Abstract
Background and Purpose
Methods
Results
Figures and Tables
Fig. 1
Transaxial (upper and middle rows) and sagittal (lower row) 18F-FC119S PET images. A: A 77-year-old male patient clinically diagnosed with Alzheimer's disease (K-MMSE score=16) showed highly increased uptakes in the gray matter and no sulcal pattern. B: A 74-year-old female patient clinically diagnosed with vascular dementia (K-MMSE score=20) showed similar 18F-FC119S uptakes in the white and gray matter. C: A 73-year-old female healthy control (K-MMSE score=30) showed low 18F-FC119S uptakes throughout the brain and similar uptakes in the white and gray matter.
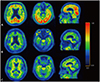
Table 1
Patient characteristics
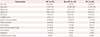
Data are n (%), mean±SD, or median [range] values.
*The following participants were included only in the safety analysis and excluded from the image analysis: 54-year-old female HC (K-MMSE score=29), 80-year-old female non-AD patient (K-MMSE score=22), 71-year-old female AD patient (K-MMSE score=15), 91-year-old female AD patient (K-MMSE score=0), and 75-year-old male AD patient (K-MMSE score=18), †Significantly different from HC (p<0.05).
AD: Alzheimer's disease, GCS: Glasgow Coma Scale, HC: healthy control, K-MMSE: Korean version of the Mini Mental State Examination, Non-AD: dementia other than AD.
Table 3
Sensitivity and specificity of visual assessments of different imaging modalities for Alzheimer's disease diagnoses
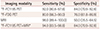
Table 4
Comparison of SUVRs for 18F-FC119S in HC, non-AD, and AD individuals
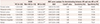
Data are mean±SD values, with 95% CI values within parentheses.
*Significantly different from HC (p<0.05), †Significantly different from non-AD (p<0.05).
AD: Alzheimer's disease, AUC: area under the ROC curve, HC: healthy control, Non-AD: dementia other than AD, ROC: receiver operating characteristic, SUVR: standardized uptake value ratio.
Table 5
Comparison of AEs following 18F-FC119S injection
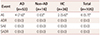
Data are n (%) values.
*The 95% CI values for AD, HC and Total are 0.4–14.7, 0.0–13.0, and 1.3–10.2 respectively.
AD: Alzheimer's disease, ADR: adverse drug reaction, AE: adverse event, HC: healthy control, Non-AD: dementia other than AD, SADR: serious adverse drug reaction, SAE: serious adverse event.
Acknowledgements
Notes
Author Contributions
Conceptualization: Inki Lee, Hae Ri Na, Byung Hyun Byun, Hee Seup Kil, Chansoo Park, Dae Yoon Chi, Jeong Ho Ha, Sang Moo Lim.
Data curation: Hae Ri Na, Su Yeon Park, Yu Keong Kim, Jun-Young Lee, Seon Hee Bu, Jung Hwa Kim, Jeong Ho Ha.
Formal analysis: Inki Lee, Byung Hyun Byun, Ilhan Lim, Byung Il Kim, Chang Woon Choi, In Ok Ko, Kyo Chul Lee, Kyeong Min Kim.
Funding acquisition: Kyo Chul Lee, Sang Moo Lim.
Investigation: Byung Hyun Byun, Su Yeon Park, Jeong Ho Ha.
Methodology: Hae Ri Na, Su Yeon Park, Yu Keong Kim, Jun-Young Lee, Seon Hee Bu, Jung Hwa Kim, Jeong Ho Ha.
Project administration: Inki Lee, Byung Hyun Byun, Sang Moo Lim.
Supervision: Jeong Ho Ha, Sang Moo Lim.
Validation: Inki Lee, Byung Hyun Byun, Ilhan Lim, Byung Il Kim, Chang Woon Choi, Sang Moo Lim.
Visualization: Inki Lee, Byung Hyun Byun.
Writing—original draft: Inki Lee, Hae Ri Na, Byung Hyun Byun, Jeong Ho Ha, Sang Moo Lim.
Writing—review & editing: Inki Lee, Hae Ri Na, Byung Hyun Byun, Ilhan Lim, Byung Il Kim, Chang Woon Choi, In Ok Ko, Kyo Chul Lee, Kyeong Min Kim, Su Yeon Park, Yu Keong Kim, Jun-Young Lee, Seon Hee Bu, Jung Hwa Kim, Hee Seup Kil, Chansoo Park, Dae Yoon Chi, Jeong Ho Ha, Sang Moo Lim.
Conflicts of Interest Sang Moo Lim received research grants and travel expenses from FutureChem Co., Ltd. Hee Seup Kil and Chansoo Park are employee of FutureChem Co., Ltd, Republic of Korea. Dae Yoon Chi is now an employee of FutureChem Co., Ltd as Chief executive officer. The other authors declare no conflicts of interest.
References








































