INTRODUCTION
Many patients with neurologic disorders hope to regain walking ability, and various treatments and techniques are used to assist gait training and to enhance neuroplasticity for walking ability. For this purpose, various devices, including robot-assisted gait training (RAGT), have been developed. Since the first introduction of robotic rehabilitation, the clinical application of robotics has become increasingly diverse because of the recent advances in technology. RAGT is gaining popularity, since robotic rehabilitation therapy can deliver high-dose and high-intensity training, while maintaining the physiologic gait pattern [
1]. In addition, RAGT enables gait training and allows early gait training even in patients with severe impairment [
234]. Moreover, RAGT was demonstrated to improve gait kinematics and muscle activation patterns [
5], to assist in gait training by providing body weight support (BWS) and reducing the risk of falling and the effort of therapists to support trunk and limb movements, and even to reduce the costs of rehabilitation by reducing the number of involved therapists, according to some studies [
6].
In recent years, various types of robotic devices have been developed for motor rehabilitation. These devices have a driving component that induces ambulatory movement and a component for BWS. Generally, these robotic devices are divided into 2 categories based on the driven principle: exoskeleton and end-effector type robots [
789]. Exoskeleton robots move in parallel to the skeleton of the patient, so that no additional degrees-of-freedom or range of motion are needed [
10]. End-effector type robots principle of operation is to place patient's feet on footplates with trajectories that simulate the stance and swing phases during gait training [
11].
By partially unloading the body weight, the patient can put less effort into balancing while walking. BWS is conventionally provided by a harness; another type of robotic treadmill supports body weight by a bicycle saddle seat [
12]. BWS enables the patient to concentrate on the leg movements and to increase their walking speed compared to walking on the ground [
9].
This study aimed to investigate the feasibility of RAGT using the Morning Walk®, an end-effector type robot with saddle seat support, for patients with various neurologic disorders. The reasons that prevent completion of RAGT based on the robot characteristics in comparison with exoskeleton or conventional treadmill locomotor devices, and improvement of motor and ambulatory function through RAGT were analyzed. This study will serve as a guide for safer and more effective gait training with end-effector type robots.
MATERIALS AND METHODS
Participants
Outpatients and inpatients from the Department of Physical Medicine and Rehabilitation, National Health Insurance Service Ilsan Hospital between April 2014 and May 2017 were included in this clinical trial. RAGT was applied to patients with diverse neurologic deficits and impaired ambulatory function. Patients who were able to maintain a sitting posture independently without support or with grade C or D spinal cord injury according to the International Standards for Neurological Classification of Spinal Cord Injury were included. Pediatric patients were also included as long as the above conditions were met. However, individuals < 120 cm in height and those with body mass > 120 kg were excluded based on device specifications. In addition, participants who were unable to walk for 5 minutes on a flat surface on the Morning Walk® at screening trial (described below) were excluded.
Device
Morning Walk
® (Curexo, Seoul, Korea) is the only end-effector type robot with body support via saddle seat that is commercially available in Korea (
Fig. 1). The end-effector footplates operate independently in the sagittal plane to simulate locomotor activity and guide the feet to reproduce gait trajectories. The movements that occur during stair ascent and descent can also be reproduced (
Fig. 2). The stride length can be adjusted from 0.30 m to 0.55 m, and the stride rate can be adjusted from 4 to 70 steps per minute. The robot is 3.90 m in length, 1.54 m in width, and 1.95 m in height and weighs 900 kg.
