxic mediators and producing IFN-gamma and IL-17. J Leukoc Biol. 2011; 89:743–752.


31. De Rosa SC, Andrus JP, Perfetto SP, Mantovani JJ, Herzenberg LA, Herzenberg LA, Roederer M. Ontogeny of gamma delta T cells in humans. J Immunol. 2004; 172:1637–1645.
32. Cruz MS, Diamond A, Russell A, Jameson JM. Human αβ and γδ T cells in skin immunity and disease. Front Immunol. 2018; 9:1304.


33. Allison TJ, Winter CC, Fournié JJ, Bonneville M, Garboczi DN. Structure of a human gammadelta T-cell antigen receptor. Nature. 2001; 411:820–824.
34. Legut M, Cole DK, Sewell AK. The promise of γδ T cells and the γδ T cell receptor for cancer immunotherapy. Cell Mol Immunol. 2015; 12:656–668.


35. Davey MS, Willcox CR, Baker AT, Hunter S, Willcox BE. Recasting human Vδ1 lymphocytes in an adaptive role. Trends Immunol. 2018; 39:446–459.


36. Pizzolato G, Kaminski H, Tosolini M, Franchini DM, Pont F, Martins F, Valle C, Labourdette D, Cadot S, Quillet-Mary A, et al. Single-cell RNA sequencing unveils the shared and the distinct cytotoxic hallmarks of human TCRVδ1 and TCRVδ2 γδ T lymphocytes. Proc Natl Acad Sci U S A. 2019; 116:11906–11915.


37. Kazen AR, Adams EJ. Evolution of the V, D, and J gene segments used in the primate gammadelta T-cell receptor reveals a dichotomy of conservation and diversity. Proc Natl Acad Sci U S A. 2011; 108:E332–E340.
38. Vavassori S, Kumar A, Wan GS, Ramanjaneyulu GS, Cavallari M, El Daker S, Beddoe T, Theodossis A, Williams NK, Gostick E, et al. Butyrophilin 3A1 binds phosphorylated antigens and stimulates human γδ T cells. Nat Immunol. 2013; 14:908–916.


39. Simões AE, Di Lorenzo B, Silva-Santos B. Molecular determinants of target cell recognition by human γδ T cells. Front Immunol. 2018; 9:929.


40. Hayes SM, Love PE. Stoichiometry of the murine gammadelta T cell receptor. J Exp Med. 2006; 203:47–52.
41. Malissen M, Gillet A, Ardouin L, Bouvier G, Trucy J, Ferrier P, Vivier E, Malissen B. Altered T cell development in mice with a targeted mutation of the CD3-epsilon gene. EMBO J. 1995; 14:4641–4653.


42. Dave VP, Cao Z, Browne C, Alarcon B, Fernandez-Miguel G, Lafaille J, de la Hera A, Tonegawa S, Kappes DJ. CD3 delta deficiency arrests development of the alpha beta but not the gamma delta T cell lineage. EMBO J. 1997; 16:1360–1370.


43. Haks MC, Krimpenfort P, Borst J, Kruisbeek AM. The CD3gamma chain is essential for development of both the TCRalphabeta and TCRgammadelta lineages. EMBO J. 1998; 17:1871–1882.


44. Hayes SM, Love PE. Distinct structure and signaling potential of the gamma delta TCR complex. Immunity. 2002; 16:827–838.


45. Siegers GM, Swamy M, Fernández-Malavé E, Minguet S, Rathmann S, Guardo AC, Pérez-Flores V, Regueiro JR, Alarcón B, Fisch P, et al. Different composition of the human and the mouse gammadelta T cell receptor explains different phenotypes of CD3gamma and CD3delta immunodeficiencies. J Exp Med. 2007; 204:2537–2544.


46. Recio MJ, Moreno-Pelayo MA, Kiliç SS, Guardo AC, Sanal O, Allende LM, Pérez-Flores V, Mencía A, Modamio-Høybjør S, Seoane E, et al. Differential biological role of CD3 chains revealed by human immunodeficiencies. J Immunol. 2007; 178:2556–2564.


