Abstract
PURPOSE
The aim of the study was to evaluate and compare the fracture resistance and modes of fracture of monolithic zirconia crowns with two preparation designs.
MATERIALS AND METHODS
Forty human maxillary first premolar teeth were extracted for orthodontic purposes and divided into two main groups (n=20): Group A: monolithic traditional zirconia; Group B: monolithic translucent zirconia. The groups were further subdivided into two subgroups (n=10): (A1, B1) shoulder margin design; (A2, B2) feather-edge margin design. Teeth were prepared with either a 1 mm shoulder margin design or a feather-edge margin design. The prepared teeth were scanned using a digital intraoral scanner. The crowns were cemented using self-adhesive resin cement. All cemented teeth were stored in water for 7 days and thermocycling was done before testing. All samples were subjected to compressive axial loading until fracture. The fractographic analysis was done to assess the modes of fracture of the tested samples.
RESULTS
The highest mean values of fracture resistance were recorded in kilo-newton and were in the order of subgroup A1 (2.903); subgroup A2 (2.3); subgroup B1 (1.854) and subgroup B2 (1.523). One-way ANOVA showed a statistically significant difference among the 4 subgroups. Concerning modes of fracture, the majority of samples in subgroups A1 and B1 were fracture of restoration and/or tooth, while in subgroups A2 and B2, the majority of samples fractured through the central fossa.
CONCLUSION
Even though all the tested crowns fractured at a higher level than the maximum occlusal forces, the shoulder margin design was better than the feather-edge margin design and the monolithic traditional zirconia was better than the monolithic translucent zirconia in terms of fracture strength.
All-ceramic restorations have been widely used because of their superior esthetic and biocompatibility,1 but the early ceramic materials had concerns about the clinical applications due to their relatively lower mechanical properties.2
With the development of CAD/CAM systems and rapid improvement in the mechanical properties of ceramic materials, zirconia became more popular.3 The zirconia is veneered with porcelain when used clinically to overcome poor optical properties.4 However, the chipping of the veneering layer has been reported.5 Thus, monolithic zirconia restorations were used to avoid the chipping of the veneering layer.6 The poor optical properties of traditional zirconia have been overcome by the development of translucent zirconia material; that developed for monolithic restorations is often referred as monolithic translucent zirconia.47 Translucent zirconia material has mechanical properties comparable to the traditional one in terms of fracture strength.8
However, it is possible to reduce the invasiveness of the tooth preparation with minimal preparation design by using high strength ceramic materials.91011
Feather-edge preparation design depends on a finish area rather than a definite finish line, and the presence of a finish line requires an excellent definition in the preparation phase before transferring it to the laboratory. All these problems are easier to be solved with feather-edge preparation design.12
Some authors have advocated the use of a vertical preparation with zirconia restorations.1013 Vertical preparation allows the use of zirconia restorations in periodontally treated tooth as abutment for fixed prosthesis because it preserves maximal amount of sound tooth structure after teeth preparations and is less invasive than horizontal margins.12 It can be also used in other clinical conditions like endodontically treated teeth, vital teeth in young individuals, and teeth that were affected by caries at the cervical one-third of the clinical crown.9
The aim of this in vitro study was to evaluate and compare the influence of preparation design on fracture resistance of different monolithic zirconia crowns and the modes of fracture of tested crowns.
From patients with the age of 18 – 22 years, 40 sound human maxillary first premolar teeth extracted for orthodontic purposes were collected in this in vitro study. Any attached soft tissue on the teeth was cleaned with hand scaler (DenTag, Terlano, Italy) and the teeth were rinsed with water. To minimize confounding variables, all teeth had normal occlusal anatomy and comparable and normal crown dimensions as measured by digital caliper (POWERFIX Profi, Model no. Z22855, OWIM, IAN 107580, Neckarsulm, Germany) and had no caries, restorations or cracks as visualized and examined under digital stereomicroscope (Koolertron, Hong Kong and Shenzhen, China) at (20×). The ones that showed any defects were excluded from the study. Then, the teeth were settled for 7 days in 0.1% thymol solution at room temperature to prevent fungal and bacterial growth. During all steps of the study, specimen dehydration was avoided.