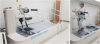 | Fig. 1Images of the Morning Walk®. (A) Gross image of the device; (B) A patient receiving robot-assisted gait training using the Morning Walk®.
|
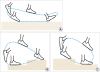 | Fig. 2Foot plate movements of the Morning Walk®. (A) Walking on a flat surface mode; (B) Stair ascent; and (C) Stair descent.
|
Interventions
The RAGT consisted of 3 phases in this study: screening trial, initial assessment, and main treatment. The screening trial was conducted prior to the initial assessment. It consisted of 5 minutes of walking along a “flat surface” mode on the Morning Walk®, followed by 5 minutes of gait in the “stair-up” mode, and another 5 minutes of gait in the “stair-down” mode. If individuals could not walk along the flat surface mode on the Morning Walk® due to lack of endurance, difficulty, or refusal for any other reason, they were excluded from the RAGT. Individuals who were able to walk for 5 minutes on the flat surface modes on the Morning Walk®, but could not complete the stair climbing modes, were still included in the study.
After the screening trial, physiatrists classified patients into hard, difficult, and possible prediction groups through the initial assessment to stratify their ability to complete the RAGT. The criteria used to allocate patients to each prediction group were based on data accumulated during the preliminary demonstration period. BWS was measured from a weight sensor placed in the saddle seat, while ground reaction force was measured from force sensors embedded into the footplates. These data were shown on the monitoring screen to indicate the patient's gait training status.
Patients were allocated to the hard group when > 70% of their body weight was supported during the RAGT and the ground reaction force remained unchanged at < 20% of body weight due to weakness or other cognitive problems, joint range of motion was limited due to contracture or muscle shortening, or an abnormal gait pattern such as genu recurvatum was observed during the robotic treatment and was considered by physiatrists and physicians to potentially cause secondary injuries. They were also assigned to the hard group if the initial assessment trial could not be completed because of discomfort from the saddle seat or if pain continued even after sufficient rest, the patient reacted aggressively during the treatment, or the patient attempted to remove the safety device (trunk fixing belt), compromising safety.
Patients were allocated to the difficult group when > 60% of body weight was supported and continuous intervention from a therapist was needed because of the low ground reaction force or if the patient needed a therapist's intervention because of low trunk stability and trunk trembling, even with a belt to fix the trunk position. Patients in the hard or difficult groups commenced the RAGT unless they refused to participate.
All patients who did not fall into the hard or difficult categories were allocated to the possible group.
After categorizing the included patients into the 3 groups, the main treatment session of RAGT was started. RAGT was performed for 30 minutes, 5 times a week. Each patient participated in 24 robot-assisted training sessions. The patients were required to successfully perform all 24 sessions to complete the RAGT. Failure was defined as an inability to complete all 24 sessions based on the decision of the patient, physical therapist, or physician. Interruptions of RAGT within 3 days due to task-irrelevant conditions such as medical conditions were not considered failure, and RAGT was re-initiated. The causes of failure were collected and analyzed by the physical therapist and physician.
This study was approved by the Institutional Review Board of the National Health Insurance Service Ilsan Hospital (IRB No. 2018-10-005-001). The trial was retrospectively registered at Clinical Research Information Service (trial registration No.
KCT0003627).
Outcome measurements
To assess the improvement in motor and function, the Medical Research Council (MRC) scales of the lower extremities and Functional Ambulation Categories (FAC) were recorded pre- and post-RAGT. Muscle strength of the hip flexor, extensor, abductor, knee flexor, extensor, ankle dorsiflexor, and plantar flexor was measured using the MRC scale, which ranges from 0 to 5. Scores of each right and left lower limb and of the total lower extremities were summed. FAC were recorded from 0 to 5.
Statistical analysis
Shapiro-Wilk test was used to determine the normality of patients' age distribution. MRC and FAC were regarded as nonparametric variables. Wilcoxon's signed-rank test was used to assess the significance of differences between parameters before and after training. Results were considered statistically significant if p values were < 0.05. SPSS Statistics version 25.0 (SPSS Inc., Chicago, IL, USA) was used for all statistical analyses.
RESULTS
A total of 189 patients (65.1% men; mean age, 53.2 [range, 5–87] years) performed RAGT. Patients were classified into the following 5 impairment categories: brain lesions (110 patients), spinal cord injuries (40 patients), Parkinson's diseases (8 patients), peripheral neuropathies (9 patients), and pediatric patients (age < 19 years, 22 patients). Of those with peripheral neuropathies, 7 patients had Guillain-Barré syndrome (GBS), 1 had Charcot-Marie-Tooth disease, and 1 had poliomyelitis (
Table 1).