47. Jensen KD, Su X, Shin S, Li L, Youssef S, Yamasaki S, Steinman L, Saito T, Locksley RM, Davis MM, et al. Thymic selection determines gammadelta T cell effector fate: antigen-naive cells make interleukin-17 and antigen-experienced cells make interferon gamma. Immunity. 2008; 29:90–100.


48. Taghon T, Yui MA, Pant R, Diamond RA, Rothenberg EV. Developmental and molecular characterization of emerging beta- and gammadelta-selected pre-T cells in the adult mouse thymus. Immunity. 2006; 24:53–64.


49. Lafont V, Sanchez F, Laprevotte E, Michaud HA, Gros L, Eliaou JF, Bonnefoy N. Plasticity of γδ T cells: impact on the anti-tumor response. Front Immunol. 2014; 5:622.


51. Parham P, Moffett A. Variable NK cell receptors and their MHC class I ligands in immunity, reproduction and human evolution. Nat Rev Immunol. 2013; 13:133–144.


52. Wensveen FM, Jelenč ićV, Polić B. NKG2D: a master regulator of immune cell responsiveness. Front Immunol. 2018; 9:441.


53. Ribot JC, debarros A, Silva-Santos B. Searching for “signal 2”: costimulation requirements of γδ T cells. Cell Mol Life Sci. 2011; 68:2345–2355.
54. Testi R, Lanier LL. Functional expression of CD28 on T cell antigen receptor gamma/delta-bearing T lymphocytes. Eur J Immunol. 1989; 19:185–188.


55. Penninger JM, Timms E, Shahinian A, Jezo-Bremond A, Nishina H, Ionescu J, Hedrick SM, Mak TW. Alloreactive gamma delta thymocytes utilize distinct costimulatory signals from peripheral T cells. J Immunol. 1995; 155:3847–3855.
56. Hamann D, Baars PA, Rep MH, Hooibrink B, Kerkhof-Garde SR, Klein MR, van Lier RA. Phenotypic and functional separation of memory and effector human CD8+ T cells. J Exp Med. 1997; 186:1407–1418.


57. De Rosa SC, Mitra DK, Watanabe N, Herzenberg LA, Herzenberg LA, Roederer M. Vdelta1 and Vdelta2 gammadelta T cells express distinct surface markers and might be developmentally distinct lineages. J Leukoc Biol. 2001; 70:518–526.
58. Seelige R, Searles S, Bui JD. Mechanisms regulating immune surveillance of cellular stress in cancer. Cell Mol Life Sci. 2018; 75:225–240.


59. Bassani B, Baci D, Gallazzi M, Poggi A, Bruno A, Mortara L. Natural killer cells as key players of tumor progression and angiogenesis: old and novel tools to divert their pro-tumor activities into potent anti-tumor effects. Cancers (Basel). 2019; 11:461.


60. O'Donnell JS, Teng MW, Smyth MJ. Cancer immunoediting and resistance to T cell-based immunotherapy. Nat Rev Clin Oncol. 2019; 16:151–167.
61. Runa F, Hamalian S, Meade K, Shisgal P, Gray PC, Kelber JA. Tumor microenvironment heterogeneity: challenges and opportunities. Curr Mol Biol Rep. 2017; 3:218–229.


62. Nakamura K, Smyth MJ. Targeting cancer-related inflammation in the era of immunotherapy. Immunol Cell Biol. 2017; 95:325–332.


63. Lo Presti E, Di Mitri R, Pizzolato G, Mocciaro F, Dieli F, Meraviglia S. γδ cells and tumor microenvironment: a helpful or a dangerous liason? J Leukoc Biol. 2018; 103:485–492.
64. Paul S, Lal G. Regulatory and effector functions of gamma-delta (γδ) T cells and their therapeutic potential in adoptive cellular therapy for cancer. Int J Cancer. 2016; 139:976–985.


66. Lee M, Park C, Woo J, Kim J, Kho I, Nam DH, Park WY, Kim YS, Kong DS, Lee HW, et al. Preferential infiltration of unique Vγ9Jγ2-Vδ2 T cells into glioblastoma multiforme. Front Immunol. 2019; 10:555.


67. Cordova A, Toia F, La Mendola C, Orlando V, Meraviglia S, Rinaldi G, Todaro M, Cicero G, Zichichi L, Donni PL, et al. Characterization of human γδ T lymphocytes infiltrating primary malignant melanomas. PLoS One. 2012; 7:e49878.


