Abstract
Longitudinal imaging of murine pancreas is technically challenging due to the mechanical softness of the tissue influenced by peristalsis. Here, we report a novel pancreatic imaging window for long-term stabilized cellular-level observation of the islets in the pancreas in vivo. By spatially separating the pancreas from the bowel movement and physiologic respiration with a metal plate integrated in the imaging window, we successfully tracked the pancreatic islets up to three weeks and visualized the dumbbell-shape transformation from the single islet. This window can be a useful tool for long-term cellular-level visualization of the microstructure in the pancreas.
High-resolution intravital imaging through an abdominal imaging window enables the visualization and quantification of cell dynamics in a solid abdominal organ in vivo [123]. However, unlike the human pancreas which is a well-defined solid organ, the mouse pancreas is a soft sheet-like structure constantly affected by the bowel movement and physiologic respiration [4]. Therefore, a longitudinal imaging of microscale structures such as the islets in a microscopic resolution over an extended period of time has been technically challenging. A stabilized imaging capability through multiple imaging sessions is imperative to observe cellular-level dynamics to investigate the pancreas cell biology and pathophysiology over several weeks [5]. To overcome this hurdle, previous studies have used glue on the titanium ring to adhere it to the pancreas [12]. However, using an adhesive could induce unexpected adverse effects such as a toxic response and non-physiological microenvironmental changes as well as unintended adhesion of the pancreas to an adjacent abdominal organ [6].
Herein, we developed a novel pancreatic imaging window optimized for long-term imaging of the pancreas islets. By integrating a modified metal plate for non-invasive immobilization of soft tissue at the window, the long-term stability of pancreas was achieved without using additional adhesives on the organ surface. Using the pancreatic imaging window, we successfully tracked a single islet up to 3 weeks with cellular-resolution and visualized its dumbbell-shape morphogenesis in young mice.
All animal experiments were conducted in accordance with the standard guidelines for the care and use of laboratory animals and were approved by the Institutional Animal Care and Use Committee (IACUC) of KAIST (protocol No. KA2017-08). All mice were housed in ventilated and temperature & humidity-controlled cages under 12-hour light/12-hour dark cycle and provided with standard diet and water ad libitum. For experimental use, 4 to 12 weeks old male of C57BL/6N (OrientBio, Seongnam, Korea) and mouse insulin 1 promoter (MIP)-green fluorescent protein (GFP) (C57BL/6J strain, Tg [Ins1-EGFP] 1Hara, kindly provided by Dr. H. Kim at KAIST) mice were used in this study. All painful procedure including surgeries were conducted under anesthesia, and all efforts were made to minimize suffering.
To visualize islet through pancreatic imaging window, a custom-built video-rate laser-scanning confocal microscopy system was utilized. For multi-color fluorescence imaging, three continuous laser modules (Wavelength at 488 nm [MLD488; Cobolt, Solna, Sweden], 561 nm [Jive; Cobolt], and 640 nm [MLD640; Cobolt]) were used as excitation light source. The laser beam was transferred to the pancreas of mice through the commercial objective lens (UplanSApo, 10X, NA 0.4, Olympus, Tokyo, Japan; LUCPLFLN, 40X, NA 0.45, Olympus). Fluorescence signals from mouse pancreas were simultaneously detected by three bandpass filters (BPF1; FF02-525/50, BPF2; FF01-600/37, BPF3; FF01-685/40, Semrock, Rochester, NY, USA) and photomultiplier tubes (R9110; Hamamatsu Photonics, Hamamatsu, Japan). Using a custom-written imaging software based on Matrox Imaging Library (MIL9; Matrox, Dorval, QC, Canada), video-rate movies were displayed and recorded in real time at the frame rate of 30 Hz and frame size of 512×512 pixels. The real-time image frames were averaged over 30 frames to improve contrast and signal-to-noise ratio.
Mice were anesthetized with zoletil (30 mg/kg) and xylazine (10 mg/kg). The thermal probe was carefully inserted into the rectum for continuous monitoring of body temperature of the mouse which was maintained at 37.0℃ by using the homeothermic system (RightTemp; Kent Scientific, Torrington, CT, USA). Mice were positioned in the right lateral decubitus followed dissection of the left flank. Skin and muscle were dissected until the exposure of spleen, followed by careful exteriorization of the spleen. Pancreas connected with the spleen was overlayed on the plate of pancreas imaging window, and spleen was positioned on the opening side of the imaging window. Purse string suture was carefully done surrounding the imaging window, and the cover glass was covered on the imaging window. N-butyl cyanoacrylate glue was carefully applied to the margin of imaging window to touch with cover glass instead of the tissue.
Mice anesthesia and temperature control were performed with the same method described above. After anesthesia, pancreas imaging window of the mouse was introduced to window holder prepared in the stage of intravital microscopy setup. To visualize the vessel, 25 µg of anti-CD31 (Clone 31, 553708; BD Biosciences, Franklin Lakes, NJ, USA) conjugated with Alexa 647 (A-20006; ThermoFisher Scientific, Waltham, MA, USA) was intravenously injected 2 hours before intravital imaging session.