With the aid of modified dental surveyor (Paraline, Dentaurum, Ispringen, Germany), each tooth sample was embedded in specially designed cylindrical rubber mold containing cold-cure acrylic (VERACRIL, AR350617 (powder), MA031018 (liquid), Antioquia, Colombia) parallel to the long axis to within 2 mm apical to the CEJ to simulate the biologic width.
Teeth were randomly divided into two groups (n = 20), according to the material used: Group A monolithic traditional zirconia (IPS e.max ZirCAD LT A3, W89335, Ivoclar Digital, Liechtenstein, Germany) and Group B monolithic translucent zirconia (IPS e.max ZirCAD MT Multi A3, X29480, Ivoclar Digital, Liechtenstein, Germany). They were further subdivided into two subgroups (n = 10) according to the type of margin design: subgroup A1 and B1 shoulder margin design and subgroup A2 and B2 feather-edge margin design.
Burs used for preparation of teeth samples were barrel-shaped trapezoid diamond bur (811 314 037, 804647, Komet, Siege, Germany) for occlusal reduction of all teeth samples; for subgroups A1 and B1, flat-end tapered fissure diamond bur for axial wall preparation (green ring) and finishing (red ring) (6847KR314 018, 950164, Komet, Siege, Germany) (8847KR 314 016, 806638, Komet, Siege, Germany); for subgroups A2 and B2, point-end tapered fissure diamond bur for axial wall preparation (green ring) and finishing (red ring) (6862 314 012, 111891, Komet, Siege, Germany) (8862 314 010, 665847, Komet, Siege, Germany).
For standardization purposes, teeth were prepared by the same operator to prevent the interexaminer differences. The vertical arm of the dental surveyor was modified to hold a turbine handpiece (DynaLED M600LGM4, OBD20053, NSK, Tokyo, Japan). Thus, the long axis of the bur was checked by using a protractor parallel with the long axis of the tooth sample, and this aided to transfer the taper of the bur to the axial walls of the tooth sample.
Standardized tooth preparation was performed on all teeth samples, which had a line drawn by a permanent color marker (Staedtler, Nuremberg, Germany) 1 mm above the cement-enamel-junction (CEJ) and a planar occlusal reduction with 4 mm height that was measured from the mesial side down to the line with a digital caliper. Specific criteria were: for subgroups A1 and B1, shoulder margin design of 1.0 mm depth with a total convergence of 6°; and for subgroups A2 and B2, feather-edge margin design with a total convergence of 4° (Fig. 1).
The digital image was taken for each tooth sample by using a powder-free digital scanner (CS 3500 intraoral scanner, Carestream Dental, Atlanta, GA, USA). The fabrication of monolithic zirconia crown restorations was done by using a 5-axis milling machine (In-Lab MC X5 milling machine, Sirona, Bensheim, Germany) with 80 µm spacer both radially and occlusally and 1 mm minimum occlusal thickness of the restoration according to the manufacturer instructions.
Sintering of zirconia crown restorations was done using sintering furnace (In Fire HTC Speed Furnace, Sirona, Bensheim, Germany) at 1540℃ to obtain the original size, strength, and color, and the intaglio surface were facing upward according to manufacturer recommendations. The cooling protocol for zirconia crowns was done according to a built-in program in the sintering furnace.14
In this in vitro study, the cementation was done by using resin cement (Rely X U200 self-adhesive resin cement, 3M ESPE, Seefeld, Starnberg/Upper Bavaria, Germany). At first, the crown was attached and held from the occlusal surface with the aid of a stamp (OptraStick, VL3752, Ivoclar Vivadent, Liechtenstein, Germany). The resin cement was injected into the internal surface of the crown using a disposable mixing tip up to thin even layer, and then custom made holding device was used to secure the zirconia crown on its respective tooth by a screw. A load sensor was attached to the device to hold an applied load of 5 Kg (approximately 50 N) and a piece of rubber on the arm facing the occlusal surface was to prevent damage from direct contact and simulate the cotton roll effect as in the clinical situation. Then, the specimens were stored in water for 7 days at 37℃.
