This article has been corrected. See "Corrigendum: Table and Text Correction. Recent Updates on Type 1 Diabetes Mellitus Management for Clinicians" in Volume 42 on page 177.
Abstract
Type 1 diabetes mellitus (T1DM) is a chronic autoimmune condition that requires life-long administration of insulin. Optimal management of T1DM entails a good knowledge and understanding of this condition both by the physician and the patient. Recent introduction of novel insulin preparations, technological advances in insulin delivery and glucose monitoring, such as continuous subcutaneous insulin infusion (CSII) and continuous glucose monitoring and improved understanding of the detrimental effects of hypoglycaemia and hyperglycaemia offer new opportunities and perspectives in T1DM management. Evidence from clinical trials suggests an important role of structured patient education. Our efforts should be aimed at improved metabolic control with concomitant reduction of hypoglycaemia. Despite recent advances, these goals are not easy to achieve and can put significant pressure on people with T1DM. The approach of physicians should therefore be maximally supportive. In this review, we provide an overview of the recent advances in T1DM management focusing on novel insulin preparations, ways of insulin administration and glucose monitoring and the role of metformin or sodium-glucose co-transporter 2 inhibitors in T1DM management. We then discuss our current understanding of the effects of hypoglycaemia on human body and strategies aimed at mitigating the risks associated with hypoglycaemia.
Type 1 diabetes mellitus (T1DM) is a chronic autoimmune condition resulting in absolute shortage of pancreatic insulin production. Regular and life-long insulin administration is therefore necessary to prevent hyperglycaemia, metabolic decompensation and life-threatening diabetic ketoacidosis (DKA). According to the International Diabetes Federation (IDF), there were approximately 425 million people living with diabetes worldwide in 2017, out of which 5% to 10% are estimated to have T1DM (42.5 to 95 million) [1]. Management of T1DM requires good understanding of this condition by patients and their physicians. At the same time, it imposes significant financial costs on health systems worldwide. Optimal management of T1DM leading to good metabolic control with prevention of micro- and macrovascular complications with concomitant avoidance of hypoglycaemia is therefore of significant social and economic importance.
Publication of the landmark study Diabetes Control and Complications Trial (DCCT) in 1993 provided clear evidence that intensive therapy consisting of insulin administration by three or more daily injections or by a pump with self-monitoring of blood glucose (SMBG) 4 times/day or more and frequent insulin dose adjustments reduced risk of microvascular complications in comparison to conventional therapy of that time (one to two insulin injections/day, daily self-monitoring of urine or blood glucose [BG] and education about diet and exercise) [2]. The incidence of severe hypoglycaemia (SH) was approximately three times higher in the intensive group, however [2]. Intensive therapy with frequent SMBG, patient education and avoidance or minimising occurrence of hypoglycaemia have since then become the pillars of modern T1DM management.
In this review, we provide an overview of the recent advances in T1DM management focusing on novel insulin preparations, ways of insulin administration and glucose monitoring and the role of metformin or sodium-glucose co-transporter 2 (SGLT2) inhibitors in T1DM management. We then discuss our current understanding of the effects of hypoglycaemia on human body and the ways how to prevent or minimise its occurrence.
Insulin administration represents the mainstay of T1DM treatment. The purpose of insulin administration is to prevent the development of DKA due to the absolute shortage of intrinsic insulin production and to maintain BG levels within the physiologic range. Insulin administration should thus ideally prevent, or at least delay development of micro- and macrovascular complications of hyperglycaemia and, at the same time, should cause as little hypoglycaemia as possible. To achieve this, pharmacokinetic and pharmacodynamic properties of administered insulin should ideally, mimic those of physiologic insulin release from pancreatic β-cells in healthy individuals in whom basal continuous insulin secretion into the portal vein together with super-added peak insulin secretions closely following the rise in plasma glucose concentration 30 to 60 minutes after eating can be observed [3]. This has proven to be a challenging task as the physiological mechanisms controlling glucose metabolism are extremely complex. Thus, current insulin formulations and modes of delivery are unable to fully reproduce the physiology of the β-cell [4]. Since the discovery of insulin in the 1920s [5] remarkable steps towards achieving this goal have been made, but there is still a long way to go. The currently available insulin preparations in the UK, as listed in the “British National Formulary (BNF)” at the time of writing of the manuscript (December 2017), together with their chemical structure and action profiles are listed in Table 1. Historically, animal insulins made from bovine or porcine pancreatic extracts were used first, since the 1970s prepared as a ‘monocomponent insulin,’ but their use has been complicated by allergic reactions caused by impurities, other immunogenicity problems and variable rates of absorption. Interestingly, there is still a minority of patients who use animal insulins at present. Their numbers are likely to decrease in the near future as the manufacturer of the Hypurin® Bovine (Wockhardt UK Ltd., Wrexham, UK) has recently announced the discontinuation of bovine insulin in the UK by the end of 2017. The production of porcine insulin is being continued, however. The amino acid sequence of insulin was reported in 1955 [6] and insulin was the first therapeutic protein to be produced by recombinant DNA technology in late 1970s [7]. Commercial production of human insulin in the early 1980s enabled production of synthetic human insulin in virtually unlimited quantities and in a cost-effective way [8]. The tendency of human soluble insulin to aggregate into dimers and hexamers in higher concentrations with resulting delay in release of insulin monomers into the bloodstream from the subcutaneous depot has important consequences for its pharmacokinetic and pharmacodynamic properties and has prompted the development of rapid-acting insulin analogues [9]. The first rapid-acting insulin analogue, insulin lispro (Humalog®; Elly Lilly Ltd., Indianapolis, IN, USA), was introduced in the USA in 1996 and the introduction of insulin aspart (Novorapid®; Novo Nordisk Ltd., Bagsværd, Denmark) and glulisine (Apidra®; Sanofi, Paris, France) followed soon after. Their pharmacokinetic and pharmacodynamic profiles show more rapid rise after injection to higher plasma insulin levels together with more rapid fall of their concentration in comparison to regular insulin [10] resulting in improved post-prandial plasma glucose (PPG) profiles [11]. Initial trials comparing the use of rapid-acting analogues versus regular insulin (combined with intermediate insulins isophane or ultralente since long-acting insulin analogues were introduced some years later) showed a significant reduction of overall rates of hypoglycaemia and SH [12], with the most prominent reduction observed in episodes at night in those with tight metabolic control (glycosylated hemoglobin [HbA1c] <7.5%) [13]. The latest step towards the goal of replicating endogenous prandial insulin secretion profiles is the introduction of faster insulin aspart (Fiasp®; Novo Nordisk Ltd.), a formulation of insulin aspart with additional L-arginine and niacinamide (vitamin B3) that promote stability and faster formation of insulin aspart monomers after subcutaneous injection [14]. In a 26-week, double-blinded, treat-to-target trial (ONSET 1), mealtime Fiasp® significantly reduced HbA1c versus insulin aspart with estimated treatment difference (ETD) −0.15% (95% confidence interval [CI], −0.23 to −0.07; P=0.0003) and also significantly reduced post-PPG increments at 1 hour (ETD, −1.18 mmol/L; 95% CI, −1.65 to −0.71; P<0.0001) and at 2 hours (ETD, −0.67 mmol/L; 95% CI, −1.29 to −0.04; P=0.0375) without increasing risk of overall hypoglycaemia [15]. BioChaparone insulin lispro (Adocia, Lyon, France) uses polysaccharides that can be modified with amino acids to facilitate its absorption into blood circulation and clinical trials of this formulation are currently in progress.
The introduction of long-acting (basal) insulin analogues reflected the drive to goal develop a ‘peakless’ continuous insulin action profile which would mimic basal insulin secretion observed in healthy individuals. The available intermediate insulins (isophane and lente/ultralente) exhibited a peak glucose lowering effect several hours into their onset of action with increased risk of hypoglycaemia, particularly at night. This was compounded by a considerable day-to-day variability of their glucose lowering action. Insulin glargine/Lantus® (Sanofi), since 2015 also produced by Elly Lilly (Abasaglar®), was first introduced in 2000 and was followed by insulin detemir/Levemir® (Novo Nordisk Ltd.) in 2005. Basal analogues were shown to have a flatter action profile and a more predictable effect, longer duration of action with resulting lower frequency of injections. This led to significantly fewer hypoglycaemic episodes in comparison to intermediate insulin although severe episodes were generally not reduced significantly, perhaps because those at greatest risk of hypoglycaemia were generally excluded from clinical trials [16]. It is noteworthy that a recent trial which compared rates of hypoglycaemia using insulin analogues (insulin aspart and levemir) to human insulin in patients at high risk of hypoglycaemia using a crossover design was able to show a significant reduction in rates of SH, particularly during the night [17]. More recently, two longer acting insulin analogues, glargine U300/Toujeo® (Sanofi) and insulin degludec/Tresiba® have been introduced. The development of glargine U300 adopts the principle that glargine at higher concentration, forms subcutaneous crystals from which insulin is absorbed more slowly. Insulin degludec utilises a glutamic acid spacer to produce both a much slower degradation into insulin monomers. The results of head to head clinical trials between these two new insulins which have half-lives of over 24 hours are awaited. However, one recent clinical trial which adopted a double blind, randomized, cross-over design showed major reductions in SH among individuals with T1DM particularly at night (SWITCH 1) [18] and similar results were observed in an identically designed trial in people with type 2 diabetes mellitus (T2DM)-SWITCH 2 trial [19].