The novel pancreas imaging window was designed to effectively isolate the soft sheet-like pancreas from the neighboring bowel movement. Two-thirds of the base of the imaging window was supported with a thin metal plate, and the pancreatic tissue was overlaid on the plate (Fig. 1A and B, Supplementary Fig. 1). Applying the imaging window to an angle-tilting window holder on the stage of a custom-built laser-scanning video-rate confocal microscopic imaging setup [789], intravital imaging of the mouse pancreas was successfully achieved in vivo (Fig. 1C). From the real-time videos acquired at a speed of 30 frames per second, a significantly enhanced tissue imaging stability with the novel pancreas imaging window was clearly noticeable while the islets in the conventional abdominal imaging window were continuously wandering accompanied with complex non-uniform deformation due to peristalsis (Supplementary Video 1). Due to severe blurring induced by motion-artifacts, the quality of the averaged image using the abdominal imaging window was too impaired to recognize the microscale structure (Supplementary Fig. 2). Additionally, physiologic characteristics were measured to assess the detrimental effect of window implantation for 5 days and no significant decrease in body weight nor increase of blood glucose were observed (Supplementary Fig. 3).
Next, we used MIP-GFP mice [10] for simultaneous imaging of pancreatic islets and adjacent vasculature. By acquiring multiple overlapping images through the pancreas imaging window, a wide-area mosaic image was produced with a high enough resolution to distinguish individual islets and capillary (Fig. 1D). Subsequently, we verified the imaging stability of the islets using the window for longitudinal time-lapse imaging over several days. Compared to the conventional abdominal imaging window where tracking of the same islets was unfeasible, the pancreas imaging window showed a remarkable immovability of the pancreatic tissue in the gross view as well as in the microscopic view identifying the MIP-GFP expressing islets until post-implantation day 7 (Fig. 1E).
Up to post-implantation day 19, we successfully achieved the tracking of the same pancreatic islets in vivo (Fig. 2A). We next investigated the imaging of the morphogenesis of the islets in the pancreas in vivo in 4-week-old mice [1112]. With repetitive imaging from post-implantation day 1 to 5, we observed a dumbbell-shape transformation of the islets from a single islet in vivo (Fig. 2B and C).
Our study is highlighted for the specificity on pancreas as well as direct visualization of the morphogenesis of islets in situ. Technical hindrance of accessibility and stability of islets imaging has proposed low-resolution imaging or heterotopic transplantation model [1314] which has limited ability to evaluate the islet fission. In addition, their microenvironment might be different from the pancreas in situ. Our study convincingly demonstrated the finding of a previous mathematical model study [11] and ex vivo study [15], which suggested the dumbbell-shape morphogenesis was mostly derived from the fission of a single islet instead of the fusion of separate islets.
This imaging window could be a useful method for studying embryology, cell biology, and endocrinology of islets in the pancreas. Additionally, its use can be extended to observe the orthotopic pancreatic cancer model which recapitulates the tumor-microenvironment more accurately than that of the ectopic model [1617].
Figures and Tables
Fig. 1
A novel murine pancreatic window for long-term stabilized imaging of islets in vivo. (A) Schematic diagram of the pancreas arrangement in the pancreatic imaging window. (B) Photograph of the pancreatic imaging window. (C) Photograph of the application of the pancreatic imaging window in the mouse on an imaging stage with a window holder. (D) Wide-area mosaic and magnified image of the islet in the pancreas and of the vasculature in the mouse insulin 1 promoter (MIP)-green fluorescent protein (GFP) mouse. Scale bar, 1 mm (mosaic), 100 µm (magnified). (E) Comparison of the long-term tissue stability in islet imaging using the abdominal imaging window and pancreas imaging window. Each arrowhead with different colors indicates the same islets. Scale bar, 500 µm.
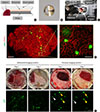
Fig. 2
Longitudinal imaging of the islets and the dumbbell-shape transformation of the islets. (A) Longitudinal imaging of the islets for 19 days in mouse insulin 1 promoter (MIP)-green fluorescent protein (GFP) mouse. Each arrowhead with different colors indicates the same islets. Scale bar, 100 µm. (B) Dumbbell-shape transformation of the islets in the pancreas. Wide-area mosaic imaging and the magnified view. Scale bar, 500 µm. (C) Magnified field of view over a period of 5 days, showing the dumbbell-shape transformation initiated from a single islet in the pancreas. Scale bar, 50 µm.
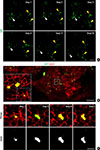
ACKNOWLEDGMENTS
This work was supported by the Global Frontier Project (NRF-2013M3A6A4044716), and the Basic Research Program (NRF-2017R1E1A1A01074190) of National Research Foundation of Korea (NRF) through funded by the Ministry of Science and ICT, Republic of Korea.
References
1. Ritsma L, Steller EJ, Beerling E, Loomans CJ, Zomer A, Gerlach C, Vrisekoop N, Seinstra D, van Gurp L, Schafer R, Raats DA, de Graaff A, Schumacher TN, de Koning EJ, Rinkes IH, Kranenburg O, van Rheenen J. Intravital microscopy through an abdominal imaging window reveals a pre-micrometastasis stage during liver metastasis. Sci Transl Med. 2012; 4:158ra145.