Table 1
Patient characteristics
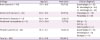
Etiology |
Age (yr) |
Male (sex) |
Clinical diagnosis |
Brain lesions (n = 110) |
57.1 ± 16.8 |
79 (71.8) |
Quadriplegia (n = 32) |
Rt. hemiplegia (n = 44) |
Lt. hemiplegia (n = 34) |
Spinal cord injuries (n = 40) |
61.8 ± 16.5 |
23 (57.5) |
Tetraplegia (n = 18) |
Paraplegia (n = 22) |
Parkinson's diseases (n = 8) |
72.0 ± 10.4 |
2 (25.0) |
- |
Peripheral neuropathies (n = 9) |
51.6 ± 20.0 |
7 (77.8) |
GBS (n = 7) |
CMT (n = 1) |
Poliomyelitis (n = 1) |
Pediatric patients (n = 22) |
12.4 ± 4.3 |
12 (54.5) |
Quadriplegia (n = 10) |
Hemiplegia (n = 6) |
Diplegia (n = 6) |
Total (n = 189) |
53.2 ± 21.9 |
123 (65.1) |
- |
Of the 189 patients, 22 (11.6%) failed to complete the RAGT, and the remaining 167 (88.4%) completed the training. The reasons for discontinuing the RAGT are shown in
Table 2. Of the 22 patients who failed to complete the sessions, 11 had brain lesions, 7 had spinal cord injuries, 1 had peripheral neuropathy (GBS), and 3 were pediatric patients. The numbers of sessions performed by the 22 patients with training failure are shown in
Table 3. No serious events, such as episodes of neurological deterioration, falling, fractures, or skin lesions, occurred during any session in patients that either completed or failed to complete the training.
Table 2
Reasons for discontinuing gait training using the Morning Walk®
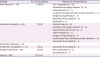
Etiology |
No. (%) of patients |
Reasons for discontinuation |
Brain lesions (n = 110) |
11 (10.0) |
Poor cooperation (n = 6) |
Excessive body-weight support* (n = 2) |
Ankle spasticity (n = 1) |
Limitation of range of motion at the knee joint (n = 1) |
Fatigue during therapy (n = 1) |
Discomfort from saddle (n = 2) |
Back pain (n = 1) |
Spinal cord injuries (n = 40) |
7 (17.5) |
Knee joint pain (n = 3) |
Excessive body-weight support* (n = 1) |
Proprioceptive dysfunction (n = 1) |
Ankle instability (n = 1) |
Fatigue during therapy (n = 1) |
Incontinence from indwelling urinary catheter (n = 1) |
Discomfort from saddle (n = 1) |
Parkinson's diseases (n = 8) |
- |
- |
Peripheral neuropathies (n = 9) |
1 (11.1) |
Discomfort from saddle (n = 1) |
Pediatric patients (n = 22) |
3 (13.6) |
Poor cooperation (n = 2) |
Hip joint pain (n = 1) |
Total (n = 189) |
22 (11.6) |
- |
Table 3
Characteristics of patients who discontinued training
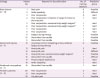
Etiology |
Patient No. |
Reasons for discontinuation |
No. of training sessions |
Prediction group |
Brain lesions (n = 11) |
1 |
Back pain |
6 |
Possible |
2 |
Ankle spasticity |
3 |
Difficult |
3 |
Poor cooperation |
2 |
Hard |
4 |
Poor cooperation, limitation of range of motion at the knee joint |
1 |
Hard |
5 |
Poor cooperation, excessive body-weight support*
|
1 |
Hard |
6 |
Poor cooperation, excessive body-weight support*
|
1 |
Hard |
7 |
Poor cooperation |
1 |
Hard |
8 |
Poor cooperation |
1 |
Hard |
9 |
Fatigue during therapy |
1 |
Possible |
10 |
Discomfort from saddle |
1 |
Possible |
11 |
Discomfort from saddle |
1 |
Possible |
Spinal cord injuries (n = 7) |
12 |
Incontinence from indwelling urinary catheter, fatigue during therapy |
6 |
Possible |
13 |
Knee joint pain |
2 |
Hard |
14 |
Knee joint pain |
2 |
Difficult |
15 |
Knee joint pain, excessive body-weight support*
|
1 |
Possible |
16 |
Discomfort from saddle |
1 |
Difficult |
17 |
Proprioceptive dysfunction |
1 |
Hard |
18 |
Ankle instability |
1 |
Hard |
Peripheral neuropathies (n = 1) |
19 |
Discomfort from saddle |
1 |
Hard |
