This article has been
cited by other articles in ScienceCentral.
Abstract
BACKGROUND
Proper scaling of cardiac dimensions is of paramount importance in making correct decisions in clinical cardiology. The usual normalization of cardiac dimensions to overall body size assumes an isometric relationship. We sought to investigate these relationships to obtain the best allometric coefficient (AC) for scaling.
METHODS
Ninety-seven healthy volunteers were included. The dimensions to be scaled were the left atrial volume, the end-diastolic and end-systolic left ventricular volumes, and the diameter of the tricuspid annulus. A Bayesian statistical analysis was applied with isometric coefficients as priors.
RESULTS
The linear correlations between cardiac dimensions and body size were modest, ranging from 0.12 (-0.10–0.32) for the left atrial volume and height to 0.70 (0.58–0.80) for the end-diastolic volume and height. The ACs varied across the different cardiac dimensions and body size measurements. For the best linear relationships, the isometric coefficients were outside the 95% highest density interval of the posterior distribution for the left atrial volume-weight (AC: 0.7; 0.4–0.9) and end-diastolic volume-height (AC: 2.3; 1.7–2.9), whereas they were different from 1 for the left atrial volume-weight, end-diastolic volume, and diameter of the tricuspid annulus-body surface area (AC: 0.6; 0.3–0.8). Not scaling the cardiac dimensions to their corresponding ACs can lead to important errors in size estimations of cardiac structure.
CONCLUSIONS
The ACs found in this study are somewhat different from the corresponding isometric coefficients and often different from 1. This finding should be considered when normalizing cardiac structures to body size when making clinical decisions.
Keywords: Size, Scaling, Cardiac dimensions, Allometry
INTRODUCTION
Allometry designates the relationship between changes in shape and overall size.
1) The scaling of cardiac dimensions is a common practice between cardiologists, especially those involved in cardiac imaging. The goal of scaling is to normalize the cardiac dimensions to the patient's body size in order to distinguish disease states from normal variants. The calculation is often done by dividing the cardiac dimension by a measure of the patient's size, usually body surface area (BSA) to the power of 1. However, this calculation assumes a linear relationship between cardiac dimension and body size, which is not often true.
2) Moreover, the correct calculation using values of different dimensions (such as the left atrial volume and BSA) should take into account the theoretical isometric coefficient (3/2 in this example), which is not always the case.
3)
Scaling of the cardiac dimensions is often used in clinical practice. For example, it is used to diagnose heart failure with preserved ejection fraction (the left atrial volume scaled to BSA)
4) to indicate surgical treatment in patients with asymptomatic significant mitral regurgitation (end-systolic volume scaled to BSA)
5) or to indicate surgical treatment in a dilated tricuspid annulus (tricuspid annulus scaled to BSA).
5)6) Correct normalization of cardiac dimensions is of paramount importance in clinical practice: an improper evaluation of a cardiac dimension could lead to wrong medical decisions. We investigated the relationship between four common cardiac dimensions used to evaluate patient's condition (left atrial volume, left ventricular end-diastolic and end-systolic volumes, and tricuspid annulus diameter) with different common measures of body size (BSA, weight, and height) in order to find the appropriate allometric coefficient (AC) to the scale cardiac dimensions. Since a theoretical value exists (the corresponding isometric exponent), we applied a Bayesian approach with pre-defined priors for the calculations.
7)
METHODS
Healthy volunteers were included in the study. Volunteers were considered healthy if they were completely asymptomatic during normal daily activities, had a normal resting electrocardiogram, and had a normal transthoracic echocardiogram. Volunteers were excluded if the image quality of the transthoracic echocardiogram prevented a reliable measure of the 4 parameters analyzed.
Transthoracic echocardiograms were obtained with the patients in the left lateral position. All measurements were done from the conventional apical views. The left atrial volume was measured by the area-length method at the end-systole, excluding the pulmonary veins. The left ventricular end-diastolic and systolic volumes were measured from the 4 and 2 apical views using the biplane modified Simpson's rule. Papillary muscles were carefully excluded. The tricuspid annulus was measured from the 4 chamber apical view at the end-diastole as the distance from the septal to the lateral tricuspid annulus. BSA was calculated with Mosteller's formula. This study was carried out in accordance with the Code of Ethics of the World Medical Association (Declaration of Helsinki) for experiments involving humans and approved by our local ethics committee. All the participants provided written informed consent to participate in the study.
Statistics
The most common method to scale a cardiac dimension is to divide it by a measurement of body size, usually the BSA to an exponent of 1 (but not always). Since the isometric exponent is theoretically known (the dimension of the cardiac structure divided by the dimension of the body structure), this prior knowledge should be taken into consideration in the statistics. For this reason, we performed a Bayesian analysis of the data. The patients' age was not taking into consideration since it is not usually used to scale cardiac dimensions in clinical practice.