2. Ritsma L, Steller EJ, Ellenbroek SI, Kranenburg O, Borel Rinkes IH, van Rheenen J. Surgical implantation of an abdominal imaging window for intravital microscopy. Nat Protoc. 2013; 8:583–594.


3. Ritsma L, Ellenbroek SIJ, Zomer A, Snippert HJ, de Sauvage FJ, Simons BD, Clevers H, van Rheenen J. Intestinal crypt homeostasis revealed at single-stem-cell level by in vivo live imaging. Nature. 2014; 507:362–365.



4. Dolensek J, Rupnik MS, Stozer A. Structural similarities and differences between the human and the mouse pancreas. Islets. 2015; 7:e1024405.


6. Woodward SC, Herrmann JB, Cameron JL, Brandes G, Pulaski EJ, Leonard F. Histotoxicity of cyanoacrylate tissue adhesive in the rat. Ann Surg. 1965; 162:113–122.



7. Kim P, Puoris'haag M, Cote D, Lin CP, Yun SH. In vivo confocal and multiphoton microendoscopy. J Biomed Opt. 2008; 13:010501.


8. Kim P, Chung E, Yamashita H, Hung KE, Mizoguchi A, Kucherlapati R, Fukumura D, Jain RK, Yun SH. In vivo wide-area cellular imaging by side-view endomicroscopy. Nat Methods. 2010; 7:303–305.



9. Park I, Choe K, Seo H, Hwang Y, Song E, Ahn J, Hwan Jo Y, Kim P. Intravital imaging of a pulmonary endothelial surface layer in a murine sepsis model. Biomed Opt Express. 2018; 9:2383–2393.


10. Hara M, Wang X, Kawamura T, Bindokas VP, Dizon RF, Alcoser SY, Magnuson MA, Bell GI. Transgenic mice with green fluorescent protein-labeled pancreatic beta-cells. Am J Physiol Endocrinol Metab. 2003; 284:E177–E183.
11. Jo J, Kilimnik G, Kim A, Guo C, Periwal V, Hara M. Formation of pancreatic islets involves coordinated expansion of small islets and fission of large interconnected islet-like structures. Biophys J. 2011; 101:565–574.



12. Jo J, Hara M, Ahlgren U, Sorenson R, Periwal V. Mathematical models of pancreatic islet size distributions. Islets. 2012; 4:10–19.



13. van Gurp L, Loomans CJM, van Krieken PP, Dharmadhikari G, Jansen E, Ringnalda FCAS, Beerling E, van Rheenen J, de Koning EJP. Sequential intravital imaging reveals in vivo dynamics of pancreatic tissue transplanted under the kidney capsule in mice. Diabetologia. 2016; 59:2387–2392.



14. Lee EM, Park I, Lee YJ, You YH, Kim JW, Kim MJ, Ahn YB, Kim P, Ko SH. Effect of resveratrol treatment on graft revascularization after islet transplantation in streptozotocin-induced diabetic mice. Islets. 2018; 10:25–39.


15. Seymour PA, Bennett WR, Slack JM. Fission of pancreatic islets during postnatal growth of the mouse. J Anat. 2004; 204:103–116.



SUPPLEMENTARY MATERIALS
Supplementary materials related to this article can be found online at https://doi.org/10.4093/dmj.2018.0268.
Supplementary Fig. 1
Designs of pancreatic imaging window and its holder. Drawing with dimensions of (A) pancreatic imaging window and (B) window holder. Unit of number is millimeter.
Supplementary Fig. 2
Comparison of averaged image of pancreas between (A) abdominal imaging window and (B) pancreas imaging window. Representative averaged image of intravital pancreas imaging in abdominal imaging window and pancreatic imaging window for 3 seconds. This figure corresponds to Supplementary Video 1. Scale bar, 500 µm. MIP, mouse insulin 1 promoter.
Supplementary Fig. 3
Physiologic characteristics in pancreatic imaging window implanted mice. Measurement of (A) body weight and (B) blood glucose in pancreatic imaging window implanted mouse for 5 days, showing no significant decrease of body weight nor increase of blood glucose. Data with error bar at each time point are represented as mean±standard deviation.
Supplementary Video 1
Comparison of imaging stability between abdominal imaging window and pancreas imaging window. Representative video of real-time intravital pancreas imaging in abdominal imaging window and pancreatic imaging window, demonstrating a significantly improved stability in pancreas imaging window model. This video corresponds to Supplementary Fig. 2. Supplementary Video is available in the online version of the paper. Scale bar, 500 µm.