68. Deniger DC, Moyes JS, Cooper LJ. Clinical applications of gamma delta T cells with multivalent immunity. Front Immunol. 2014; 5:636.


69. Davey MS, Willcox CR, Joyce SP, Ladell K, Kasatskaya SA, McLaren JE, Hunter S, Salim M, Mohammed F, Price DA, et al. Clonal selection in the human Vδ1 T cell repertoire indicates γδ TCR-dependent adaptive immune surveillance. Nat Commun. 2017; 8:14760.


70. Sandstrom A, Peigné CM, Léger A, Crooks JE, Konczak F, Gesnel MC, Breathnach R, Bonneville M, Scotet E, Adams EJ. The intracellular B30.2 domain of butyrophilin 3A1 binds phosphoantigens to mediate activation of human Vγ9Vδ2 T cells. Immunity. 2014; 40:490–500.


71. Yang Y, Li L, Yuan L, Zhou X, Duan J, Xiao H, Cai N, Han S, Ma X, Liu W, et al. A structural change in butyrophilin upon phosphoantigen binding underlies phosphoantigen-mediated Vγ9Vδ2 T cell activation. Immunity. 2019; 50:1043–1053. e5.


72. Sebestyen Z, Scheper W, Vyborova A, Gu S, Rychnavska Z, Schiffler M, Cleven A, Chéneau C, van Noorden M, Peigné CM, et al. RhoB mediates phosphoantigen recognition by Vγ9Vδ2 T cell receptor. Cell Reports. 2016; 15:1973–1985.


73. Rigau M, Ostrouska S, Fulford TS, Johnson DN, Woods K, Ruan Z, McWilliam HE, Hudson C, Tutuka C, Wheatley AK, et al. Butyrophilin 2A1 is essential for phosphoantigen reactivity by γδ T cells. Science. 2020; 367:eaay5516.


74. Dai Y, Chen H, Mo C, Cui L, He W. Ectopically expressed human tumor biomarker MutS homologue 2 is a novel endogenous ligand that is recognized by human γδ T cells to induce innate anti-tumor/virus immunity. J Biol Chem. 2012; 287:16812–16819.


75. Tyler CJ, Doherty DG, Moser B, Eberl M. Human Vγ9/Vδ2 T cells: innate adaptors of the immune system. Cell Immunol. 2015; 296:10–21.


76. Uldrich AP, Le Nours J, Pellicci DG, Gherardin NA, McPherson KG, Lim RT, Patel O, Beddoe T, Gras S, Rossjohn J, et al. CD1d-lipid antigen recognition by the γδ TCR. Nat Immunol. 2013; 14:1137–1145.


77. Wu D, Wu P, Qiu F, Wei Q, Huang J. Human γδ T-cell subsets and their involvement in tumor immunity. Cell Mol Immunol. 2017; 14:245–253.


78. Le Nours J, Gherardin NA, Ramarathinam SH, Awad W, Wiede F, Gully BS, Khandokar Y, Praveena T, Wubben JM, Sandow JJ, et al. A class of γδ T cell receptors recognize the underside of the antigen-presenting molecule MR1. Science. 2019; 366:1522–1527.


79. Hudspeth K, Silva-Santos B, Mavilio D. Natural cytotoxicity receptors: broader expression patterns and functions in innate and adaptive immune cells. Front Immunol. 2013; 4:69.


80. Almeida AR, Correia DV, Fernandes-Platzgummer A, da Silva CL, da Silva MG, Anjos DR, Silva-Santos B. Delta one t cells for immunotherapy of chronic lymphocytic leukemia: clinical-grade expansion/differentiation and preclinical proof of concept. Clin Cancer Res. 2016; 22:5795–5804.


81. Willcox CR, Pitard V, Netzer S, Couzi L, Salim M, Silberzahn T, Moreau JF, Hayday AC, Willcox BE, Déchanet-Merville J. Cytomegalovirus and tumor stress surveillance by binding of a human γδ T cell antigen receptor to endothelial protein C receptor. Nat Immunol. 2012; 13:872–879.