The effect of insulin analogues on HbA1c reduction, as compared with regular and intermediate insulins, in the case of T1DM (in T2DM as well) has been very modest, if any, with significant number of trials showing no improvement at all [20]. However, this in part is due to a treat-to-target design mandated by the regulatory authorities in insulin dosing algorithms are used in both arms to achieve comparable fasting glucose targets are patients. Nevertheless, the higher cost of insulin analogues, has contributed to an ongoing debate as their overall cost-effectiveness in diabetes management [2021]. It is increasingly accepted; however, that the use of insulin analogues in a form of a multiple daily insulin (MDI) regimen or as a continuous subcutaneous insulin infusion (CSII) is the optimal management strategy in people with T1DM [202223].
Currently, the most widespread mode of insulin delivery is via a subcutaneous injection by the help of an insulin pens and single-use thin needles. For this purpose, manufacturers either provide pre-filled single-use disposable pens or insulin pens with replaceable cartridges. The use of disposable syringes and insulin vials has been nearly completely abolished across the Europe and also in North America.
CSII, also known as an ‘insulin pump’ was introduced into the clinical practice in the early 1980s. CSII uses a portable electromechanical pump continuously infusing rapid-acting insulin into the subcutaneous tissue at pre-selected rates (‘basal rate’) mimicking the basal insulin secretion in non-diabetic individuals with patient-activated insulin boluses delivered with meals. In a meta-analysis of 12 randomised controlled trials (RCTs) comparing CSII versus MDI regimens, CSII was shown to reduce mean HbA1c by approximately 0.51% as well as BG variability and this was achieved by an average reduction of total insulin dose of 14% [24]. Another meta-analysis of 22 trials which included patients with high baseline rates of SH showed that occurrence of SH was reduced more than four-fold during CSII in comparison with MDI (rate ratio of 4.19; 95% CI, 2.86 to 6.13) with the greatest reduction seen in those with the highest initial rates of SH [25]. It has to be mentioned; however, that none of the RCTs reporting benefit included long-acting insulin analogues in the MDI arm. The benefits of CSII in relation to the reduction of HbA1c and rates of hypoglycaemia seem to persist over several years as a recent study has indicated [26]. The above benefits of CSII have to be matched against significantly higher costs in comparison to MDI regimens and hence most health systems recommend the use of CSII only once adequate metabolic control can't be achieved with MDI regimen or in cases of troublesome SH. On the other hand, the HypoCOMPaSS (Comparison of Optimised MDI versus Pumps with or without Sensors in Severe Hypoglycemia) trial with a 2×2 factorial design examined the effect of CSII versus MDI regimen (using long and rapid-acting insulin analogues) and continuous glucose monitoring (CGM) versus SMBG on the restoration of hypoglycaemia awareness and decrease of SH as well as overall biochemical hypoglycaemia [27]. All participants received comparable education, support and identical therapeutic targets aiming at rigorous avoidance of hypoglycaemia. After 24 weeks, more than 10-fold decrease in incidence of SH, decrease in occurrence of overall biochemical hypoglycaemia without deterioration in HbA1c and improvement in hypoglycaemia awareness were observed. Interestingly, no statistically significant differences in rates of SH or awareness improvement were observed comparing CSII versus MDI or CGM versus SMBG. This clearly highlights the essential role of education and patient support in management of SH and impaired awareness of hypoglycaemia (IAH) [27].
Metformin, a biguanide commonly used in management of T2DM has been used off-label in people with T1DM in an attempt to reduce effective insulin dose requirements and associated weight gain [28]. The current National Institute for Health and Care Excellence (NICE) guidelines for management of T1DM recommend considering metformin in adults with T1DM and a body mass index (BMI) ≥25 kg/m2 who ‘want to improve glucose control while minimising their effective insulin dose’ [22]. American Diabetes Association (ADA) also recommend adding metformin to insulin therapy which may reduce insulin requirements and improve metabolic control in overweight or obese people with poorly controlled T1DM [23]. It is noteworthy that these recommendations are based on relatively limited amount of evidence. A meta-analysis undertaken by Lund et al. [29] in 2010 (only five trials included) reported a significant reduction of insulin dose (6.6 units/day, P<0.001), but changes in HbA1c were inconsistent. A recent trial reported that adding metformin (1g twice daily) to titrated insulin therapy (target HbA1c 53 mmol/mol) in people with T1DM aged 40 years or older and with three or more cardiovascular (CV) risk factors for duration of 3 years resulted in significant reduction of progression of caroT1DM artery intima-media thickness, a surrogate marker for progression of atherosclerosis (P=0.0093) [30]. This approach also led to significant falls in low density lipoprotein cholesterol (−0.13 mmol/L, P=0.012), sustained weight reduction (−1.17 kg, P<0.0001) and lower HbA1c at 3 months by 2.6 mmol/mol (P<0.001), although this was not sustained [30]. These data while interesting, are arguably insufficient to recommend a change in practice, in the absence of a large trial which includes outcomes of more relevance to patients. This leaves clinicians to use their clinical judgement based on currently existing evidence when considering initiation of metformin in people with T1DM.
Inhibitors of SGLT2 are the newest class of drugs approved for treatment of T2DM [31]. SGLT2 inhibition reduces renal tubule glucose reabsorption by promoting urinary glucose excretion. The three currently available SGLT2 inhibitors—dapagliflozin, empagliflozin, and canagliflozin result in improvements of metabolic control and were also shown to cause weight and blood pressure reduction [31]. In addition, two large CV outcome trials have shown their positive effects on hard CV endpoints—EMPA-REG (Empagliflozin Cardiovascular Outcome Event Trial in Type 2 Diabetes Mellitus Patients) for empagliflozin [32] and the CANVAS (Canagliflozin Cardiovascular Assessment Study) trial for canagliflozin [33] but in patients with T2DM at increased CV risk. Clearly, the insulin-independent mode of action of SGLT2 inhibitors might also benefit individuals with T1DM, but the initial excitement was set back by the reports of euglycaemic DKA both in people with T2DM and T1DM [34]. In the recently published phase 3 trial DEPICT-1 (Dapagliflozin Evaluation in Patients with Inadequately Controlled Type 1 Diabetes), dapagliflozin, when given as an oral adjunct to adjustable insulin in patients with inadequately-controlled T1DM demonstrated significant reductions from baseline in HbA1c, body weight, and also lowered total daily insulin dose at 24 weeks at both the 5 and 10 mg dose compared to placebo whilst the incidence of hypoglycaemia, SH and adjudicated ketoacidosis did not differ significantly between both treatment arms and the placebo arm [35]. Participants in this study were provided with a combined BG and ketone meter and were asked to measure BG four times a day and ketonaemia whenever BG readings were consistently elevated. Participants were also instructed to reduce insulin doses by no more than 20% on initiation of the study medication and then subsequently to up-titrate insulin doses back to baseline [36]. A 26-week extension phase of this trial is currently in progress. Sotagliflozin is a novel inhibitor of SGLT1 and SGLT2. SGLT1 inhibition in the intestine reduces glucose absorption in proximal intestine resulting in significant blunting and delay of post-prandial hyperglycaemia [37]. Results of a recently published phase 3 double-blinded RCT show that sotagliflozin versus placebo added to insulin therapy for 24 weeks in people with T1DM resulted in significantly increased number of participants who achieved HbA1c levels <7.0% (28.6% vs. 15.2%, P<0.001) with similar rates of SH in both groups [37]. Sotagliflozin use versus placebo was also linked with significantly higher reduction of weight (−2.98 kg), systolic blood pressure (−3.5 mm Hg) and mean daily bolus of insulin (−2.8 units/day) (P<0.002 for all comparisons). The rate of DKA was higher in the sotagliflozin group (3.0% vs. 0.6%). Mean baseline BMI in the sotagliflozin group was 28.29±5.13 and in the placebo group 28.10±5.18 [37].
These results suggest that SGLT2/SGLT1 inhibitors may be an appropriate adjunct to insulin in people with T1DM who monitor BG regularly, have the availability to monitor ketonaemia in home environment and have good understanding of early signs of ketoacidosis. The risk of DKA will need to be balanced by the potential benefits. More research is required, particularly as there is emerging evidence that these agents may provide protection from long-term renal disease.