Pediatric patients (n = 3) |
20 |
Poor cooperation |
2 |
Hard |
21 |
Poor cooperation |
1 |
Hard |
22 |
Hip joint pain |
1 |
Possible |
The most common reason for failure was difficulty following directions due to poor cooperation. Eight patients had difficulties to follow the therapist's instructions; 6 of these patients had brain lesions and 2 were pediatric patients who were both 5 years old. Among the 6 patients with brain lesions, 1 patient had a Mini-Mental State Examination (MMSE) score of 25 and could obey a 3-step command, but had decreased motivation; 4 patients had MMSE scores of 11, 23, 29, and 29, and were unable to cooperate due to aggressive behavior; and 1 patient who had an MMSE score of 7 could only obey a 1-step command and had difficulties to follow instructions. Three pediatric patients who failed to complete the RAGT were diagnosed with Angelman syndrome, hypoxic brain injury, or cerebral palsy. All patients who discontinued the RAGT due to poor cooperation were children or adults with brain lesions.
The second most common reason for failure was musculoskeletal pain. Of the 5 patients who discontinued the training due to pain, 3 patients experienced knee joint pain, 1 had back pain, and 1 had hip joint pain. All patients with knee joint pain had spinal cord injuries; the patient with back pain had a brain lesion, and a pediatric patient with cerebral palsy experienced hip joint pain. Spinal X-rays in the 2 patients who complained of back pain did not show any specific findings. All 5 individuals were evaluated and treated immediately, and pain was successfully alleviated. Two patients with knee pain had osteoarthritis, and the other patient with knee pain was diagnosed with septic arthritis. RAGT was not considered the major cause of the pain, but it was not resumed because of possible pain recurrence and as a precaution to prevent pain worsening.
The third most common reason was saddle seat discomfort (4 patients). Two of these patients had brain lesions, 1 had a spinal cord injury, and the other had peripheral neuropathy.
In 3 individuals, the reason for failure was excessive BWS. This was defined as the support > 70% body weight, with a ground reaction force < 20% of body weight. Two of these patients had brain lesions and 1 had a spinal cord injury. Other reasons for discontinuing the RAGT included ankle spasticity, restricted knee joint motion, ankle instability, proprioceptive dysfunction, incontinence from an indwelling urinary catheter, and fatigue during the therapy in patients with spinal cord injuries or brain lesions (
Table 2).
Patient's refusal to continue RAGT or physical therapist's judgment to discontinue RAGT was reported to the physician, and the physician made the final decision on RAGT continuation. The reasons for patient's request for discontinuation included saddle discomfort, muscular or joint pain, or fatigue during therapy. Poor cooperation, excessive BWS, limitation of the knee joint range of motion, ankle spasticity, proprioceptive dysfunction, and incontinence from indwelling catheter were causes that physical therapists reported to the physicians to stop RAGT.
After the initial assessment, there were 12, 9, and 168 patients in the hard, difficult, and possible prediction groups, respectively. All patients in the hard group failed to complete the RAGT sessions. Three patients in the difficult group dropped out, and 6 (66.7%) completed the sessions. Of the 168 patients in the Possible group, only 7 (4.2%) patients failed to complete the RAGT (
Table 4).