82. Deniger DC, Maiti SN, Mi T, Switzer KC, Ramachandran V, Hurton LV, Ang S, Olivares S, Rabinovich BA, Huls MH, et al. Activating and propagating polyclonal gamma delta T cells with broad specificity for malignancies. Clin Cancer Res. 2014; 20:5708–5719.


83. Li Z, Xu Q, Peng H, Cheng R, Sun Z, Ye Z. IFN-γ enhances HOS and U2OS cell lines susceptibility to γδ T cell-mediated killing through the Fas/Fas ligand pathway. Int Immunopharmacol. 2011; 11:496–503.


84. Tawfik D, Groth C, Gundlach JP, Peipp M, Kabelitz D, Becker T, Oberg HH, Trauzold A, Wesch D. TRAIL-receptor 4 modulates γδ T cell-cytotoxicity toward cancer cells. Front Immunol. 2019; 10:2044.


85. Pauza CD, Liou ML, Lahusen T, Xiao L, Lapidus RG, Cairo C, Li H. Gamma delta T cell therapy for cancer: it is good to be local. Front Immunol. 2018; 9:1305.


86. Angelini DF, Borsellino G, Poupot M, Diamantini A, Poupot R, Bernardi G, Poccia F, Fournié JJ, Battistini L. FcgammaRIII discriminates between 2 subsets of Vgamma9Vdelta2 effector cells with different responses and activation pathways. Blood. 2004; 104:1801–1807.


87. Gentles AJ, Newman AM, Liu CL, Bratman SV, Feng W, Kim D, Nair VS, Xu Y, Khuong A, Hoang CD, et al. The prognostic landscape of genes and infiltrating immune cells across human cancers. Nat Med. 2015; 21:938–945.


88. Meraviglia S, Lo Presti E, Tosolini M, La Mendola C, Orlando V, Todaro M, Catalano V, Stassi G, Cicero G, Vieni S, et al. Distinctive features of tumor-infiltrating γδ T lymphocytes in human colorectal cancer. OncoImmunology. 2017; 6:e1347742.


89. Kim JS, Kim YG, Park EJ, Kim B, Lee HK, Hong JT, Kim Y, Han SB. Cell-based immunotherapy for colorectal cancer with cytokine-induced killer cells. Immune Netw. 2016; 16:99–108.


90. Park C, Kim TJ. Expansion and sub-classification of T cell-dependent antibody responses to encompass the role of innate-like T cells in antibody responses. Immune Netw. 2018; 18:e34.


91. Gu-Trantien C, Loi S, Garaud S, Equeter C, Libin M, de Wind A, Ravoet M, Le Buanec H, Sibille C, Manfouo-Foutsop G, et al. CD4+ follicular helper T cell infiltration predicts breast cancer survival. J Clin Invest. 2013; 123:2873–2892.


92. Caccamo N, La Mendola C, Orlando V, Meraviglia S, Todaro M, Stassi G, Sireci G, Fournié JJ, Dieli F. Differentiation, phenotype, and function of interleukin-17-producing human Vγ9Vδ2 T cells. Blood. 2011; 118:129–138.


94. Xia A, Zhang Y, Xu J, Yin T, Lu XJ. T cell dysfunction in cancer immunity and immunotherapy. Front Immunol. 2019; 10:1719.


95. Kim PS, Ahmed R. Features of responding T cells in cancer and chronic infection. Curr Opin Immunol. 2010; 22:223–230.


96. Meraviglia S, Caccamo N, Guggino G, Tolomeo M, Siragusa S, Stassi G, Dieli F. Optimizing tumor-reactive γδ T cells for antibody-based cancer immunotherapy. Curr Mol Med. 2010; 10:719–726.


97. Zou C, Zhao P, Xiao Z, Han X, Fu F, Fu L. γδ T cells in cancer immunotherapy. Oncotarget. 2017; 8:8900–8909.


98. Wu D, Wu P, Wu X, Ye J, Wang Z, Zhao S, Ni C, Hu G, Xu J, Han Y, et al. Ex vivo expanded human circulating Vδ1 γδ T cells exhibit favorable therapeutic potential for colon cancer. OncoImmunology. 2015; 4:e992749.
99. Siegers GM, Dhamko H, Wang XH, Mathieson AM, Kosaka Y, Felizardo TC, Medin JA, Tohda S, Schueler J, Fisch P, et al. Human Vδ1 γδ T cells expanded from peripheral blood exhibit specific cytotoxicity against B-cell chronic lymphocytic leukemia-derived cells. Cytotherapy. 2011; 13:753–764.