BG monitoring requires individuals with diabetes to apply finger-prick capillary samples to reagent test strips which are then inserted into portable meters (SMBG) and was originally introduced into clinical practice in 1978 [38]. Initially indicated for use in pregnancy, SMBG spread widely and has become an essential component of flexible intensified insulin therapy pioneered by Muhlhauser et al. [39] in the early 1980s. The approach was reinforced by the publication of the DCCT trial in 1993 [2]. SMBG is now an integral part of modern T1DM management included as part of structured education courses, such as dose adjustment for normal eating (DAFNE) [40] and others. SMBG is usually undertaken pre-meal and before bed to guide insulin dosing based on carbohydrate intake. In addition to detecting hypo- or hyperglycaemia, serial SMBG readings inform trends and fluctuations in BG and help individuals to achieve glucose targets and optimise metabolic control. All modern BG meters are plasma-calibrated (BG readings from plasma are up to 14% higher compared to whole-blood readreadings due to lower glucose concentrations in erythrocytes), use a minimal amount of capillary blood (<1 µL) and have a memory with a capacity to store several hundreds of BG readings. Most BG meter manufacturers provide software enabling BG data download into a PC or mobile device where a more detailed statistical analysis can be performed as detailed below. There is evidence available suggesting a strong association between increased frequency of SMBG and improved metabolic control, however the available information needs to be used effectively in diabetes management as the act of performing SMBG alone is not directly related to improved HbA1c [41]. Some new BG meters can also measure concentrations of plasma ketone bodies (β-hydroxybutyrate). Early detection of increased ketonaemia by the patient outside the hospital, for example in cases of intercurrent illness, can lead to timely treatment preventing the development of full-blown DKA and need for hospital admissions.
Systems which monitor glucose every few minutes (CGM) have moved from the research to the clinical arena in the last decades [42]. CGM use has steadily increased as accuracy and costs have fallen although high running costs and resulting reimbursement issues have limited its widespread use in in most healthcare systems. CGM devices monitor interstitial, rather than plasma glucose levels (IG) in regular closely spaced time intervals, mostly every 5 to 10 minutes. IG data is then transmitted from a sensor to a reader with a monitor where it can be accessed in a graphical or numerical format. In clinical practice, real-time CGM usually displays real-time IG readings together with trends, rate of change and offer an option to set alarms for high and low readings at pre-set thresholds. This permits immediate action in cases of impeding hypo- or hyperglycaemia. The physiological lag time of 4 to 10 minutes between changes in plasma glucose and IG concentrations [43] is particularly relevant when BG is changing rapidly, for example during hypoglycaemia. RCTs have shown modest benefits of CGM versus SMBG in relation to HbA1c reduction in participants who were generally experienced pump users [4445]. Two recently published trials have also shown a clear benefit of CGM in this aspect in those using MDI. The open-label crossover GOLD trial examined the effect of 26-week long use of CGM versus SMBG in 161 participants with T1DM on MDI (mean baseline HbA1c 8.6% or 70 mmol/mol) and found a significant fall in HbA1c linked with the use of CGM to 7.9% (63 mmol/mol) compared to a fall in the SMBG arm to 8.35% (68 mmol/mol) giving a mean difference of −0.4% (95% CI, −0.57% to −0.29%) (4.7 mmol/mol; 95% CI, −6.3 to −3.1 mmol/mol; P<0.001) [46]. Similarly, the DIAMOND (Multiple Daily Injections and Continuous Glucose Monitoring in Diabetes) study reported a mean HbA1c fall from baseline of 1.1% at 12 weeks and 1.0% at 24 weeks of CGM use, significantly more than the difference of 0.5% at 12 and 0.4% at 24 weeks seen in the control (SMBG) group (repeated measures model P<0.001) [47]. These trials also reported positive effect of CGM on the time spent in hypoglycaemia and prevention of hypoglycaemic episodes, and, in case of the GOLD study, also on incidence of SH [454647]. The important prerequisite for the beneficial effects of CGM to manifest is good compliance with its use, in the above mentioned studies it neared 90% of the time.
Sensor-augmented pump (SAP) therapy refers to a combination of a CGM and a CSII therapy which are integrated in a way that the insulin pump acts as a receiver of the CGM data sent to it wirelessly from the sensor by a transmitter. In SAP, CGM readings do not influence the rate of insulin delivery by the insulin pump per se and it is up to the user to adjust insulin rates based on the available CGM data. Several studies have reported a clear benefit of SAP versus CSII alone or MDI (with SMBG) on metabolic control, but this required good sensor compliance, at least 70% of the time. The RealTrend trial examined the effect of SAP versus CSII alone treatment modalities in patients with suboptimal metabolic control (HbA1c ≥8.0%) who have previously been on MDI regimen [48]. After 6 months, HbA1c improved significantly in both groups (SAP group −0.81%±1.09%, P<0.001; CSII group −0.57%±0.94%, P<0.001), with no significant difference between the groups. However, when only those with sensor compliance of ≥70% in the SAP group were considered, HbA1c improvement was significantly greater in the SAP group (P=0.004) (SAP group −0.96%±0.93%, P<0.001; CSII group −0.55%±0.93%, P< 0.001) [48].
SAP systems with the low glucose suspend (LGS) feature, like the MiniMed™ Paradigm Veo™ (Medtronic Inc., Watford, UK), allow for a temporary suspension of insulin delivery (usually for up to 2 hours) when IG level falls below a pre-defined threshold value. The ASPIRE (Automation to Simulate Pancreatic Insulin Response) trial showed a clear benefit of SAP with LGS versus SAP alone in reduction of nocturnal hypoglycaemia. Nocturnal hypoglycaemic events occurred 31.8% less frequently and the mean area under the curve for nocturnal hypoglycaemia was 37.5% lower in the LGS group (both P<0.001) with comparable changes in HbA1c. Rates of SH were also reduced [49]. In a RCT published by Ly et al. [50], use of SAP with LGS for 6 months resulted in decrease of SH from 175 events per 100 patient-months to 35 and in the SAP only group the decrease in the event rate was from 28 to 16. Despite randomisation, significant difference in the baseline prevalence of hypoglycaemia between the study groups existed in this study. Nevertheless, the results are certainly promising.
The MiniMed™ 640G Insulin Pump System (Medtronic Inc.) with the SmartGuard™ technology is a next-generation LGS system enabling suspension of insulin delivery in response to a predicted, rather than actual hypoglycaemic level. Insulin suspension is activated if IG is predicted to reach or fall below a pre-set low limit within next 30 minutes. Insulin delivery will automatically re-start 30 minutes later if IG at that time is above the pre-set limit and is predicted to remain above the low limit for subsequent 30 minutes. Initial observational studies indicate that this system could prevent a substantial number of hypoglycaemic episodes: 2,322 suspend-before-low events (2.1 per subject-day) in a group of 40 adults with T1DM were encountered and in 1,930 events (83.1%) subsequent IG levels did not reach the pre-set low limit meaning that hypoglycaemic episode was avoided, and this device seems to be well tolerated by its users [51]. The last step towards the ‘artificial pancreas’ so far has been made with the introduction of the first hybrid closed-loop system on the US market in 2017, the MiniMed™ 670G Insulin Pump System (Medtronic Inc.). This system, on top of the above listed features, combines the user-delivered pre-meal insulin boluses with the ability to automatically adjust basal insulin delivery every 5 minutes based on the IG readings [52]. Other more advanced closed-loop systems are being developed at present [53].
Another important development on the CGM market is the introduction of a first flash glucose monitoring system, the FreeStyle® Libre (FL; Abbott Diabetes Care, Maidenhead, UK). FL consists of a subcutaneous button-like sensor that measures IG every minute with a storage capacity of 8 hours of data and of a reader with a monitor. Swiping the reader over the sensor collects IG data at the moment of scanning plus up to 8 hours of prior readings every 15 minutes. Hence in order to get a continuous IG trace, the user is asked to swipe the reader at least once in every 8-hour time interval. FL is factory-calibrated and running costs are approximately half of those of current CGM devices. On the down side, given its character, it provides IG trends but no hypo/hyperglycaemia alarms can be set. The accuracy of FL is comparable to currently used standard CGM devices, with mean absolute relative difference of 11.4%, as reported by the manufacturer and also in real-life settings with most accurate results measured with a sensor inserted in the upper arm [54]. FL has been shown to reduce the time spent in hypoglycaemia by 38% in a group of well controlled patients with T1DM (HbA1c ≤7.5%) and has been well tolerated by its users thus far [5556]. It therefore represents an attractive alternative to ‘classic’ CGM systems in certain, but not all clinical scenarios in T1DM management as well as in other indications for CGM use [57]. Table 2 provides a summary of the main features of currently available devices for BG/IG monitoring.
With increasing amounts of data available from frequent SMBG, but mainly with the introduction and wider use of CGM systems, a more complex and clinically relevant analysis of glucose readings became possible. Indices such as glycaemic variability (assessed by coefficient of variation as a primary, or standard deviation [SD] as a secondary metric), time in range (time spent in an individual's target glucose range) or ‘time in level 1 or level 2 of hypo- or hyperglycaemia’ provide valuable additional information about an individual's BG control well beyond the HbA1c and the recently published international consensus on use of CGM provides guidance of their reporting [58].
Iatrogenic hypoglycaemia continues to remain a major barrier in achieving tight glycaemic control in T1DM [59]. The precise definition of hypoglycaemia is, however, debatable. According to an ADA working group, symptomatic hypoglycaemia is defined as typical symptoms of hypoglycaemia with a glucose measurement of <3.9 mmol/L, whereas SH is defined by the need for assistance from another person during an episode [60]. There is no clear global consensus on single definition of hypoglycaemia leading to heterogeneity in reporting in both observational studies and in clinical trials. This inconsistency makes it challenging to directly compare different populations. Recently, the International Hypoglycaemia Study Group has proposed an additional definition of hypoglycaemia with glucose concentrations <3.0 mmol/L [61]. It is hoped that this definition proposed jointly by the ADA and European Association for the Study of Diabetes will be adopted broadly allowing for meaningful comparisons between studies investigating the epidemiology of hypoglycaemia and interventions aimed at reducing hypoglycaemia.