Table 4
Patient prediction categories and completion or failure of the RAGT
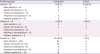
Prediction group |
Completion |
Failure |
Hard (n = 12) |
0 (0.0) |
12 (100.0) |
|
Brain lesions (n = 6) |
0 |
6 |
|
Spinal cord injuries (n = 3) |
0 |
3 |
|
Parkinson's diseases (n = 0) |
- |
- |
|
Peripheral neuropathies (n = 1) |
0 |
1 |
|
Pediatric patients (n = 2) |
0 |
2 |
Difficult (n = 9) |
6 (66.7) |
3 (33.3) |
|
Brain lesions (n = 5) |
4 |
1 |
|
Spinal cord injuries (n = 3) |
1 |
2 |
|
Parkinson's diseases (n = 0) |
- |
- |
|
Peripheral neuropathies (n = 0) |
- |
- |
|
Pediatric patients (n = 1) |
1 |
0 |
Possible (n = 168) |
161 (95.8) |
7 (4.2) |
|
Brain lesions (n = 99) |
95 |
4 |
|
Spinal cord injuries (n = 34) |
32 |
2 |
|
Parkinson's diseases (n = 8) |
8 |
0 |
|
Peripheral neuropathies (n = 8) |
8 |
0 |
|
Pediatric patients (n = 19) |
18 |
1 |
In the comparison between the pre- and post-training motor and ambulatory functions, patients with brain lesions, spinal cord injuries, and peripheral neuropathies showed statistically significant improvement in both the MRC scales and FAC. Patients with Parkinson's diseases and pediatric patients did not show meaningful improvement in the MRC scales of the lower extremities, but showed improvement in FAC (
Table 5).
Table 5
Changes in functional outcome measures pre- and post-training
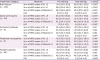
Impairment category |
Measures |
Pre-training |
Post-training |
p value*
|
Brain lesions (n = 100) |
Sum of MRC scales of Rt. LE |
21.0 (18.0–35.0) |
24.0 (19.0–35.0) |
< 0.001†
|
Sum of MRC scales of Lt. LE |
26.0 (20.0–35.0) |
28.0 (21.0–35.0) |
< 0.001†
|
Sum of MRC scales of bilateral LE |
50.0 (43.8–56.0) |
52.0 (47.0–56.0) |
< 0.001†
|
FAC |
2.0 (1.0–3.0) |
3.0 (2.0–4.0) |
< 0.001†
|
Spinal cord injuries (n = 40) |
Sum of MRC scales of Rt. LE |
22.0 (17.0–26.0) |
23.1 (19.5–27.0) |
< 0.001†
|
Sum of MRC scales of Lt. LE |
20.8 (17.0–26.5) |
22.7 (19.0–27.0) |
< 0.001†
|
Sum of MRC scales of bilateral LE |
42.5 (34.3–52.0) |
46.0 (40.0–54.0) |
< 0.001†
|
FAC |
1.7 (0.5–3.0) |
3.0 (2.0–4.0) |
< 0.001†
|
Parkinson's diseases (n = 8) |
Sum of MRC scales of Rt. LE |
26.5 (24.0–28.0) |
27.0 (24.0–28.0) |
0.157 |
Sum of MRC scales of Lt. LE |
26.5 (21.8–28.0) |
27.0 (21.8–28.0) |
0.180 |
Sum of MRC scales of bilateral LE |
53.0 (43.3–56.0) |
54.0 (43.3–56.0) |
0.180 |
FAC |
1.0 (0.8–2.0) |
2.0 (2.0–3.0) |
0.014†
|
Peripheral neuropathies (n = 9) |
Sum of MRC scales of Rt. LE |
21.0 (19.0–22.0) |
25.0 (22.0–26.0) |
0.018†
|
Sum of MRC scales of Lt. LE |
21.0 (19.0–25.0) |
25.0 (22.0–27.0) |
0.028†
|
Sum of MRC scales of bilateral LE |
42.0 (35.0–48.5) |
50.0 (43.0–53.5) |
0.018†
|
FAC |
2.0 (1.0–3.0) |
3.0 (3.0–4.0) |
0.023†
|
Pediatric patients (n = 22) |
Sum of MRC scales of Rt. LE |
19.0 (17.0–23.0) |
19.0 (17.0–23.0) |
0.066 |
Sum of MRC scales of Lt. LE |
21.0 (17.0–23.5) |
21.0 (17.0–25.8) |
0.180 |
Sum of MRC scales of bilateral LE |
42.0 (34.0–46.3) |
42.0 (34.8–48.3) |
0.066 |
FAC |
2.0 (0.0–3.0) |
2.5 (0.0–4.0) |
0.002†
|
DISCUSSION
This study demonstrated that an end-effector-type automated gait machine with saddle support is feasible and safe to use in patients with a diverse spectrum of neurologic disorders.