The thermocycling was done on all specimens by using specially designed automatic thermocycling device. The thermocycling device consisted of two bunkers (one for cold and the other for hot water) and an arm that holds samples and encircles them on both bunkers. Digital thermostats were put in water to ensure that both bunkers reached the desired temperatures, which are 5° ± 0.5°C and 55° ± 0.5℃. When the device launched, a screen showed editable options to control the time and the speed of the movable arm, water temperature, and the number of cycles. The number of cycles was set to 500 with a dwell time of 30 seconds and, after the cycles, the specimens were air-dried.
The fracture strength of the zirconia crowns was tested by using a single load to failure test by a computer-controlled universal testing machine (Electronic universal testing machine, Laryee Technology, Beijing, China). Each zirconia crown was subjected to a compressive axial load centrally at the central fossa with 4 mm diameter round-end stainless steel indenter at a crosshead speed of 0.5 mm/min. 1 mm thickness specially designed rubber material was placed between the zirconia crown and the indenter to prevent crown damage from direct contact. All samples were loaded until a fracture occurred and a maximum breaking load was recorded automatically in Kilo-Newton (KN).
After completion of the fracture resistance test, all samples were examined under a digital stereomicroscope (Koolertron, Hong Kong and Shenzhen, China) at (40×) to assess the mode of fracture, according to the classification by Burke in 199915 (Table 1).
The fractographic analysis was done on two representative samples for each subgroup to examine the crack propagation direction under a scanning electron microscope (VEGA and VEGA III, TESCAN, Brno, Czech Republic) at different magnifications.
Statistical analysis of results was performed using the SPSS program (Statistical Package for Social Science, version 24.0; IBM Corp., Armonk, NY, USA). First, the Shapiro-Wilk test was used to show the normality of distribution of results and Levene's test was used to confirm the homogeneity of variances. One-way ANOVA test and Bonferroni test were used to analyze the data at a level of significance (P ≤ .05).
Shapiro-Wilk test indicated a normal distribution of results. Results showed the means and standard deviations of the fracture resistance of zirconia crowns, recorded in Kilo Newton. in the results were in the order from highest to lowest of subgroup A1 (2.903 ± .21721); subgroup A2 (2.3 ± .251); subgroup B1 (1.854 ± .19586) and subgroup B2 (1.523 ± .20945).
One-way ANOVA showed a statistically significant difference among the 4 subgroups (P > .05). A Bonferroni test was used for multiple comparisons among the subgroups (Table 2).
Regarding the modes of fracture, results for the subgroups A1 and B1 were irreparable fractures of crowns and/or teeth (code V), which represented 100% and 90%, respectively (Fig. 2). Results for the subgroups A2 and B2 were fracture through the midline of the crowns (code III) and also a midline fracture in which both the restoration and the tooth fractured together (code V), which represented 40% and 60%, respectively (Fig. 3). No samples from either subgroups showed fracture mode code I (Table 3).
In the last decades, the horizontal margin design was widely used and the academic world considered it as the gold standard, while the vertical margin design was used for conservation of tooth structure especially in periodontally compromised teeth.16
The zirconia material has gained popularity in recent years in comparison with other ceramic materials because of its superior mechanical properties.17 Regarding the esthetic outcome, veneering the zirconia with porcelain was used, but a common problem was a cohesive or adhesive failure of the veneering porcelain from the zirconia core.18 Due to the opaque appearance of zirconia with the above-mentioned cause, the manufacturers aimed to develop translucent zirconia to be used in many clinical situations.18 The monolithic zirconia became popular in clinical situations such as limited interocclusal distance.19
In this comparative study, we chose freshly extracted human natural teeth as abutments because the resistance to fracture of all-ceramic restorations was dependent on the elastic modulus of the substrate.2021 Additionally, using human teeth allowed for adhesive cementation of restorations and strength, which were closer to the clinical condition than other material abutments.22
In this study, we used zirconia (IPS e.max ZirCAD LT and MT Multi) from the same company for standardization purposes because the strength of translucent zirconia is dependent on brands.7
Teeth preparations for all-ceramic crown restorations are dependent on the material choice. The manufacturers of these materials stated that any ceramic materials' strength was determined by the material thickness and the design of the preparation.23 The design of the preparation, crown design, and method of manufacturing of crowns affect load at fracture so any alteration in one of these variables will cause changes in other variables.24
Regarding thermocycling in this study, the number of cycles was set to 500 and dwell time was 30 seconds. According to the international organization of standardization standard, 500 cycles in a range of temperature between of 5° ± 0.5℃ and 55° ± 0.5℃ was indicated as a proper aging procedure for zirconia.25
We found that monolithic traditional zirconia with shoulder margin design has higher fracture load than monolithic traditional zirconia with feather-edge margin design in both groups. It might be because the stress distribution pattern in the shoulder margin design as the circumferential shoulder withstands occlusal forces and results in less concentration of stress on axial walls.2627 Furthermore, an increase in the crown margin and axial wall thickness lead to fracture at a higher load. This was in accordance with previous studies concerning an increase in the crown margin thickness.2428
Another finding of this study showed that shoulder and feather-edge margin design with monolithic traditional zirconia had higher fracture load than shoulder and feather-edge with monolithic translucent zirconia; it was related to the 25% cubic phase that was found in the monolithic translucent zirconia (4 yttria-stabilized tetragonal zirconia polycrystal (4 Y-TZP)), which limits the transformation toughening mechanism (tetragonal to monoclinic phase transformation) found in monolithic traditional zirconia (3 yttria-stabilized tetragonal zirconia polycrystal (3 Y-TZP)).