Studies investigating the epidemiology of hypoglycaemia in T1DM are compromised by several limitations. Most studies have focused on retrospective patient reported events which are prone to recall bias. Where data on hypoglycaemia occurrence has been collected prospectively, this has been over brief periods of time typically between 30 to 90 days introducing the possibility of sampling error. Finally, the populations studied have been prone to selection bias as those particularly prone to hypoglycaemia were likely to participate. One of the most reliable prospective population-based studies has estimated the incidence of SH in T1DM at 1.15 events per person per year [62].
It is recognised, that repeated episodes of iatrogenic hypoglycaemia attenuate the defensive physiological autonomic response to subsequent episodes of hypoglycaemia [63]. This phenomenon resets the glycaemic threshold for activation of counter-regulation to a lower glucose value. Consequently, it impairs patient's ability to perceive the onset of hypoglycaemia [64]. No comprehensive definition of IAH is currently in use but Gold et al. [65] and Clarke et al. [66] have proposed scales to recognise IAH in T1DM. The prevalence of IAH is estimated to be as high as 50% in those with T1DM after 25 years of treatment [67].
Hypoglycaemia is associated with considerable morbidity and even mortality in T1DM. It is important to mention; however, that the association of hypoglycaemia with increased mortality does not necessarily imply causation due to potential confounding. Hypoglycaemia might be purely a surrogate marker of poor health as it is likely to be more prevalent in renal and liver pathologies, which independently increase CV risk [68]. In 1991, Tattersall and Gill [69] described the phenomenon of sudden unexplained nocturnal death in young people with T1DM. This fortunately rare, but tragic event was termed the “dead-in-bed” syndrome [70]. It has been hypothesised that nocturnal hypoglycaemia can lead to the dead-in-bed syndrome via its proarrhythmogenic effects [717273]. Mechanisms through which hypoglycaemia exerts its proarrhythmogenic effects include sympathoadrenal activation, hypokalaemia and direct inhibition of the rapid delayed rectifier potassium channels (IKr) in the cardiomyocyte membrane with resulting QT-interval prolongation [7475]. The sympathoadrenal response to hypoglycaemia can also lead to intracytoplasmic calcium overload in cardiomyocytes which is another well-recognised proarrhythmogenic trigger [76]. In observational studies, hypoglycaemia has been shown to increase the relative risk of nocturnal bradycardia in young and otherwise healthy individuals with T1DM [77], as well as in middle-aged people with T2DM and present CV risk factors [78]. Hypopglycaemia has been shown to exert a number of effects on inflammation and thrombosis that can serve to increase CV risk. Hypoglycaemia has been shown to be pro-thrombotic by causing both platelet aggregation and activation [7980] which is likely to be mediated by the catecholamine response to hypoglycaemia [80]. In addition, hypoglycaemia also increases levels of factor VIII, von Willebrand factor and impairs thrombolysis [81]. Furthermore, hypoglycaemia promotes a pro-inflammatory milieu by inducing an acute leukocytosis [82], increasing levels of the pro-inflammatory cytokines tumor necrosis factor α and interleukin 6 and cell adhesion molecules ICAM-1 (intercellular adhesion molecule 1), VCAM-1 (vascular cell adhesion molecule 1), and E-selectin [81]. It has also recently been shown that repeated episodes of hypoglycaemia may directly damage the vascular endothelium in man by impairing nitric oxide mediated endothelial function [83]. Fig. 1 summarises the mechanisms through which hypoglycaemia may potentially increase CV risk.
In addition to adverse biological effects, hypoglycaemia has been shown to significantly impair quality of life in people with T1DM [84]. Negative psychological effects of hypoglycaemia are particularly deleterious as they can form a cognitive barrier preventing treatment of future episodes and thus impeding the treatment of IAH [85].
Given the many risks associated with hypoglycaemia various advances in T1DM have specifically been aimed at mitigating this risk. In addition to new generation insulins and use of technology, structured diabetes education programmes are extremely important interventions that can be deployed in T1DM. Programmes aimed at reversing IAH and reducing SH are based on the observation that even in long-standing IAH, scrupulous avoidance of further hypoglycaemia can restore reduced symptomatic and neuroendocrine responses to hypoglycaemia [8687]. The basic premise of structured education/training programmes in the clinical management of T1DM is to allow patients to better self-manage their diabetes by empowering them with critical skills. The common features amongst various programmes are the use of a fixed curriculum and trained educators that focus on intensive BG monitoring (including nocturnal testing), carbohydrate counting to allow a flexible diet and separation of basal insulin from pre-meal bolus insulin. These fundamental principles are based on the Diabetes Teaching and Treatment Programme (DTTP) that was first delivered over 5 days to inpatients in Germany and former Eastern Bloc European countries in the 1980s [39]. The authors showed in larger trials that education by way of DTTP resulted in improvement of HbA1c in the order of 1.5% to 3% at up to 1 year of follow-up with reduced ketoacidosis but no change in rates of SH [8889]. However, a large observational study involving 9,583 individuals with T1DM has shown that an exponential relationship between the levels of HbA1c and risk of SH can be abolished in those that underwent DTTP training [90]. In the UK, a 5-day structured education programme ‘DAFNE’ modelled on the DTTP was adopted following a multi-centre RCT [40]. In the DAFNE cohort, there were observed improvements in HbA1c at 6 months compared to controls (0.7% to 1%, P<0.0001); however, there was no improvement in the incidence of SH, potentially because the trial was underpowered to detect this difference [40]. Longer-term observational data from a larger number of DAFNE participants have however demonstrated up to a 50% reduction in the rates of SH (mean±SD: pre-DAFNE 1.7±8.5 vs. post-DAFNE 0.6±3.7 episodes per person-year, P<0.05) in addition to improved awareness of hypoglycaemia in up to 43% of participants at 12 months of follow-up [91].
Despite structured education, some people with T1DM continue to experience significant hypoglycaemia or develop IAH. These individuals may have cognitive and psychological barriers that impair their ability to avoid hypoglycaemia [92]. A number of structured education interventions termed ‘psychoeducational’ have been developed to specifically address the needs of this population especially in the context of IAH. Amongst these, the blood glucose awareness training (BGAT) is the most long-standing [93]. In BGAT, participants are taught to recognise and appropriately respond to hypoglycaemia cues including physical symptoms but also cognitive and mood changes. A trained psychologist is part of the education team and assesses progress. BGAT has been shown to improve detection of hypoglycaemia in IAH and reduce hypoglycaemia in those with intact awareness without deteriorating glycaemic control [9495]. The DAFNE-Hypoglycaemia Awareness Restoration Training (DAFNE-HART) is a pilot psycho-educational intervention targeted at those that continue to experience IAH following DAFNE training [85]. The DAFNE-HART intervention has been primarily developed by clinical psychologists that focus on motivational interviews and cognitive behavioural therapy techniques. In 24 individuals with T1DM and confirmed IAH (Gold score ≥4), investigators have reported a significant reduction in SH (median [range]: pre-DAFNE-HART 3 [0 to 104] vs. post-DAFNE-HART 0 [0–3] episodes per person-year, P<0.001) and improved awareness of hypoglycaemia without a worsening of glycaemic control (pre-DAFNE-HART 7.8% [62 mmol/mol] vs. post-DAFNE-HART 7.8% [61.8 mmol/mol]) at 12 months following intervention [85]. Data from this small pilot study await replication in randomized trials with adequate power.
Significant advances in management of T1DM have been made in last decades. This is thanks to novel insulin preparations, advances in technology (CSII, CGM) and a better understanding of the physiology behind detrimental effects of both hypo- and hyperglycaemia. Despite this, daily management of T1DM is a challenging, complex task requiring the continuous application of considerable skill on the part of the person with the condition. There are very few, if any, medical conditions that would require a comparable level of skills, knowledge, and understanding than T1DM. With this in mind, the role of the healthcare professional (ideally working in a multi-disciplinary team) should be supporting individuals to achieve the optimal long-term outcomes. This needs to reflect individual differences in circumstances, social and cultural background and own perceptions and goals in relation to their condition.
Notes
CONFLICTS OF INTEREST: Ahmed Iqbal and Peter Novodvorsky have no relevant conflicts of interest to disclose. Simon R. Heller received research grants from Medtronic UK Ltd. He has served on speaker panels for Sanofi Aventis, Eli Lilly, Takeda, NovoNordisk, and Astra Zeneca for which he has received remuneration. He has served on advisory panels or as a consultant for Boeringher Ingelheim, Novo Nordisk, Eli Lilly, and Takeda for which his institution has received remuneration.
References
1. International Diabetes Federation. IDF diabetes atlas. 8th ed. Brussells: International Diabetes Federation;2017.
2. Diabetes Control and Complications Trial Research Group. Nathan DM, Genuth S, Lachin J, Cleary P, Crofford O, Davis M, Rand L, Siebert C. The effect of intensive treatment of diabetes on the development and progression of long-term complications in insulin-dependent diabetes mellitus. N Engl J Med. 1993; 329:977–986. PMID: 8366922.