Previous studies have evaluated the safety and feasibility of other gait assist robotic devices, such as body-weight-supported treadmill training (BWSTT) or exoskeleton devices. A small pilot study with nonrandomized design evaluated the effect of gait training with manual BWSTT using an ankle-foot orthosis for 30 minutes every day in 7 post-stroke adults. No adverse event was reported by any participant who used the treadmill [
8]. In another study on locomotor training, 408 participants with stroke were stratified into early group (n = 139; 2 months after stroke onset), late group (n = 143; 6 months after stroke onset), and home exercise group (n = 126); patients performed manual BWSTT for 36 sessions of 20–30 minutes for 12–16 weeks. Multiple falls were the most common serious adverse event, with 57 cases (41.0%) in the early locomotor-training group, 47 (32.9%) in the late locomotor-training group, and 35 (27.8%) in the home-exercise group. Fracture occurred in 8 (5.8%) patients in the early locomotor group, 8 (5.6%) in the late locomotor group, and 5 (4.0%) in the home-exercise group. The reported rate of minor adverse events, mostly falls with no fracture, was 55.9%, with no significant differences among groups [
13]. In the present study, no events of fall or fracture were recorded, which suggests that manual support-only BWSTT might require more balance and cooperation, thus increasing the above-mentioned risks.
Another study reported on adverse events encountered during gait training of children and adolescents with the Lokomat
® (Hocoma AG, Volketswil, Switzerland), an exoskeleton device with a treadmill and a suspension system [
1415]. In 89 patients who underwent 12 therapy sessions for over a 3-week period, 16 (34.0%) had muscle pain, 14 (29.8%) had joint pain, 12 (25.5%) had skin erythema, 4 (8.5%) had open skin lesions, and 1 (2.1%) had tendinopathy. Skin erythema at the cuffs and leg orthoses of the device and muscle and joint pains were generally mild, but in 5 (5.6%) patients, open skin lesions (n = 2), joint pain (n = 2), or tendinopathy (n = 1) led to discontinuation of the therapy [
14]. In the present study, there were also 2 cases of skin erythema at the saddle site, but it was not very severe to interfere with the treatment.
The failure rate might seem higher in our study compared to the aforementioned studies. However, most of the patients in the Failure group only underwent 1 trial session. We believe that the present study is valuable in terms of categorizing patients through initial assessment to predict the possibility of RAGT completion.
The most common reason for failure was poor cooperation with 8 patients, including 6 patients with brain lesions and 2 pediatric patients. Exoskeleton type robots have components for the hip, knee, and ankle joints that provide direct control of individual joints including patient's distal limbs and programmable drives that flex the hips and knees during the swing phase [
21116]. Thus, gait cycles could be more easily controlled by exoskeleton devices. End-effector type robots require relatively more strength and balance, hence requiring more cooperation [
8]. Considering the characteristics of patients who dropped out of the study, patients' ability to adequately follow instructions before applying robotic training should be taken into account, as this cannot be predicted by the MMSE score alone. Pediatric patients also need to have a certain degree of awareness and comprehension skills to successfully complete RAGT.
