Abstract
Background
The CS-5100 high-throughput automated coagulometer (Sysmex, Japan) can perform general and special coagulation assays. We evaluated the performance of the CS-5100 coagulometer to measure fibrinogen degradation product (FDP), plasma level of unfractionated heparin (UFH), low molecular weight heparin (LMWH), and direct oral anticoagulants (DOACs).
Methods
Precision, linearity, and carryover rate of the CS-5100 while measuring FDP, UFH, and LMWH were validated. Precision and linearity were validated for measurements of DOACs. Results of FDP measurement using CS-5100 were compared to those using the currently used STA-R coagulometer (Diagnostica Stago, France). The reference range of FDP was established. All evaluation procedures were in accordance with Clinical and Laboratory Standards Institute guidelines.
The CS-5100 (Sysmex, Kobe, Japan) is a fully automated coagulation analyzer that optically detects clot formation. It can achieve high sample throughput, with 357 tests per hour for prothrombin time (PT) and activated partial thromboplastin time (aPTT), and 268 tests per hour for PT, aPTT, brinogen, and D-dimer. A newly installed feature of the CS-5100 is a pre-analytic check system with multi-wavelength detection. It can automatically detect insufficient sample volume and pre-analytical variables, such as hemolysis, hyperbilirubinemia, and hyperlipidemia [12]. The CS-5100 can perform general and special coagulation assays that include PT, aPTT, brinogen, D-dimer, brinogen degradation product (FDP), and coagulation factor activity. It can also measure plasma anticoagulant concentration. Several studies have demonstrated that the CS-5100 is suitable for measuring PT, aPTT, brinogen, D-dimer, and coagulation factor activity [1234].
The objective of this study was to evaluate the performance of CS-5100 in measuring FDP, plasma levels of unfractionated heparin (UFH), low molecular weight heparin (LMWH), and three direct oral anticoagulants (DOACs, including rivaroxaban, apixaban, and dabigatran).
The BL-2 P-FDP latex test kit (Biolinks Co. Ltd., Tokyo, Japan) was used for FDP analysis. The BIOPHEN Heparin (LRT; Hyphen BioMed, Neuville-sur-Oise, France) was used for heparin and LMWH tests. The BIOPHEN™ DiXaI (Hyphen BioMed) was used for rivaroxaban and apixaban tests. HEMOCLOT™ Thrombin Inhibitors (Hyphen BioMed) were used for dabigatran tests. Control materials and their concentrations were as follows: P-FDP control, 8 and 28 µg/mL (BIOLINKS Co., Ltd, Japan); BIOPHEN™UFH Control Plasma, 0.20 and 0.50 IU/mL; BIOPHEN™LMWH Control Plasma (Low Range), 0.25 and 0.50 IU/mL; BIOPHEN™LMWH Control Plasma (High Range), 0.80 and 1.20 IU/mL; BIOPHEN™ Rivaroxaban Control, 100 and 300 ng/mL; BIOPHEN™ Apixaban Control, 200 and 400 ng/mL; and Dabigatran Control Plasma, 100 and 300 ng/mL. All anticoagulant controls were manufactured by Hyphen BioMed.
The turbidimetric latex immunoassay was used to measure FDP. The chromogenic anti-factor Xa assay was used to measure plasma concentrations of UFH, LMWH, rivaroxaban, and apixaban. The assay was based on the inhibition of activated factor X (factor Xa) by factor Xa inhibitors. Factor Xa can hydrolyze para-nitroaniline (p-NA) connected to a chromogenic substrate. Increasing factor Xa inhibitor concentrations will block the activity of factor Xa on the chromogenic substrate in a dose-dependent manner. Therefore, the amount of p-NA generated is inversely proportional to the concentration of factor Xa inhibitor in the tested plasma. The concentration of factor Xa inhibitor is plotted against the optical density measured at 405 nm, which is the absorbance of the released p-NA. To measure the plasma concentration of dabigatran, the diluted thrombin time assay was used with diluted tested plasma and a constant amount of human alpha-thrombin. The clotting time measured was directly related to the concentration of dabigatran in the tested plasma.
The study protocol was reviewed and approved by the Institutional Review Board of Asan Medical Center, Seoul, Korea (2015-0595). Whole blood was collected via venipuncture into a 3.2% sodium citrate tube (Becton Dickenson, Franklin Lakes, NJ, USA) and centrifuged at 2,500 g for 10 minutes to separate plasma. The plasma was either analyzed within 4 hours after blood collection or aliquoted and stored at below −70℃ when analysis was delayed. In addition, we made platelet-poor normal pooled plasma (NPP) for preparing rivaroxaban and apixaban-spiked plasma. The NPP was divided into aliquots and frozen immediately at −70℃. Just before each experiment, frozen NPP aliquots were thawed and heated at 37℃ for 10 min. Thawed NPP aliquots were spiked with rivaroxaban or apixaban to obtain target drug concentrations