3. Polonsky KS, Given BD, Van Cauter E. Twenty-four-hour profiles and pulsatile patterns of insulin secretion in normal and obese subjects. J Clin Invest. 1988; 81:442–448. PMID: 3276730.


4. Rizza RA, Gerich JE, Haymond MW, Westland RE, Hall LD, Clemens AH, Service FJ. Control of blood sugar in insulin-dependent diabetes: comparison of an artificial endocrine pancreas, continuous subcutaneous insulin infusion, and intensified conventional insulin therapy. N Engl J Med. 1980; 30:1313–1318.


5. Banting FG, Best CH, Collip JB, Campbell WR, Fletcher AA. Pancreatic extracts in the treatment of diabetes mellitus. Can Med Assoc J. 1922; 12:141–146. PMID: 20314060.


6. Brown H, Sanger F, Kitai R. The structure of pig and sheep insulins. Biochem J. 1955; 60:556–565. PMID: 13249948.


7. Goeddel DV, Kleid DG, Bolivar F, Heyneker HL, Yansura DG, Crea R, Hirose T, Kraszewski A, Itakura K, Riggs AD. Expression in Escherichia coli of chemically synthesized genes for human insulin. Proc Natl Acad Sci U S A. 1979; 76:106–110. PMID: 85300.


8. Zaykov AN, Mayer JP, DiMarchi RD. Pursuit of a perfect insulin. Nat Rev Drug Discov. 2016; 15:425–439. PMID: 26988411.


9. Heller SR. Insulin analogues. Curr Med Res Opin. 2002; 18(Suppl 1):s40–s47. PMID: 12365818.
10. Bolli GB, Di Marchi RD, Park GD, Pramming S, Koivisto VA. Insulin analogues and their potential in the management of diabetes mellitus. Diabetologia. 1999; 42:1151–1167. PMID: 10525654.


11. Lindholm A, McEwen J, Riis AP. Improved postprandial glycemic control with insulin aspart. A randomized double-blind cross-over trial in type 1 diabetes. Diabetes Care. 1999; 22:801–805. PMID: 10332685.


12. Brunelle BL, Llewelyn J, Anderson JH Jr, Gale EA, Koivisto VA. Meta-analysis of the effect of insulin lispro on severe hypoglycemia in patients with type 1 diabetes. Diabetes Care. 1998; 21:1726–1731. PMID: 9773738.


13. Heller SR, Amiel SA, Mansell P. Effect of the fast-acting insulin analog lispro on the risk of nocturnal hypoglycemia during intensified insulin therapy. U.K. Lispro Study Group. Diabetes Care. 1999; 22:1607–1611. PMID: 10526722.


14. Heise T, Pieber TR, Danne T, Erichsen L, Haahr H. A pooled analysis of clinical pharmacology trials investigating the pharmacokinetic and pharmacodynamic characteristics of fast-acting insulin aspart in adults with type 1 diabetes. Clin Pharmacokinet. 2017; 56:551–559. PMID: 28205039.


15. Russell-Jones D, Bode BW, De Block C, Franek E, Heller SR, Mathieu C, Philis-Tsimikas A, Rose L, Woo VC, Osterskov AB, Graungaard T, Bergenstal RM. Fast-acting insulin aspart improves glycemic control in basal-bolus treatment for type 1 diabetes: results of a 26-week multicenter, active-controlled, treat-to-target, randomized, parallel-group trial (onset 1). Diabetes Care. 2017; 40:943–950. PMID: 28356319.


16. Ratner RE, Hirsch IB, Neifing JL, Garg SK, Mecca TE, Wilson CA. Less hypoglycemia with insulin glargine in intensive insulin therapy for type 1 diabetes. U.S. Study Group of Insulin Glargine in Type 1 Diabetes. Diabetes Care. 2000; 23:639–643. PMID: 10834423.


17. Pedersen-Bjergaard U, Kristensen PL, Beck-Nielsen H, Nørgaard K, Perrild H, Christiansen JS, Jensen T, Hougaard P, Parving HH, Thorsteinsson B, Tarnow L. Effect of insulin analogues on risk of severe hypoglycaemia in patients with type 1 diabetes prone to recurrent severe hypoglycaemia (HypoAna trial): a prospective, randomised, open-label, blinded-endpoint crossover trial. Lancet Diabetes Endocrinol. 2014; 2:553–561. PMID: 24794703.


18. Lane W, Bailey TS, Gerety G, Gumprecht J, Philis-Tsimikas A, Hansen CT, Nielsen TSS, Warren M. Group Information. SWITCH 1. Effect of insulin degludec vs insulin glargine U100 on hypoglycemia in patients with type 1 diabetes: the SWITCH 1 Randomized Clinical Trial. JAMA. 2017; 318:33–44. PMID: 28672316.
19. Wysham C, Bhargava A, Chaykin L, de la Rosa R, Handelsman Y, Troelsen LN, Kvist K, Norwood P. Effect of insulin degludec vs insulin glargine U100 on hypoglycemia in patients with type 2 diabetes: the SWITCH 2 Randomized Clinical Trial. JAMA. 2017; 318:45–56. PMID: 28672317.
20. Davidson MB. Insulin analogs: is there a compelling case to use them? No! Diabetes Care. 2014; 37:1771–1774. PMID: 24855160.
21. Grunberger G. Insulin analogs: are they worth it? Yes! Diabetes Care. 2014; 37:1767–1770. PMID: 24855159.
22. National Institute for Health and Care Excellence: Type 1 diabetes in adults: diagnosis and management. updated 2016 July. https://www.nice.org.uk/guidance/ng17.
23. American Diabetes Association. Standards of medical care in diabetes 2017. Diabetes Care. 2017; 40(Suppl 1):S1–S35. PMID: 27979885.
24. Pickup J, Mattock M, Kerry S. Glycaemic control with continuous subcutaneous insulin infusion compared with intensive insulin injections in patients with type 1 diabetes: meta-analysis of randomised controlled trials. BMJ. 2002; 324:705. PMID: 11909787.


25. Pickup JC, Sutton AJ. Severe hypoglycaemia and glycaemic control in type 1 diabetes: meta-analysis of multiple daily insulin injections compared with continuous subcutaneous insulin infusion. Diabet Med. 2008; 25:765–774. PMID: 18644063.
26. Beato-Víbora P, Yeoh E, Rogers H, Hopkins D, Amiel SA, Choudhary P. Sustained benefit of continuous subcutaneous insulin infusion on glycaemic control and hypoglycaemia in adults with type 1 diabetes. Diabet Med. 2015; 32:1453–1459. PMID: 26213236.


27. Little SA, Leelarathna L, Walkinshaw E, Tan HK, Chapple O, Lubina-Solomon A, Chadwick TJ, Barendse S, Stocken DD, Brennand C, Marshall SM, Wood R, Speight J, Kerr D, Flanagan D, Heller SR, Evans ML, Shaw JA. Recovery of hypoglycemia awareness in long-standing type 1 diabetes: a multicenter 2×2 factorial randomized controlled trial comparing insulin pump with multiple daily injections and continuous with conventional glucose self-monitoring (HypoCOMPaSS). Diabetes Care. 2014; 37:2114–2122. PMID: 24854041.
28. Livingstone R, Boyle JG, Petrie JR. REMOVAL Study Team. A new perspective on metformin therapy in type 1 diabetes. Diabetologia. 2017; 60:1594–1600. PMID: 28770327.