Interestingly, we recorded a higher fracture resistance for monolithic traditional zirconia with feather-edge margin design, than monolithic translucent zirconia with shoulder margin design. This likely is due to the effect of the material more than the effect of the margin design.
Higher mechanical properties of the material with minimal preparation design is advantageous, since the result of this study revealed the fracture of all crowns at a higher level than the maximum mastication forces (850 N).29 This gives an indication that the feather-edge preparation design results in satisfactorily strong crowns especially for the monolithic traditional zirconia. Furthermore, feather-edge preparation design is related to biological benefits such as minimal removal of sound tooth structure and avoiding over-contouring of the margin of crown restoration, thus not compromising gingival and periodontal health.2430 Increasing thickness in the crown walls has a detrimental effect on the tooth, especially for endodontically treated teeth.31
The concentration of stress and the mode of fracture were determined by the nature of the single load to failure test. This was related to the direction of the load applied by the indenter as the fractographic analysis revealed that the crowns of all the tested samples fractured from the site of contact between the indenter and the occlusal surface of zirconia crowns. Furthermore, it was related to the complexity of the occlusal morphology of crown design such as the cusp angle and radius of fissure areas.32 Steeper cusps of upper premolar teeth make them more susceptible to fracture under occlusal loads.33
Majority of samples for the shoulder subgroups in this study showed an irreparable fracture of tooth (major samples) especially in the shoulder margin area of the substrate and/or restorations (code V). This is because an increase in reduction of tooth preparation leads to decrease in the fracture strength when compared with unprepared natural tooth.31 The fractographic analysis for the shoulder margin design in both subgroups revealed that the fracture origin started from the occlusal surface of zirconia crowns even though the shoulder withstood part of the occlusal stresses, and this was due to the contact between the indenter and the zirconia crowns.
Beuer et al. in 2008 discussed the pattern of stress distribution for the feather-edge preparation; during an increase in load, the zirconia coping distributed the force to the axial walls of the substrate without limitation by the margin, so the stress was concentrated on the occlusal surface in the fractographic analysis.26 Thus, the occlusal thickness is the most important aspect for the feather-edge subgroups because any decrease in the occlusal thickness results in weaker restorations. The load to fracture value was affected by occlusal thickness more than the cervical thickness.34 As the finding of this study, the majority of samples for feather-edge subgroups were fractured from the midline of crowns (code III) and a midline fracture occurred when both the restoration and the tooth fractured together (code V).
In the current study, a single (static) load to failure test was used after 7 days of water storage and thermocycling without mechanical cyclic loading. Therefore, the results of this comparative study can provide restricted information regarding the initial performance of zirconia crowns.
Within the limitations of this study, even though all the tested crowns fractured at a higher level than the maximum occlusal forces, the shoulder margin design was better than the feather-edge margin design and the monolithic traditional zirconia was better than the monolithic translucent zirconia in terms of fracture strength.
Figures and Tables
Fig. 2
Irreparable fracture of the monolithic zirconia crown and the tooth under a digital stereomicroscope (40×) for the shoulder margin design (Code V).