Rivaroxaban was prepared from 20 mg Xarelto® tablet (Bayer-Schering-Pharma, Wuppertal, Germany) and apixaban was prepared from 2.5 mg Eliquis® tablet (Bristol-Myers Squibb Company, Princeton, NJ, USA). Each DOAC was dissolved in dimethylsulfoxide (DMSO) to make a stock solution diluted in phosphate-buffered saline without Ca2+ or Mg2+. Each working solution was obtained by mixing these stock solutions with NPP. The plasma DMSO concentration was ≤0.05% (v/v), which did not influence coagulation [5].
According to the Clinical and Laboratory Standards Institute (CLSI) EP05-A3 guidelines [6], precision was estimated using low and high concentrations of FDP, UFH, LMWH, rivaroxaban, apixaban, and dabigatran control materials. Within-run and between-run precisions were determined by analyzing two runs per day with duplicates for each concentration daily for five days. Precision was considered acceptable if the coefficient of variation (CV) was ≤5%.
According to the CLSI EP06-A guidelines [7], linearity was evaluated for FDP, UFH, LMWH, rivaroxaban, and apixaban. Two samples with high and low concentrations of FDP (4.7 µg/mL and 88.3 µg/mL), UFH (0.00 IU/mL and 1.65 IU/mL), LMWH (0.00 IU/mL and 2.00 IU/mL), rivaroxaban (50 ng/mL and 500 ng/mL), and apixaban (50 ng/mL and 500 ng/mL) were mixed at ratios of 4:0, 3:1, 1:1, 1:3, and 0:4, respectively. Each mixed sample was measured in duplicate. Correlation coefficient and regression line were calculated between expected value on the X axis and the measured value on the Y axis.
A total of six patient samples with high and low concentrations of FDP, UFH, and LMWH were assayed in the order H1, H2, H3, H4, L1, L2, L3, and L4 (H for high and L for low) as described in the CLSIEP10-A3 guideline [8]. The carryover rate (%) between specimens was calculated as {L1−(L3+L4)/2}/{(H3+H2)/2−(L3+L4)/2}×100. The result was considered acceptable if the rate was <1%.
Results obtained with the CS-5100 coagulometer were compared with those from the STA-R Evolution coagulometer (Diagnostica Stago, Asnieres, France), which is the coagulation analyzer currently used to measure FDP. According to the CLSI EP09-A3 guideline [9], 29 normal samples (FDP range 0.7–5.8 µg/mL) and 24 abnormal samples (FDP range 7.4–107.7 µg/mL) obtained from patients with disseminated intravascular coagulation due to malignancy, sepsis, liver cirrhosis, and trauma were used to obtain FDP results using the CS-5100 analyzer and STA-R analyzer in duplicate. Correlation between two coagulometers was evaluated using the Passing-Bablok regression analysis and Spearman's correlation coefficient.
The reference range of FDP was established according to CLSI EP28-A3c [10]. A total of 120 blood samples that showed normal results for complete blood count, PT, and aPTT were collected from 60 healthy men and 60 healthy women. The FDP level was analyzed with the CS-5100 system. Lower and upper reference limits were estimated as the 2.5th and 97.5th percentiles of the distribution of FDP test results.
All statistical analyses were performed using MedCalc® Statistical Software version 14.12.0 (MedCalc Software, Mariakerke, Belgium). Correlation of FDP results between the CS-5100 and STA-R coagulometers was analyzed using Passing-Bablok regression analysis and Spearman's correlation coefficient. In Passing-Bablok regression analysis, we judged that there was no significant difference between two analyzers if the 95% confidence interval (CI) of the intercept included point zero and the 95% confidence interval of the slope included one [12]. Statistical significance level was set at P<0.05.
Within-run and between-run precisions expressed as CV (%) was <5% with low and high levels of control materials (Table 1). Linear regression equation obtained from estimated and actual measurements was y=1.004x + 0.299. Coefficients of determination (R2) were >0.99 (Fig. 1). The correlation between the CS-5100 and STA-R coagulometer was evaluated using the Spearman's correlation coefficient, Passing-Bablok regression analysis, and Bland-Altman difference plot (Fig. 2). In Passing-Bablok regression, the 95% CI of the slope was 0.944–1.112 (containing value one), while that of the intercept was 1.048–1.157 (not containing value zero). Spearman's correlation coefficient was 0.955. At the current upper limit of reference range of 5 µg/mL on STA-R analyzer, the estimated value on CS-5100 was 6.1 µg/mL (95% CI: 5.4–6.4 µg/mL). The carryover rate between specimens was 0.4%, which was within acceptable limits. Results of FDP level of citrated plasma from 120 healthy adults showed a minimum of 0.0 µg/mL and a maximum of 5.3 µg/mL. The mean value of measured FDP level was 1.3 µg/mL with a standard deviation of 0.85. The range between the 2.5th and 97.5th percentiles was 0.0 to 3.48 µg/mL. This range was adopted as the reference range of the FDP level on the CS-5100 system.