First, we did a log-transformation of the general formula used to scale the obtained data:
where X is the cardiac structure size, M is the body size measurement, and b is the allometric signal, which indicates whether the slope is linear (b = 1) or curvilinear. The posterior distribution of b and ρ (correlation coefficient) was simulated using standard Gibbs sampling (a multivariate normal draw for the betas and an inverse gamma draw for the conditional error variance).
8)
We chose the theoretical isometric coefficients depending on the magnitude being scaled as priors for β, with a standard error of 0.12 to account for some uncertainty. The Markov chain Monte Carlo algorithm was programmed to 10,000 iterations with a burn-in of 1,000 and a thin factor of 1.
The posterior distribution of ρ (the correlation coefficient) and b are shown as the mean and the 95% highest density interval (HDI). The interobserver variability was assessed in 30 random observations. Two independent observers measured the cardiac dimensions. The intraclass correlation coefficient was used to evaluate concordance. We considered a value of r > 0.8 as a good interobserver agreement. The statistical software R was used for handling the data with the packages MCMCpack, LearnBayes, and BayesianFirstAid.
RESULTS
Baseline characteristics
Ninety-seven individuals were included, of which 30 (30.9%) were men. Thirty-eight (39.2%) did not perform any kind of regular exercise. The mean and standard deviation of the body size measurements were weight of 69 ± 15 kg (range 43–124 kg), height of 166 ± 10 cm (range 146–190 cm), and BSA of 1.8 ± 0.2 m2 (range 1.35–2.32 m2). The mean age was 45 years (range 15-65 years).
Analysis of cardiac size
The results are shown in
Table 1. The theorical isometric coefficients and their prespecified grades of uncertainty are depicted (priors in the
Table 1), and the b
-values found after fitting the linear relationship between the logarithmic transformation of the x and y values. These b
-values (the slope of these linear correlations) are the ACs obtained from our data. The HDI of these b-values (the most credible values) are also shown. A point estimate outside the HDI is considered inappropriate to scale cardiac dimensions.
Table 1
Evaluation of cardiac size
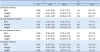
|
ρ |
b |
PE |
HDI |
Prior |
PE |
HDI |
Left atrial volume |
|
|
|
|
|
|
BSA |
0.38 |
0.21–0.56 |
1.5 (1.3–1.7) |
1.0 |
0.5–1.5 |
|
Weight |
0.42 |
0.24–0.58 |
1.0 (0.8–1.2) |
0.7 |
0.4–0.9 |
|
Height |
0.12 |
−0.10–0.32 |
3.0 (2.8–3.2) |
0.6 |
−0.5–1.7 |
LV end-diastolic volume |
|
|
|
|
|
|
BSA |
0.69 |
0.57–0.79 |
1.5 (1.3–1.7) |
1.5 |
1.1–1.8 |
|
Weight |
0.61 |
0.46–0.73 |
1.0 (0.8–1.2) |
0.8 |
0.6–1.0 |
|
Height |
0.70 |
0.58–0.80 |
3.0 (2.8–3.2) |
2.3 |
1.7–2.9 |
LV end-systolic volume |
|
|
|
|
|
|
BSA |
0.69 |
0.56–0.81 |
1.5 (1.3–1.7) |
1.7 |
1.0–2.3 |
|
Weight |
0.62 |
0.47–0.75 |
1.0 (0.8–1.2) |
0.8 |
0.5–1.2 |
|
Height |
0.68 |
0.55–0.80 |
3.0 (2.8–3.2) |
3.7 |
2.5–4.8 |
Tricuspid annulus |
|
|
|
|
|
|
BSA |
0.45 |
0.28–0.61 |
0.5 (0.3–0.7) |
0.6 |
0.3–0.8 |
|
Weight |
0.43 |
0.24–0.60 |
0.3 (0.1–0.5) |
0.3 |
0.2–0.5 |
|
Height |
0.26 |
0.05–0.45 |
1.0 (0.8–1.2) |
0.6 |
0.1–1.0 |
The linear correlations between the different measures of body size and the measures of cardiac dimensions were modest at best. The best linear correlation of each cardiac dimension is shown in
Figures 1 and
2. The AC did not coincide with the isometric coefficient in the majority of cases and is not even a credible value for the pairs of left atrial volume with weight and height and of the end-diastolic volume with height. The most common measure of body size used to scale cardiac dimensions in clinical practice is BSA with an exponent of 1. This value is outside the HDI for end-diastolic volume and length of the tricuspid annulus, and there is a very low correlation in the latter case (ρ = 0.38).
Figure 1
Bayesian correlation between cardiac structures and body size (left atrial volume and weight; LV end-diastolic volume and height). For details, see Table 1. LV: left ventricle.
Figure 2
Bayesian correlation between cardiac structures and body size (LV end-systolic volume and body surface area; tricuspid annulus diameter and body surface area). For details, see Table 1. LV: left ventricle.