Muscle and joint pain as well as skin erythema were reported to be the most common adverse events in exoskeleton type robots, with joint pain affecting lower extremity, including the hip, knee, and ankles [
14]. In the present study, the knee joint was the most common site of pain occurrence, and developed only in patients with spinal cord injuries and was the most common cause of discontinuity in these patients. These findings correlate with the results of a previous report on overuse pain and swelling developed in patients with spinal cord injuries using exoskeleton type robots [
17]. This previous study analyzed 52 participants with spinal cord injuries who underwent gait training with wearable exoskeletons from Ekso Bionics (Ekso Bionics, Richmond, CA, USA). Ankle swelling developed in 3 patients who dropped out of the study due to overuse injury. Therefore, motor training in patients with spinal cord injury and insufficient motor power may be more burdensome and cause joint strain, which should be considered carefully for further enrollment.
Moreover, hip joint pain developed in a pediatric patient with cerebral palsy and hip dislocation. Since the Morning Walk® is a saddle-assisted robot, special care will be needed for such patients. Patients with hip dislocations, arthritic symptoms, or any other musculoskeletal pain symptoms should be screened before RAGT initiation and carefully monitored during RAGT to prevent worsening of their symptoms.
Saddle seat discomfort, which was one of the common failure reasons, seems to be specific to the Morning Walk
® because of its saddle seat-supported design. The off-loading of some of the patient's weight by the BWS reduces the load that needs to be overcome by the patient, ensures safety and stability during walking, and reduces the effort required from therapists [
18]. A harness is commonly used for BWS; however, it requires modification of the ceiling and takes time and effort of therapists during training. The saddle seat support is much less time consuming and requires, on average, less than 5 minutes to prepare the patient. Compared to a suspension harness, the use of a saddle seat for BWS makes mounting and dismounting easier. This avoids the discomfort caused by the harness suspension and saves time [
19]. However, some participants found the seat uncomfortable, and this should be considered by the device manufacturers. Some obese patients complained of saddle seat discomfort, and in some cases, their inner thigh was swept and irritated by the saddle seat. Moreover, there were 2 additional cases of inner thigh irritation caused by the saddle seat that did not lead to training discontinuation. Changing the seat material and shape might improve the discomfort. However, exoskeleton type robots were also associated with erythema or stage 1 or 2 pressure ulcers at the thigh, tibia, or foot points that are directly in contact with the device [
17]. In addition, exoskeleton type robots with BWS belt caused mechanical irritations and open skin lesions at the thigh [
14].
While exoskeleton type robots support the knee joint during the stance phase, end-effector type robots may need support from a saddle or harness, manual assistance, or functional electrical stimulation of the quadriceps to stabilize the knee in patients with significant weakness [
11]. Therefore, patients who require more BWS may have increased difficulty of completing the RAGT. Failed cases due to excessive BWS were all included in the hard or difficult group at the initial assessment. Moreover, they had difficulties in performing the stair-up and stair-down modes and all failed in the first trial of treatment.
There was one patient with ankle joint spasticity, one patient with ankle joint instability, and another patient with limited knee joint motion who failed to complete the RAGT. For the end-effector type robots, the footplates are the only place for interaction with the patient's body; therefore, poor joint stability typically cannot be controlled. Since only the distal parts of the body are fixed, the patients' ankles and knees cannot be fully stabilized [
1]. This explains the ankle spasticity, ankle instability, limited knee joint range of motion as reasons for the RAGT failure. Additionally, this characteristic of end-effector type robots may have made their application more difficult in patients with knee joint pain or proprioceptive dysfunction.