Within-run and between-run precisions expressed as CV (%)
for UFH was <5% with low and high levels of control materials (Table 1). Linear regression equation obtained from estimated and actual measurements was y=0.995x + 0.006. Coefficients of determination (R2) were >0.99 (Fig. 1). The carryover rate between specimens was 0.00%, which was within acceptable limits.
Within-run and between-run precisions expressed as CV (%) for LMWH was <5% with low and high levels of control materials (Table 1). Linear regression equation obtained from estimated and actual measurements was y=−0.006x + 1.008. Coefficients of determination (R2) were >0.99 (Fig. 1). The carryover rate between specimens was 0.00%, which was within acceptable limits.
Within-run and between-run precisions expressed as CV (%) for rivaroxaban and apixaban were <5% with low and high levels of control materials (Table 1). Linear regression equation obtained from estimated and actual measurements were as follows: y=0.996x + 15.132 for rivaroxaban, and y=0.978x + 4.502 for apixaban. Coefficients of determination (R2) were >0.99 for both parameters (Fig. 1).
Within-run and between-run precisions expressed as CV (%) for dabigatran was <5% with low and high levels of control materials (Table 1).
The CS-5100 is an automated, high-throughput coagulometer with multi-wavelength technology (340, 405, 575, 660, and 800 nm) that can be used for analysis in various coagulation assays using chromogenic and immunologic methods [24]. Several studies have already confirmed that the CS-5100 can perform well in routine and some special coagulation assays, such as coagulation factor activity and D-dimer assay [1234]. Our study evaluated the performance of the CS-5100 system in routine coagulation assays as well as the measurements of FDP and plasma heparin and DOAC concentrations.
FDP is a fragment produced by plasmin-mediated degradation of brinogen or insoluble brin. Although FDP has low sensitivity and specificity for diagnosing disseminated intravascular coagulation, it is widely used as a predictive marker for brinolysis [11]. In this study, the CS-5100 coagulometer showed acceptable performance for measuring FDP. Correlation with the STA-R coagulometer was good. In Passing-Bablok regression analysis, the 95% CI of the slope included value one, indicating no significant difference between the two analyzers. However, the intercept revealed significant differences as the 95% CI did not include value zero. The reason why these two coagulation analyzers showed some differences might be due to differences in detection methods and reagents [12]. The STA-R analyzer uses a mechanical clot detection method, while the CS-5100 analyzer uses an optical clot detection method. For the currently used upper limit of reference range for FDP set at 5 µg/mL with the STA-R coagulometer, the corresponding level on CS-5100 system was 6.1 µg/mL. Therefore, a new cutoff point for decision of increased FDP will be needed for the CS-5100 system.
UFH is a highly sulfated glycosaminoglycan. The pentasaccharide sequence of UFH is responsible for its anticoagulant activity because this sequence binds to antithrombin [1314]. Due to variability in anticoagulant response to UFH among different patients, monitoring plasma UFH concentration should be performed using dose adjustment [13]. A therapeutic aPTT range of 1.5 to 2.5 times that of control has been widely used [13]. However, the clinical relevance of therapeutic aPTT range is uncertain because many biologic factors unrelated to heparin concentration can affect the results of aPTT. The sensitivity of aPTT to heparin also varies among different reagents and coagulometers. In addition, aPTT is not a standardized test [1315]. Therefore, anti-Xa calibrated with UFH is more reliable than aPTT for monitoring plasma concentration of UFH.