100. Gonçalves-Sousa N, Ribot JC, deBarros A, Correia DV, Caramalho I, Silva-Santos B. Inhibition of murine gammadelta lymphocyte expansion and effector function by regulatory alphabeta T cells is cell-contact-dependent and sensitive to GITR modulation. Eur J Immunol. 2010; 40:61–70.


101. Li W, Kubo S, Okuda A, Yamamoto H, Ueda H, Tanaka T, Nakamura H, Yamanishi H, Terada N, Okamura H. Effect of IL-18 on expansion of gammadelta T cells stimulated by zoledronate and IL-2. J Immunother. 2010; 33:287–296.


102. Li W, Yamamoto H, Kubo S, Okamura H. Modulation of innate immunity by IL-18. J Reprod Immunol. 2009; 83:101–105.


103. Van Acker HH, Anguille S, Willemen Y, Van den Bergh JM, Berneman ZN, Lion E, Smits EL, Van Tendeloo VF. Interleukin-15 enhances the proliferation, stimulatory phenotype, and antitumor effector functions of human gamma delta T cells. J Hematol Oncol. 2016; 9:101.


104. Wang X, Zhao X, Feng C, Weinstein A, Xia R, Wen W, Lv Q, Zuo S, Tang P, Yang X, et al. IL-36γ transforms the tumor microenvironment and promotes type 1 lymphocyte-mediated antitumor immune responses. Cancer Cell. 2015; 28:296–306.


105. Thedrez A, Harly C, Morice A, Salot S, Bonneville M, Scotet E. IL-21-mediated potentiation of antitumor cytolytic and proinflammatory responses of human V gamma 9V delta 2 T cells for adoptive immunotherapy. J Immunol. 2009; 182:3423–3431.


106. Chen J, Niu H, He W, Ba D. Antitumor activity of expanded human tumor-infiltrating gammadelta T lymphocytes. Int Arch Allergy Immunol. 2001; 125:256–263.


107. Choudhary A, Davodeau F, Moreau A, Peyrat MA, Bonneville M, Jotereau F. Selective lysis of autologous tumor cells by recurrent gamma delta tumor-infiltrating lymphocytes from renal carcinoma. J Immunol. 1995; 154:3932–3940.
108. Couzi L, Levaillant Y, Jamai A, Pitard V, Lassalle R, Martin K, Garrigue I, Hawchar O, Siberchicot F, Moore N, et al. Cytomegalovirus-induced gammadelta T cells associate with reduced cancer risk after kidney transplantation. J Am Soc Nephrol. 2010; 21:181–188.


109. Knight A, Arnouk H, Britt W, Gillespie GY, Cloud GA, Harkins L, Su Y, Lowdell MW, Lamb LS. CMV-independent lysis of glioblastoma by ex vivo expanded/activated Vδ1+ γδ T cells. PLoS One. 2013; 8:e68729.
110. Knight A, Mackinnon S, Lowdell MW. Human Vdelta1 gamma-delta T cells exert potent specific cytotoxicity against primary multiple myeloma cells. Cytotherapy. 2012; 14:1110–1118.


111. Lança T, Costa MF, Gonçalves-Sousa N, Rei M, Grosso AR, Penido C, Silva-Santos B. Protective role of the inflammatory CCR2/CCL2 chemokine pathway through recruitment of type 1 cytotoxic γδ T lymphocytes to tumor beds. J Immunol. 2013; 190:6673–6680.