One patient failed to complete the RAGT due to incontinence from the indwelling urinary catheter. Many other patients with spinal cord injuries had indwelling Foley or suprapubic catheters, but they did not develop incontinence problems. The saddle itself did not cause irritation of the catheters. The patient who failed had previous incontinence problems from volume-oriented clamping training due to small bladder capacity, and a diaper was recommended, but the patient refused because of discomfort. The trial had to be discontinued due to safety issues regarding the robot being an electric device.
In the present study, 3 satisfaction surveys were conducted for 5 physical therapists. The survey revealed considerable reduced setup time of the Morning Walk
®, compared to the previous exoskeleton robotic devices used in our center (Lokomat
® and Exowalk
®), which therefore indicated reduced physical therapist workloads. In traditional overground locomotor training, 3 or more therapists are required to stabilize the patient's pelvis and to guide leg movements; in gait with treadmill training, 2 therapists are required to stabilize the patient's pelvis and to control the treadmill [
20]. The hip, knee, and ankle joints in exoskeleton type robots minimize abnormal posture or movement, but correlate with time and effort required to set up the devices [
2]. End-effector type robots have superiority in effectiveness to traditional locomotor or treadmill training and exoskeleton type devices [
220]. Physical therapists also reported satisfaction for the flat surface, stair-up and stair-down modes, through which the most suitable mode could be applied individually to the patients.
The participants were also surveyed after the completion of 24 sessions, and most of them reported satisfaction of the device. Especially, patients with FAC scores of 2–3 with motor recovery, who just started to walk with physical assistance, expressed their preference for the end-effector due to the subjective feelings of walking with their own effort compared to the exoskeleton type robot. In line with this self-satisfaction, a previous study reported that the end-effector type robot might allow better legs extension with more freedom, even though requiring more effort for balance [
8]. The patients were also satisfied with the 3 modes of the robot.
Our prediction criteria were accurate for the hard and possible groups, but they did not correlate well with the difficult group. Since the difficult group was defined by BWS and trunk instability, the assistance of physical therapists may have provided positive reinforcement for completing the sessions. Nevertheless, this requirement for therapist intervention emphasizes that safety issues must be carefully considered when conducting RAGT with patients in this group.
Previous studies have focused on RAGT in patients with brain lesions or spinal cord injuries [
2621]; however, the results of this study are significant due to the inclusion of patients with Parkinson's diseases, peripheral neuropathies, and pediatric patients with diverse neurologic diagnoses. Nevertheless, this study has limitations. First, the proportion of patients with brain lesions and spinal cord injuries was much higher than that of the other impairment groups. Further studies using randomized controlled designs and a larger number of patients with other diagnoses will help clarify the feasibility of RAGT. Second, the time from the onset of the disorders was uncertain and ranged widely in included patients. Since recovery of walking function is known to mainly occur within the first 11 weeks after many neurologic disorders, such as stroke [
22], and better gait recovery occurs when training starts earlier [
18], further studies focusing on patients after shorter onset periods may enhance compliance and improvements.
Even though the Morning Walk
® still requires the intervention of therapists or constant vigilance, by classifying the participants according to our criteria, the need for continuous intervention and manual assistance that have been challenging for therapists and patients will be reduced [
23]. The complaints of the participants and the difficulties identified in this study will contribute to the development and customization of safe and comfortable end-effector type robots and to the design of future clinical trials. RAGT is widely used, and appropriate patients selection according to the device characteristics is mandatory. We conducted a preliminary assessment and classified patients into hard, difficult, and possible groups considering BWS and ground reaction force. This classification has proven to be very useful when applied to the treatment of patients in clinical practice.
In conclusion, this study demonstrated that the Morning Walk®, an end-effector type robotic device with saddle seat support, is feasible and safe for RAGT in patients with diverse neurologic disorders. The study results also indicate that various factors, such as patient cooperation, musculoskeletal pain, saddle seat discomfort, restricted joint motion, and ankle spasticity, should be considered before applying the Morning Walk®. Furthermore, categorizing patients by our preliminary initial assessment was proven to be useful in predicting the completion of RAGT.