The molecular weight of LMWH is about one-third the molecular weight of UFH [13]. It is one of the commonly used parenteral anticoagulants and is applied as a fixed dose. Similar to UFH, the anticoagulant mechanism of LMWH is through antithrombin-mediated inhibition of coagulation factors. However, the number of pentasaccharide sequences of LMWH is fewer than that of UFH. More than half of the LMWH chains are too short to catalyze thrombin inhibition, but they can inactivate coagulation factor Xa [13]. Although routine monitoring of plasma level of LMWH is unnecessary, accurate dosing is recommended for patients with high risk of bleeding, such as the elderly, patients with obesity, patients with renal failure, and those who are pregnant [1617]. In these situations, the anti-Xa assay with LMWH calibrator is recommended [13]. In this study, the CS-5100 system showed good performance for monitoring plasma UFH and LMWH concentrations. Since UFH and LMWH are the most widely used parenteral anticoagulants, the CS-5100 coagulation analyzer is suitable for therapeutic anticoagulant monitoring by clinical laboratories.
DOACs target a certain coagulation factor or a step of the coagulation cascade [18]. Rivaroxaban and apixaban can directly inhibit factor Xa, while dabigatran inhibits thrombin directly. Routine monitoring of the concentrations of DOACs is not necessary because they have predictable pharmacodynamics and pharmacokinetics with fixed dose administration [1920], a wide on-therapeutic range, and few interactions with other drugs [21]. However, monitoring of DOACs might be useful in specific clinical situations and patient populations, such as pre-surgery or invasive surgery if the patient has received DOACs in the previous 24 hours, bleeding or recurrent thrombosis with DOAC treatment, suspicious overdose, hepatic or renal impairment, and extremely low or high body weights [2223]. Traditional coagulation tests are limited in terms of accuracy for determining plasma levels of DOACs. In comparison, high-performance liquid chromatography (HPLC), tandem mass spectrometry (MS/MS), and specific coagulation assays, such as anti-factor Xa and dilute thrombin time assay, can determine the concentrations of DOACs more accurately. However, HPLC and MS/MS have relatively long turnaround times and are expensive. In addition, they require a skilled technician [24] and are not widely available in many clinical laboratories. On the contrary, anti-Xa assays for rivaroxaban and apixaban and diluted thrombin time assay for dabigatran are suitable methods to measure concentrations of DOACs with specific calibrators and controls [2325]. The present data reveal that the CS-5100 system might be suitable for measuring plasma concentrations of DOACs in terms of precision. If other aspects of performance, such as linearity, carryover, and accuracy, are confirmed, the CS-5100 coagulometer might be useful for monitoring plasma concentrations of anticoagulants, not only for UFH and LMWH, but also for newly developed and increasingly used DOACs.
Our study has some limitations. First, DOACs were spiked into NPP obtained from healthy subjects instead of patient samples treated with DOACs. However, spiking of DOACs into NPP is a widely used method [1826]. Further performance evaluation and comparison with other coagulometers using patient samples treated with DOACs should be done. Second, direct thrombin inhibitor dabigatran was evaluated only for the aspect of precision performance. Pharmacologically active concentrations of dabigatran are achieved after oral administration [27]. Thus, dabigatran-spiked NPP was not appropriate for performing diluted thrombin time assay. Finally, the correlation between plasma concentration of anticoagulants and clinical efficacy or safety outcomes was not evaluated.
In conclusion, our study demonstrates that the CS-5100 coagulometer precisely measures the special coagulation parameter, FDP. The CS-5100 apparatus is also a suitable analyzer for monitoring the concentrations of UFH and LMWH, the most widely used parenteral anticoagulants. Furthermore, it might be useful to monitor plasma concentrations of DOACs. The CS-5100 system is a reliable coagulation analyzer for clinical laboratories.
Figures and Tables
Fig. 1
Linearity of (A) FDP, (B) UFH, (C) LMWH, (D) rivaroxaban, and (E) apixaban measured with the CS-5100 coagulometer.
Abbreviations: FDP, fibrinogen degradation product; UFH, unfractionated heparin; LMWH, low molecular weight heparin.
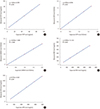
References
1. Ratzinger F, Schmetterer KG, Haslacher H, Perkmann T, Belik S, Quehenberger P. Evaluation of the automated coagulation analyzer CS-5100 and its utility in high throughput laboratories. Clin Chem Lab Med. 2014; 52:1193–1202.