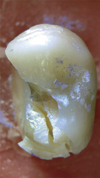
Fig. 3
Fracture of monolithic zirconia crown under a digital stereomicroscope (40×) for feather-edge margin design (Code III).
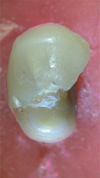
Fig. 4
Fractographic analysis. SEM for the shoulder subgroups revealed the crack origin (star) and the crack propagation direction (CPD) (arrow) which was started from the occlusal surface of the crown toward the shoulder margin. (A) Non-approximated view (19×), (B) Closer view (94×).
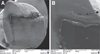
Fig. 5
Fractographic analysis. SEM for the feather-edge subgroups revealed the crack origin (star) and the crack propagation direction (CPD) (arrow) which was started from the occlusal surface of the crown toward the tooth. (A) Non-approximated view (25×), (B) Closer view (99×).
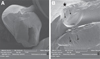
References
1. Beuer F, Stimmelmayr M, Gueth JF, Edelhoff D, Naumann M. In vitro performance of full-contour zirconia single crowns. Dent Mater. 2012; 28:449–456.
2. Zhang Y, Kelly JR. Dental Ceramics for Restoration and Metal Veneering. Dent Clin North Am. 2017; 61:797–819.
3. Monaco C, Caldari M, Scotti R. AIOP Clinical Research Group. Clinical evaluation of 1,132 zirconia-based single crowns: a retrospective cohort study from the AIOP clinical research group. Int J Prosthodont. 2013; 26:435–442.
4. Zhang Y. Making yttria-stabilized tetragonal zirconia translucent. Dent Mater. 2014; 30:1195–1203.
5. de Lima E, Meira JBC, Özcan M, Cesar PF. Chipping of veneering ceramics in zirconium dioxide fixed dental prosthesis. Curr Oral Health Rep. 2015; 2:169–173.
6. Mitov G, Anastassova-Yoshida Y, Nothdurft FP, von See C, Pospiech P. Influence of the preparation design and artificial aging on the fracture resistance of monolithic zirconia crowns. J Adv Prosthodont. 2016; 8:30–36.
7. Johansson C, Kmet G, Rivera J, Larsson C, Vult Von Steyern P. Fracture strength of monolithic all-ceramic crowns made of high translucent yttrium oxide-stabilized zirconium dioxide compared to porcelain-veneered crowns and lithium disilicate crowns. Acta Odontol Scand. 2014; 72:145–153.
8. Vichi A, Sedda M, Fabian Fonzar R, Carrabba M, Ferrari M. Comparison of contrast ratio, translucency parameter, and flexural strength of traditional and “Augmented translucency” zirconia for CEREC CAD/CAM system. J Esthet Restor Dent. 2016; 28:S32–S39.
9. Schmitt J, Wichmann M, Holst S, Reich S. Restoring severely compromised anterior teeth with zirconia crowns and feather-edged margin preparations: a 3-year follow-up of a prospective clinical trial. Int J Prosthodont. 2010; 23:107–109.
10. Poggio CE, Dosoli R, Ercoli C. A retrospective analysis of 102 zirconia single crowns with knife-edge margins. J Prosthet Dent. 2012; 107:316–321.
11. Schmitz JH, Cortellini D, Granata S, Valenti M. Monolithic lithium disilicate complete single crowns with feather-edge preparation design in the posterior region: A multicentric retrospective study up to 12 years. Quintessence Int. 2017; 601–608.
12. Patroni S, Chiodera G, Caliceti C, Ferrari P. CAD/CAM technology and zirconium oxide with feather-edge marginal preparation. Eur J Esthet Dent. 2010; 5:78–100.
13. Reich S, Petschelt A, Lohbauer U. The effect of finish line preparation and layer thickness on the failure load and fractography of ZrO2 copings. J Prosthet Dent. 2008; 99:369–376.
14. Dentsply Sirona. In fire HTC speed. Operating instructions. 2017. https://manuals.sirona.com/home.HomeDmsDocument.download.html?id=20054.