Figure 1.
Differential recruitment of Vδ1 and Vγ9δ2 γδ T cells into the tumor tissue. In blood, Vγ9δ2 γδ T cells are predominant over Vδ2 γδ T cells in healthy individuals. Most of the Vγ9δ2 γδ T cells have canonical TCRs responding to prenyl pyrophosphates that are elevated in cancer cells and are recruited into the tumor via chemokine receptors. In contrast, some clonotypes of Vδ1 γδ T cells are selected from a diverse Vδ1 TCR repertoire. Specific Vδ1 γδ T cells migrate into the tumor tissues, expand, and kill cancer cells. The tissue-resident Vδ1 γδ T cells may respond to the tissue stress and proliferate to kill cancer cells.
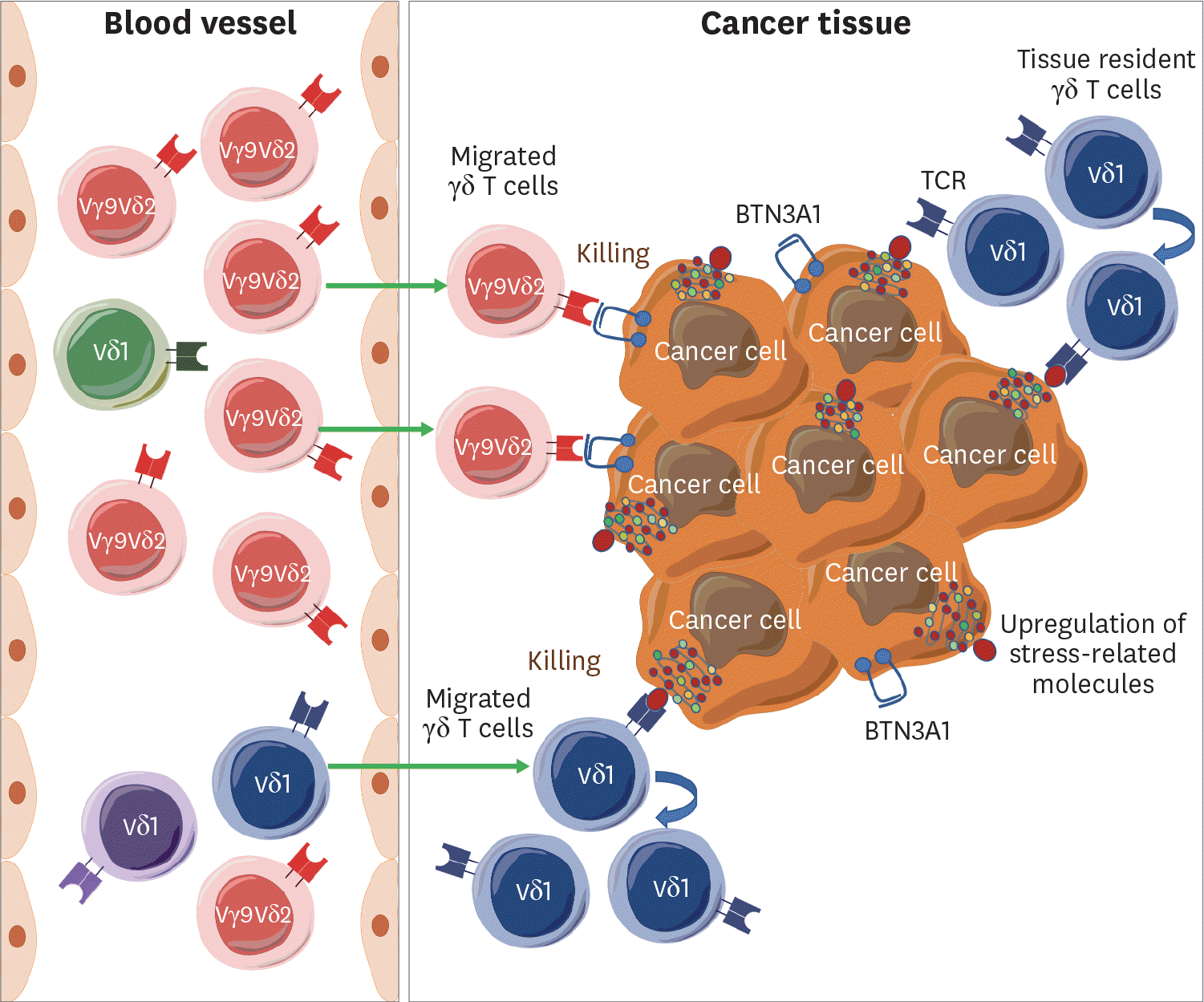