2. Geens T, Vertessen F, Malfait R, Deiteren K, Maes MB. Validation of the Sysmex CS5100 coagulation analyzer and comparison to the Stago STA-R analyzer for routine coagulation parameters. Int J Lab Hematol. 2015; 37:372–381.


3. Chen L, Chen Y. Performance evaluation of the Sysmex CS-5100 automated coagulation analyzer. Clin Lab. 2015; 61:653–660.


4. Flieder T, Gripp T, Knabbe C, Birschmann I. The Sysmex CS-5100 coagulation analyzer offers comparable analytical performance and excellent throughput capabilities. Pract Lab Med. 2016; 6:38–47.



5. Camici GG, Steffel J, Akhmedov A, Schafer N, Baldinger J, Schulz U, et al. Dimethyl sulfoxide inhibits tissue factor expression, thrombus formation, and vascular smooth muscle cell activation: a potential treatment strategy for drug-eluting stents. Circulation. 2006; 114:1512–1521.


6. Clinical and Laboratory Standards Institute. Evaluation of precision of quantitative measurement procedures; approved guideline—third edition (EP05-A3). Wayne, PA: Clinical and Laboratory Standards Institute;2014.
7. Clinical and Laboratory Standards Institute. Evaluation of the linearity of quantitative measurement procedures: a statistical approach; approved guideline (EP06-A). Wayne, PA: Clinical and Laboratory Standards Institute;2003.
8. Clinical and Laboratory Standards Institute. Preliminary evaluation of quantitative clinical laboratory measurement procedures; approved guideline—third edition (EP10-A3-AMD). Wayne, PA: Clinical and Laboratory Standards Institute;2014.
9. Clinical and Laboratory Standards Institute. Measurement procedure comparison and bias estimation using patient samples; approved guideline—third edition (EP09-A3). Wayne, PA: Clinical and Laboratory Standards Institute;2013.
10. Clinical and Laboratory Standards Institute. Dening, establishing, and verifying reference intervals in the clinical laboratory; approved guideline—third edition (EP28-A3c). Wayne, PA: Clinical and Laboratory Standards Institute;2008.
11. McPherson RA, Pincus MR, editors. Henry's clinical diagnosis and management by laboratory methods. 23rd ed. St. Louis: Elsevier;2017.
12. Gardiner C, Kitchen S, Dauer RJ, Kottke-Marchant K, Adcock DM. Recommendations for evaluation of coagulation analyzers. Lab Hematol. 2006; 12:32–38.