15. Burke FJ. Maximising the fracture resistance of dentine-bonded all-ceramic crowns. J Dent. 1999; 27:169–173.
16. Ramos RM, Clark D, Mazza M, Venuti P, Maiolino M, Kopanja S, Cirimpei V, Tawfik AA, Bordonali D, Acatrinei B, Sutradhar JC, Czerwinski M, Sienkiewicz A, Khademi J. The shoulderless approach a new rationale in prosthetic dentistry. Tomorrow Tooth J. 2017; 1:1–29.
17. Church TD, Jessup JP, Guillory VL, Vandewalle KS. Translucency and strength of high-translucency monolithic zirconium oxide materials. Gen Dent. 2017; 65:48–52.
18. Lawson NC, Burgess JO. Dental ceramics: a current review. Compend Contin Educ Dent. 2014; 35:161–166.
19. Jang GW, Kim HS, Choe HC, Son MK. Fracture strength and mechanism of dental ceramic crown with zirconia thickness. Procedia Eng. 2011; 10:1556–1560.
20. Wu XP, Li DS, Zhang J, Ma X, Zhao K, Zhang XP. Effects of different die materials on fracture and failure behaviors of all-ceramic crowns. Zhonghua Kou Qiang Yi Xue Za Zhi. 2009; 44:483–487.
21. Yucel MT, Yondem I, Aykent F, Eraslan O. Influence of the supporting die structures on the fracture strength of all-ceramic materials. Clin Oral Investig. 2012; 16:1105–1110.
22. Chitmongkolsuk S, Heydecke G, Stappert C, Strub JR. Fracture strength of all-ceramic lithium disilicate and porcelain-fused-to-metal bridges for molar replacement after dynamic loading. Eur J Prosthodont Restor Dent. 2002; 10:15–22.
23. Dolidze T, Bitarova I. Advantages and dısadvantages of E-max and Zırconıa crowns. Eur Sci J. 2017; 12:465–468.
24. Skjold A, Schriwer C, Øilo M. Effect of margin design on fracture load of zirconia crowns. Eur J Oral Sci. 2019; 127:89–96.
25. ISO/TR 11405. Dental materials - Guidance on testing of adhesion to tooth structure. Geneva; Switzerland: International Standards Organization (ISO);1994. Available at: https://www.iso.org/standard/19347.html.
26. Beuer F, Aggstaller H, Edelhoff D, Gernet W. Effect of preparation design on the fracture resistance of zirconia crown copings. Dent Mater J. 2008; 27:362–367.
27. Miura S, Kasahara S, Yamauchi S, Egusa H. Effect of finish line design on stress distribution in bilayer and monolithic zirconia crowns: a three-dimensional finite element analysis study. Eur J Oral Sci. 2018; 126:159–165.
28. Jalalian E, Rostami R, Atashkar B. Comparison of chamfer and deep chamfer preparation designs on the fracture resistance of zirconia core restorations. J Dent Res Dent Clin Dent Prospects. 2011; 5:41–45.
29. Sun T, Zhou S, Lai R, Liu R, Ma S, Zhou Z, Longquan S. Load-bearing capacity and the recommended thickness of dental monolithic zirconia single crowns. J Mech Behav Biomed Mater. 2014; 35:93–101.
30. Lang NP, Kiel RA, Anderhalden K. Clinical and microbiological effects of subgingival restorations with overhanging or clinically perfect margins. J Clin Periodontol. 1983; 10:563–578.
31. Rekow ED, Harsono M, Janal M, Thompson VP, Zhang G. Factorial analysis of variables influencing stress in all-ceramic crowns. Dent Mater. 2006; 22:125–132.
32. Shahrbaf S, van Noort R, Mirzakouchaki B, Ghassemieh E, Martin N. Fracture strength of machined ceramic crowns as a function of tooth preparation design and the elastic modulus of the cement. Dent Mater. 2014; 30:234–241.
33. Sornsuwan T, Ellakwa A, Swain MV. Occlusal geometrical considerations in all-ceramic pre-molar crown failure testing. Dent Mater. 2011; 27:1127–1134.
34. Nakamura K, Harada A, Inagaki R, Kanno T, Niwano Y, Milleding P, Örtengren U. Fracture resistance of monolithic zirconia molar crowns with reduced thickness. Acta Odontol Scand. 2015; 73:602–608.