29. Lund SS, Tarnow L, Astrup AS, Hovind P, Jacobsen PK, Alibegovic AC, Parving I, Pietraszek L, Frandsen M, Rossing P, Parving HH, Vaag AA. Effect of adjunct metformin treatment on levels of plasma lipids in patients with type 1 diabetes. Diabetes Obes Metab. 2009; 11:966–977. PMID: 19558610.


30. Petrie JR, Chaturvedi N, Ford I, Brouwers MCGJ, Greenlaw N, Tillin T, Hramiak I, Hughes AD, Jenkins AJ, Klein BEK, Klein R, Ooi TC, Rossing P, Stehouwer CDA, Sattar N, Colhoun HM. REMOVAL Study Group. Cardiovascular and metabolic effects of metformin in patients with type 1 diabetes (REMOVAL): a double-blind, randomised, placebo-controlled trial. Lancet Diabetes Endocrinol. 2017; 5:597–609. PMID: 28615149.
31. Solini A. Role of SGLT2 inhibitors in the treatment of type 2 diabetes mellitus. Acta Diabetol. 2016; 53:863–870. PMID: 27038028.


32. Zinman B, Wanner C, Lachin JM, Fitchett D, Bluhmki E, Hantel S, Mattheus M, Devins T, Johansen OE, Woerle HJ, Broedl UC, Inzucchi SE. EMPA-REG OUTCOME Investigators. Empagliflozin, cardiovascular outcomes, and mortality in type 2 diabetes. N Engl J Med. 2015; 373:2117–2128. PMID: 26378978.


33. Neal B, Perkovic V, Mahaffey KW, de Zeeuw D, Fulcher G, Erondu N, Shaw W, Law G, Desai M, Matthews DR. CANVAS Program Collaborative Group. Canagliflozin and cardiovascular and renal events in type 2 diabetes. N Engl J Med. 2017; 377:644–657. PMID: 28605608.


34. Peters AL, Buschur EO, Buse JB, Cohan P, Diner JC, Hirsch IB. Euglycemic diabetic ketoacidosis: a potential complication of treatment with sodium-glucose cotransporter 2 inhibition. Diabetes Care. 2015; 38:1687–1693. PMID: 26078479.


35. Dandona P, Mathieu C, Phillip M, Hansen L, Griffen SC, Tschope D, Thoren F, Xu J, Langkilde AM. DEPICT-1 Investigators. Efficacy and safety of dapagliflozin in patients with inadequately controlled type 1 diabetes (DEPICT-1): 24 week results from a multicentre, double-blind, phase 3, randomised controlled trial. Lancet Diabetes Endocrinol. 2017; 5:864–876. PMID: 28919061.
36. Petrie JR. SGLT2 inhibitors in type 1 diabetes: knocked down, but up again? Lancet Diabetes Endocrinol. 2017; 5:841–843. PMID: 28919060.


37. Garg SK, Henry RR, Banks P, Buse JB, Davies MJ, Fulcher GR, Pozzilli P, Gesty-Palmer D, Lapuerta P, Simo R, Danne T, McGuire DK, Kushner JA, Peters A, Strumph P. Effects of sotagliflozin added to insulin in patients with type 1 diabetes. N Engl J Med. 2017; 377:2337–2348. PMID: 28899222.


38. Walford S, Gale EA, Allison SP, Tattersall RB. Self-monitoring of blood-glucose. Improvement of diabetic control. Lancet. 1978; 1:732–735. PMID: 76746.
39. Muhlhauser I, Jorgens V, Berger M, Graninger W, Gurtler W, Hornke L, Kunz A, Schernthaner G, Scholz V, Voss HE. Bicentric evaluation of a teaching and treatment programme for type 1 (insulin-dependent) diabetic patients: improvement of metabolic control and other measures of diabetes care for up to 22 months. Diabetologia. 1983; 25:470–476. PMID: 6363175.


40. DAFNE Study Group. Training in flexible, intensive insulin management to enable dietary freedom in people with type 1 diabetes: dose adjustment for normal eating (DAFNE) randomised controlled trial. BMJ. 2002; 325:746. PMID: 12364302.
41. Miller KM, Beck RW, Bergenstal RM, Goland RS, Haller MJ, McGill JB, Rodriguez H, Simmons JH, Hirsch IB. T1DM Exchange Clinic Network. Evidence of a strong association between frequency of self-monitoring of blood glucose and hemoglobin A1c levels in T1DM exchange clinic registry participants. Diabetes Care. 2013; 36:2009–2014. PMID: 23378621.
42. Rodbard D. Continuous glucose monitoring: a review of successes, challenges, and opportunities. Diabetes Technol Ther. 2016; 18(Suppl 2):S3–S13.


43. Boyne MS, Silver DM, Kaplan J, Saudek CD. Timing of changes in interstitial and venous blood glucose measured with a continuous subcutaneous glucose sensor. Diabetes. 2003; 52:2790–2794. PMID: 14578298.


44. Juvenile Diabetes Research Foundation Continuous Glucose Monitoring Study Group. Tamborlane WV, Beck RW, Bode BW, Buckingham B, Chase HP, Clemons R, Fiallo-Scharer R, Fox LA, Gilliam LK, Hirsch IB, Huang ES, Kollman C, Kowalski AJ, Laffel L, Lawrence JM, Lee J, Mauras N, O'Grady M, Ruedy KJ, Tansey M, Tsalikian E, Weinzimer S, Wilson DM, Wolpert H, Wysocki T, Xing D. Continuous glucose monitoring and intensive treatment of type 1 diabetes. N Engl J Med. 2008; 359:1464–1476. PMID: 18779236.
45. Battelino T, Phillip M, Bratina N, Nimri R, Oskarsson P, Bolinder J. Effect of continuous glucose monitoring on hypoglycemia in type 1 diabetes. Diabetes Care. 2011; 34:795–800. PMID: 21335621.


46. Lind M, Polonsky W, Hirsch IB, Heise T, Bolinder J, Dahlqvist S, Schwarz E, Olafsdottir AF, Frid A, Wedel H, Ahlen E, Nystrom T, Hellman J. Continuous glucose monitoring vs conventional therapy for glycemic control in adults with type 1 diabetes treated with multiple daily insulin injections: the GOLD Randomized Clinical Trial. JAMA. 2017; 317:379–387. PMID: 28118454.
47. Beck RW, Riddlesworth T, Ruedy K, Ahmann A, Bergenstal R, Haller S, Kollman C, Kruger D, McGill JB, Polonsky W, Toschi E, Wolpert H, Price D. DIAMOND Study Group. Effect of continuous glucose monitoring on glycemic control in adults with type 1 diabetes using insulin injections: the DIAMOND Randomized Clinical Trial. JAMA. 2017; 317:371–378. PMID: 28118453.
48. Raccah D, Sulmont V, Reznik Y, Guerci B, Renard E, Hanaire H, Jeandidier N, Nicolino M. Incremental value of continuous glucose monitoring when starting pump therapy in patients with poorly controlled type 1 diabetes: the RealTrend study. Diabetes Care. 2009; 32:2245–2250. PMID: 19767384.
49. Bergenstal RM, Klonoff DC, Garg SK, Bode BW, Meredith M, Slover RH, Ahmann AJ, Welsh JB, Lee SW, Kaufman FR. ASPIRE In-Home Study Group. Threshold-based insulin-pump interruption for reduction of hypoglycemia. N Engl J Med. 2013; 369:224–232. PMID: 23789889.


50. Ly TT, Nicholas JA, Retterath A, Lim EM, Davis EA, Jones TW. Effect of sensor-augmented insulin pump therapy and automated insulin suspension vs standard insulin pump therapy on hypoglycemia in patients with type 1 diabetes: a randomized clinical trial. JAMA. 2013; 310:1240–1247. PMID: 24065010.
51. Choudhary P, Olsen BS, Conget I, Welsh JB, Vorrink L, Shin JJ. Hypoglycemia prevention and user acceptance of an insulin pump system with predictive low glucose management. Diabetes Technol Ther. 2016; 18:288–291. PMID: 26907513.


52. Bergenstal RM, Garg S, Weinzimer SA, Buckingham BA, Bode BW, Tamborlane WV, Kaufman FR. Safety of a hybrid closed-loop insulin delivery system in patients with type 1 diabetes. JAMA. 2016; 316:1407–1408. PMID: 27629148.