13. Garcia DA, Baglin TP, Weitz JI, Samama MM. Parenteral anticoagulants: Antithrombotic Therapy and Prevention of Thrombosis, 9th ed: American College of Chest Physicians Evidence-Based Clinical Practice Guidelines. Chest. 2012; 141:e24S–e43S.
14. Vandiver JW, Vondracek TG. Antifactor Xa levels versus activated partial thromboplastin time for monitoring unfractionated heparin. Pharmacotherapy. 2012; 32:546–558.


15. Baluwala I, Favaloro EJ, Pasalic L. Therapeutic monitoring of unfractionated heparin - trials and tribulations. Expert Rev Hematol. 2017; 10:595–605.


16. Gouin-Thibault I, Pautas E, Siguret V. Safety prole of different low-molecular weight heparins used at therapeutic dose. Drug Saf. 2005; 28:333–349.


17. Thomas O, Lybeck E, Strandberg K, Tynngard N, Schott U. Monitoring low molecular weight heparins at therapeutic levels: dose-responses of, and correlations and differences between aPTT, anti-factor Xa and thrombin generation assays. PLoS One. 2015; 10:e0116835.


18. Samama MM, Contant G, Spiro TE, Perzborn E, Flem LL, Guinet C, et al. Evaluation of the prothrombin time for measuring rivaroxaban plasma concentrations using calibrators and controls: results of a multicenter eld trial. Clin Appl Thromb Hemost. 2012; 18:150–158.


19. Mueck W, Eriksson BI, Bauer KA, Borris L, Dahl OE, Fisher WD, et al. Population pharmacokinetics and pharmacodynamics of rivaroxaban--an oral, direct factor Xa inhibitor--in patients undergoing major orthopaedic surgery. Clin Pharmacokinet. 2008; 47:203–216.


20. Perzborn E, Strassburger J, Wilmen A, Pohlmann J, Roehrig S, Schlemmer KH, et al. In vitro and in vivo studies of the novel antithrombotic agent BAY 59-7939--an oral, direct Factor Xa inhibitor. J Thromb Haemost. 2005; 3:514–521.


21. Kubitza D, Becka M, Roth A, Mueck W. Absence of clinically relevant interactions between rivaroxaban--an oral, direct Factor Xa inhibitor-- and digoxin or atorvastatin in healthy subjects. J Int Med Res. 2012; 40:1688–1707.


22. Douxls J, Tamigniau A, Chatelain B, Gofnet C, Dogne JM, Mullier F. Measurement of non-VKA oral anticoagulants versus classic ones: the appropriate use of hemostasis assays. Thromb J. 2014; 12:24.



23. Lippi G, Favaloro EJ. Recent guidelines and recommendations for laboratory assessment of the direct oral anticoagulants (DOACs): is there consensus? Clin Chem Lab Med. 2015; 53:185–197.


24. Adcock DM, Gosselin R. Direct oral anticoagulants (DOACs) in the laboratory: 2015 review. Thromb Res. 2015; 136:7–12.


25. Harenberg J, Erdle S, Marx S, Kramer R. Determination of rivaroxaban in human plasma samples. Semin Thromb Hemost. 2012; 38:178–184.