To illustrate the importance of a correct scaling, we plotted the difference between a scaling of a theoretical left ventricular end-diastolic volume of 120 mL scaled to BSA and scaled to BSA
1.7 against BSA. This difference increases as the BSA increases, and the maximum occurs at a BSA between 2.0–2.2 m
2 on the order of 23 mL/m
2 (
Figure 3).
Figure 3
Difference between indexed left ventricular EDV to the BSA to power of 1 and 1.5 across different BSAs for a theoretical absolute value of 120 mL. BSA: body surface area, EDV: end-diastolic volume.
Interobserver variability
The intraclass correlation coefficients between two independent observers are depicted in
Table 2. As shown, there is very good agreement between both observers in the evaluation of cardiac dimensions.
Table 2
Analysis of agreement. Intraclass correlation coefficient between two independent observers

|
r (95% CI) |
Left atrial volume (mL) |
0.91 (0.80–0.96) |
Tricuspid annulus (mm) |
0.81 (0.57–0.91) |
LV end-diastolic volume (mL) |
0.94 (0.86–0.98) |
LV end-systolic volume (mL) |
0.88 (0.73–0.95) |
DISCUSSION
It is very intuitive that larger individuals would have larger hearts
9): it has been reported that body size accounts for up to 50% of adult left ventricular dimension variability.
10) Normalization of cardiac dimensions to body size has a very important application in making comparisons between patients of different sizes. This process leads to universal cut-off values that can be applied to all patients with heart diseases and can theoretically distinguish diseased from normal states. With this goal in mind, many clinical guidelines and leaders in cardiology recommend normalization of cardiac dimensions.
11)
The conventional method of scaling is based on two important presumptions: a linear relationship between cardiac size and body size and an allometric exponent of 1.
9) However, if the sizes of both the heart and body were isometric, the exponent of the body size would be the ratio between the dimensions of the numerator and denominator (1 for linear dimensions, 2 for surfaces, and 3 for volumes/masses). This information is very important since it is prior knowledge that should be taken into consideration. We performed Bayesian statistics to manage the data mainly for this reason.
To investigate the relationships between common measures of cardiac size and body sizes, we conducted this study on healthy individuals. The main findings were a modest or even low linear relationship between echocardiographic cardiac dimensions and common measures of body size, as well as an AC that is different from 1 and different from the theoretical isometric coefficient. Applying the coefficients obtained in this study provides very different estimation of cardiac size in normal individuals and thus in patients with heart diseases.
The linear relationship of the left atrial volume and tricuspid annulus diameter with BSA was very low. The r
2 values were 0.14 and 0.20, respectively. Thus, only 14% and 20% of the change in these cardiac dimensions were explained with the changes in body size. Moreover, the value of 1 was inside the 95% HDI, although with a broad estimation between 0.5 and 1. For the tricuspid annulus, 1 is not a credible value since the estimate is 0.6. This finding implies that the conventional scaling of these dimensions provides a wide margin of error. However, the left ventricular end-diastolic and end-systolic volumes had better linear correlations with BSA, but the ACs are far from 1 and on the order of 1.5 and 1.7, respectively (see
Table 1). Thus, a better scaling of these volumes would use BSA raised to a power of 1.5 or 1.7. The normalization using BSA
1 instead of BSA
1.5 or BSA
1.7 provides an overestimation of the left ventricle size that could prompt arguable clinical decisions.
Some studies have addressed this subject. In general, three-dimensional cardiac structures are indexed to weight or BSA. de Simone et al.
2) analyzed the left ventricular mass determined by echocardiography in healthy individuals and its relationship with common measures of body size. As expected, they found allometric exponents of 1, 1.5, and 2.7 for weight, BSA, and height, respectively. In accordance with our results, Gutgesell and Rembold
12) demonstrated that the left ventricular end-diastolic volume was related to BSA to a power of 1.5. Our study expands this concept to other important cardiac dimensions, such as the left atrial volume and tricuspid annulus diameter, and the results emphasize the importance of correct scaling of cardiac structures.
Limitations
Although a broad range of body sizes were included in the study, a larger sample would have increased the precision of the estimates. We included more women than men. Although allometric exponents could be different for women and men,
13) this was not a focus of our study, and dividing the entire group in two would reduce the sample size further and provide a broader range of the estimates. The results suggest that our ability to distinguish normal from diseased states based on cardiac dimensions can be improved. However, this does not imply that our capacity to discriminate patients with heart disease and worse outcomes is better as suggested in other studies.
14)
Conclusions
The relationship between cardiac dimensions and overall body size is not always linear. As such, the usual practice of scaling cardiac structures to the first power of a measure of body size does not always provide a reliable indexed measure, which can lead to erroneous clinical decisions. Specific trials should be done to determine if normalization to the allometrically correct power will improve the outcome of patients with heart diseases.