53. Trevitt S, Simpson S, Wood A. Artificial pancreas device systems for the closed-loop control of type 1 diabetes: what systems are in development? J Diabetes Sci Technol. 2016; 10:714–723. PMID: 26589628.
54. Fokkert MJ, van Dijk PR, Edens MA, Abbes S, de Jong D, Slingerland RJ, Bilo HJ. Performance of the FreeStyle Libre Flash glucose monitoring system in patients with type 1 and 2 diabetes mellitus. BMJ Open Diabetes Res Care. 2017; 5:e000320.


55. Bailey T, Bode BW, Christiansen MP, Klaff LJ, Alva S. The performance and usability of a factory-calibrated flash glucose monitoring system. Diabetes Technol Ther. 2015; 17:787–794. PMID: 26171659.


56. Bolinder J, Antuna R, Geelhoed-Duijvestijn P, Kroger J, Weitgasser R. Novel glucose-sensing technology and hypoglycaemia in type 1 diabetes: a multicentre, non-masked, randomised controlled trial. Lancet. 2016; 388:2254–2263. PMID: 27634581.


57. Novodvorsky P, Walkinshaw E, Rahman W, Gordon V, Towse K, Mitchell S, Selvarajah D, Madhuvrata P, Munir A. Experience with FreeStyle Libre Flash glucose monitoring system in management of refractory dumping syndrome in pregnancy shortly after bariatric surgery. Endocrinol Diabetes Metab Case Rep. 2017; 2017.


58. Danne T, Nimri R, Battelino T, Bergenstal RM, Close KL, DeVries JH, Garg S, Heinemann L, Hirsch I, Amiel SA, Beck R, Bosi E, Buckingham B, Cobelli C, Dassau E, Doyle FJ 3rd, Heller S, Hovorka R, Jia W, Jones T, Kordonouri O, Kovatchev B, Kowalski A, Laffel L, Maahs D, Murphy HR, Norgaard K, Parkin CG, Renard E, Saboo B, Scharf M, Tamborlane WV, Weinzimer SA, Phillip M. International consensus on use of continuous glucose monitoring. Diabetes Care. 2017; 40:1631–1640. PMID: 29162583.


59. Cryer PE. The barrier of hypoglycemia in diabetes. Diabetes. 2008; 57:3169–3176. PMID: 19033403.


60. Workgroup on Hypoglycemia. American Diabetes Association. Defining and reporting hypoglycemia in diabetes: a report from the American Diabetes Association Workgroup on Hypoglycemia. Diabetes Care. 2005; 28:1245–1249. PMID: 15855602.
61. International Hypoglycaemia Study Group. Glucose concentrations of less than 3.0 mmol/l (54 mg/dl) should be reported in clinical trials: a joint position statement of the American Diabetes Association and the Europian Association for the Study of Diabetes. Diabetologia. 2017; 60:3–6. PMID: 27872948.
62. Donnelly LA, Morris AD, Frier BM, Ellis JD, Donnan PT, Durrant R, Band MM, Reekie G. DARTS/MEMO Collaboration. Frequency and predictors of hypoglycaemia in type 1 and insulin-treated type 2 diabetes: a population-based study. Diabet Med. 2005; 22:749–755. PMID: 15910627.


63. Heller SR, Cryer PE. Reduced neuroendocrine and symptomatic responses to subsequent hypoglycemia after 1 episode of hypoglycemia in nondiabetic humans. Diabetes. 1991; 40:223–226. PMID: 1991573.


64. Korzon-Burakowska A, Hopkins D, Matyka K, Lomas J, Pernet A, Macdonald I, Amiel S. Effects of glycemic control on protective responses against hypoglycemia in type 2 diabetes. Diabetes Care. 1998; 21:283–290. PMID: 9539997.


65. Gold AE, MacLeod KM, Frier BM. Frequency of severe hypoglycemia in patients with type I diabetes with impaired awareness of hypoglycemia. Diabetes Care. 1994; 17:697–703. PMID: 7924780.


66. Clarke WL, Cox DJ, Gonder-Frederick LA, Julian D, Schlundt D, Polonsky W. Reduced awareness of hypoglycemia in adults with IDDM. A prospective study of hypoglycemic frequency and associated symptoms. Diabetes Care. 1995; 18:517–522. PMID: 7497862.


67. Hepburn DA, Patrick AW, Eadington DW, Ewing DJ, Frier BM. Unawareness of hypoglycaemia in insulin-treated diabetic patients: prevalence and relationship to autonomic neuropathy. Diabet Med. 1990; 7:711–717. PMID: 2147633.


68. Iqbal A, Heller S. Managing hypoglycaemia. Best Pract Res Clin Endocrinol Metab. 2016; 30:413–430. PMID: 27432075.


69. Tattersall RB, Gill GV. Unexplained deaths of type 1 diabetic patients. Diabet Med. 1991; 8:49–58. PMID: 1826245.


70. Campbell I. Dead in bed syndrome: a new manifestation of nocturnal hypoglycaemia? Diabet Med. 1991; 8:3–4. PMID: 1826241.


71. Harris ND, Heller SR. Sudden death in young patients with type 1 diabetes: a consequence of disease, treatment or both? Diabet Med. 1999; 16:623–625. PMID: 10477205.
72. Marques JL, George E, Peacey SR, Harris ND, Macdonald IA, Cochrane T, Heller SR. Altered ventricular repolarization during hypoglycaemia in patients with diabetes. Diabet Med. 1997; 14:648–654. PMID: 9272590.


73. Tanenberg RJ, Newton CA, Drake AJ. Confirmation of hypoglycemia in the “dead-in-bed” syndrome, as captured by a retrospective continuous glucose monitoring system. Endocr Pract. 2010; 16:244–248. PMID: 19833577.


74. Robinson RT, Harris ND, Ireland RH, Lee S, Newman C, Heller SR. Mechanisms of abnormal cardiac repolarization during insulin-induced hypoglycemia. Diabetes. 2003; 52:1469–1474. PMID: 12765959.


75. Zhang Y, Han H, Wang J, Wang H, Yang B, Wang Z. Impairment of human ether-a-go-go-related gene (HERG) K+ channel function by hypoglycemia and hyperglycemia. Similar phenotypes but different mechanisms. J Biol Chem. 2003; 278:10417–10426. PMID: 12531891.
76. Nordin C. The case for hypoglycaemia as a proarrhythmic event: basic and clinical evidence. Diabetologia. 2010; 53:1552–1561. PMID: 20407743.


77. Novodvorsky P, Bernjak A, Chow E, Iqbal A, Sellors L, Williams S, Fawdry RA, Parekh B, Jacques RM, Marques JLB, Sheridan PJ, Heller SR. Diurnal differences in risk of cardiac arrhythmias during spontaneous hypoglycemia in young people with type 1 diabetes. Diabetes Care. 2017; 40:655–662. PMID: 28213374.


78. Chow E, Bernjak A, Williams S, Fawdry RA, Hibbert S, Freeman J, Sheridan PJ, Heller SR. Risk of cardiac arrhythmias during hypoglycemia in patients with type 2 diabetes and cardiovascular risk. Diabetes. 2014; 63:1738–1747. PMID: 24757202.


79. Wright RJ, Newby DE, Stirling D, Ludlam CA, Macdonald IA, Frier BM. Effects of acute insulin-induced hypoglycemia on indices of inflammation: putative mechanism for aggravating vascular disease in diabetes. Diabetes Care. 2010; 33:1591–1597. PMID: 20587725.
80. Hutton RA, Mikhailidis D, Dormandy KM, Ginsburg J. Platelet aggregation studies during transient hypoglycaemia: a potential method for evaluating platelet function. J Clin Pathol. 1979; 32:434–438. PMID: 469000.


81. Gogitidze Joy N, Hedrington MS, Briscoe VJ, Tate DB, Ertl AC, Davis SN. Effects of acute hypoglycemia on inflammatory and pro-atherothrombotic biomarkers in individuals with type 1 diabetes and healthy individuals. Diabetes Care. 2010; 33:1529–1535. PMID: 20587723.


82. Razavi Nematollahi L, Kitabchi AE, Stentz FB, Wan JY, Larijani BA, Tehrani MM, Gozashti MH, Omidfar K, Taheri E. Proinflammatory cytokines in response to insulin-induced hypoglycemic stress in healthy subjects. Metabolism. 2009; 58:443–448. PMID: 19303962.


83. Joy NG, Tate DB, Younk LM, Davis SN. Effects of acute and antecedent hypoglycemia on endothelial function and markers of atherothrombotic balance in healthy humans. Diabetes. 2015; 64:2571–2580. PMID: 25695946.


84. Davis RE, Morrissey M, Peters JR, Wittrup-Jensen K, Kennedy-Martin T, Currie CJ. Impact of hypoglycaemia on quality of life and productivity in type 1 and type 2 diabetes. Curr Med Res Opin. 2005; 21:1477–1483. PMID: 16197667.


85. de Zoysa N, Rogers H, Stadler M, Gianfrancesco C, Beveridge S, Britneff E, Choudhary P, Elliott J, Heller S, Amiel SA. A psychoeducational program to restore hypoglycemia awareness: the DAFNE-HART pilot study. Diabetes Care. 2014; 37:863–866. PMID: 24319119.


86. Fanelli CG, Epifano L, Rambotti AM, Pampanelli S, Di Vincenzo A, Modarelli F, Lepore M, Annibale B, Ciofetta M, Bottini P, Bolli GB. Meticulous prevention of hypoglycemia normalizes the glycemic thresholds and magnitude of most of neuroendocrine responses to, symptoms of, and cognitive function during hypoglycemia in intensively treated patients with short-term IDDM. Diabetes. 1993; 42:1683–1689. PMID: 8405713.


87. Cranston I, Lomas J, Maran A, Macdonald I, Amiel SA. Restoration of hypoglycaemia awareness in patients with long-duration insulin-dependent diabetes. Lancet. 1994; 344:283–287. PMID: 7914259.


88. Muhlhauser I, Bruckner I, Berger M, Cheta D, Jorgens V, Ionescu-Tirgoviste C, Scholz V, Mincu I. Evaluation of an intensified insulin treatment and teaching programme as routine management of type 1 (insulin-dependent) diabetes. The Bucharest-Dusseldorf Study. Diabetologia. 1987; 30:681–690. PMID: 3123298.
89. Starostina EG, Antsiferov M, Galstyan GR, Trautner C, Jorgens V, Bott U, Muhlhauser I, Berger M, Dedov II. Effectiveness and cost-benefit analysis of intensive treatment and teaching programmes for type 1 (insulin-dependent) diabetes mellitus in Moscow: blood glucose versus urine glucose self-monitoring. Diabetologia. 1994; 37:170–176. PMID: 8163051.
90. Samann A, Muhlhauser I, Bender R, Kloos Ch, Muller UA. Glycaemic control and severe hypoglycaemia following training in flexible, intensive insulin therapy to enable dietary freedom in people with type 1 diabetes: a prospective implementation study. Diabetologia. 2005; 48:1965–1970. PMID: 16132954.


91. Hopkins D, Lawrence I, Mansell P, Thompson G, Amiel S, Campbell M, Heller S. Improved biomedical and psychological outcomes 1 year after structured education in flexible insulin therapy for people with type 1 diabetes: the U.K. DAFNE experience. Diabetes Care. 2012; 35:1638–1642. PMID: 22619082.
92. Rogers HA, de Zoysa N, Amiel SA. Patient experience of hypoglycaemia unawareness in type 1 diabetes: are patients appropriately concerned? Diabet Med. 2012; 29:321–327. PMID: 21913969.
93. Kinsley BT, Weinger K, Bajaj M, Levy CJ, Simonson DC, Quigley M, Cox DJ, Jacobson AM. Blood glucose awareness training and epinephrine responses to hypoglycemia during intensive treatment in type 1 diabetes. Diabetes Care. 1999; 22:1022–1028. PMID: 10388961.


94. Cox DJ, Gonder-Frederick LA, Lee JH, Julian DM, Carter WR, Clarke WL. Effects and correlates of blood glucose awareness training among patients with IDDM. Diabetes Care. 1989; 12:313–318. PMID: 2721340.


95. Cox D, Gonder-Frederick L, Polonsky W, Schlundt D, Julian D, Clarke W. A multicenter evaluation of blood glucose awareness training-II. Diabetes Care. 1995; 18:523–528. PMID: 7497863.


Fig. 1
Putative mechanisms linking hypoglycaemia to increased cardiovascular (CV) risk. Hypoglycaemia is characterised by sympathoadrenal activation. Abnormal cardiac repolarisation and deranged autonomic function as well as the acute haemodynamic consequences of hypoglycaemia can induce CV events via arrhythmias (1) and cardiac ischemia (2). Enhanced coagulation and impaired fibrinolysis (3) in addition to platelet activation and aggregation (6) promotes atherothrombosis. Acute leucocytosis and production of pro-inflammatory cytokines (4) as well as upregulation of cell adhesion molecules (5) is likely to encourage atherogenesis in an incremental fashion.
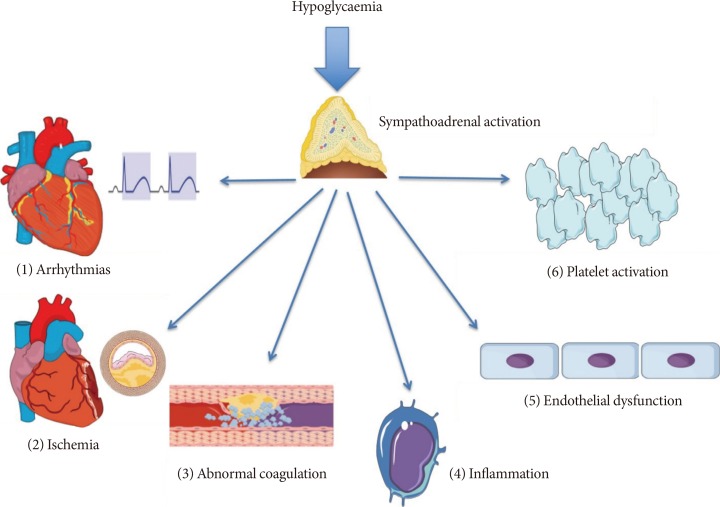
Table 1
The list of currently available insulin preparations in the UK (December 2017)
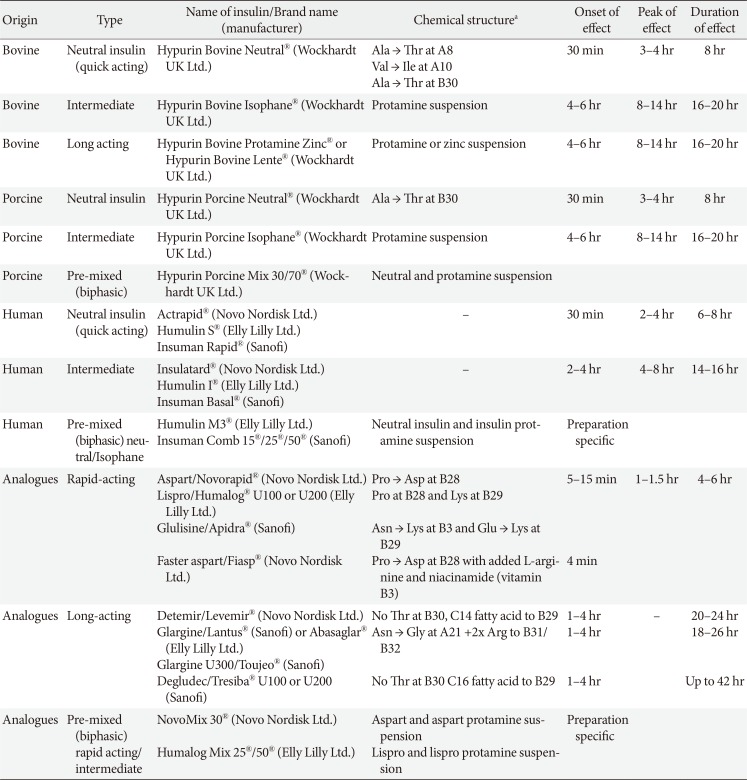
Table 2
Devices for blood/interstitial glucose monitoring: comparison of main features
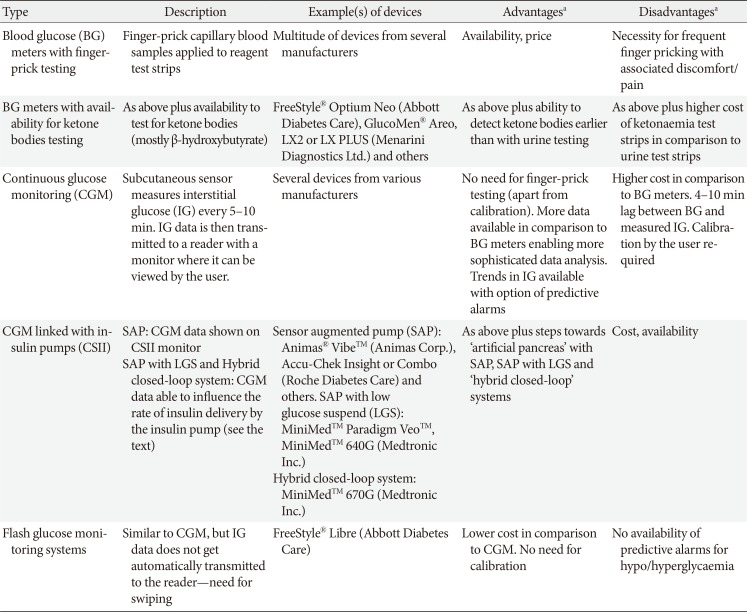