Abstract
The purpose of our study was to evaluate anti-AD potential of Cirsium maackii flowers. MeOH extract, CH2Cl2, EtOAc, and n-BuOH fraction of this flower notably inhibited BACE1 (IC50 = 76.47 ± 1.66, 22.98 ± 1.45, 8.65 ± 0.63, and 72.47 ± 3.04 µg/mL, respectively). β-amyrenone (49.70 mg) (1), lupeol acetate (1.43 g) (2), lupeol (1.22 g) (3), lupenone (23.70 mg) (4), β-sitosterol (1.01 g) (6), and β-sitosterol glucoside (13.00 mg) (7) from CH2Cl2, apigenin (100.20 mg) (8), luteolin (19.00 mg) (9), apigenin 7-O-glucuronide methyl ester (21.30 mg) (14), and tracheloside (53.70 mg) (5) from EtOAc, apigenin 5-O-glucoside (11.00 mg) (10), luteolin 5-O-glucoside (11.00 mg) (11) and apigenin 7-O-glucuronide (91.00 mg) (12) from n-BuOH, and luteolin 7-O-glucuronide (22.00 mg) (13) from H2O fraction were isolated. HPLC showed high levels of 8, 9 and 12 in MeOH extract (33.07 ± 0.07, 31. 44 ± 0.17 and 16.89 ± 0.33 mg/g, respectively), EtOAc (161.01 ± 1.78, 96.93 ± 0.34 and 73.38 ± 0.06 mg/g, respectively), and n-BuOH fraction (32.18 ± 0.33, 44.31 ± 0.32 and 105.94 ± 0.36 mg/g, respectively). Since, 3 and 9 are well-known BACE1 inhibitors, the anti-AD activity of C. maackii flower might be attributable to their presence.
Alzheimer's disease (AD) is a progressive neurodegenerative disease where brain regions, particularly cerebral cortex and hippocampus, are affected by deposition of amyloid-β (Aβ) plaques and neurofibrillary tangles accompanied by synaptic dysfunction and neurodegeneration.12 Increased proteolytic processing of β-amyloid precursor protein (APP) can lead to the production and accumulation of neurotoxic forms of Aβ in the brain.3 According to the amyloid cascade hypothesis, APP is generally cleaved by α-secretase and simultaneously processed by β- and γ-secretases. Disparity between production and clearance of Aβ peptide can result in aggregation of Aβ peptide into soluble oligomers and forms insoluble fibrils of beta-sheet conformation that are eventually deposited in diffused senile plaques.4 Beta-secretase 1, also known as beta-site amyloid precursor protein cleaving enzyme 1 (BACE1) is an enzyme that leads to the production of neurotoxic Aβ in the pathogenesis of AD. Due to the result disabling nature and chronicity of AD with high prevalence in older population and inability of available AD treatments to justify their costs, current AD treatments have been criticized because they fail to provide sufficient benefit.
Emerging researches have been directed toward BACE1 inhibitors due to their potential in lowering cerebral Aβ concentrations and treating as well as preventing AD.5 Moreover, BACE1 initiates the production of toxic Aβ that plays a crucial role in the early phase of AD pathogenesis. Due to its apparent rate limiting function, BACE1 appears to be a prime target to prevent and lower Aβ generation in AD.6
Cirsium maackii (C. maackii) is an herbaceous perennial plant of family Compositae. It is native to Korea.7 Roots or whole plant of Cirsii Radix et Herba have been used as a folk medicine to treat hemorrhage, diuresis, inflammation of the liver and kidney, and a variety of abdominal and intestinal disorders.8 Cirsium species have been found to exert various bioactivities, including anti-microbial,9 anti-proliferative,10 anti-diabetic,11 anti-oxidant,12 anti-inflammatory, 7 vasorelaxant,13 anti-cancer,14 and hepatoprotective15 effects. In addition, numerous phytochemicals, including flavonoids, phenolic acids,916 lignans, neolignans, sesquineolignans,1617 phenylacrylic acid esters, polyacetylenes,18 sterols, and triterpenes have been isolated from these species.1920
Walesiuk et al. have reported that Cirsium rivulare flowers extract shows pro-cognitive and its leaf extract possesses anxiolytic effect in a rat model.21 Kim and Kim have shown that, among methanol extracts of root, stem, leaves, and flowers of Cirsium japonicum, flowers and leaves have optimum peaks of luteolin, apigenin, and hispidulin when analyzed by high-performance liquid chromatography (HPLC).22
Our previous study has focused on inhibitory effects of C. maackii leaves on advanced glycation end products (AGEs).16 Considering mounting interest in assessing the anti-AD capacity of compounds from natural source and the ease of access to Cirsium species, the objective of the present study was to determine whether C. maackii flower could alleviate AD by evaluating its BACE1 inhibition effect and clarifying its activity through quantitative analysis of compounds present in its different extracts and fractions by HPLC.
BACE1 kit was obtained from PanVera Corp. (Madison, WI, USA). Ultra-pure grade water was used in this study. All chemicals and solvents used in this study were purchased from Sigma-Aldrich (St. Louis, MO, USA) unless otherwise stated. All solvents used in HPLC were of HPLC grade.
Flowers of C. maackii were collected from the southeast area of Gyeongsangnam-do Province, Korea, in 2016 and authenticated by Professor Jae Sue Choi (Pukyong national university, Korea). A voucher specimen (No.20160901) was deposited at the laboratory of Professor J.S. Choi.
Dried flowers of C. maackii (2.8 kg) were extracted four times with MeOH at 70 ℃. The final yield of MeOH extract was 168.28 g. The obtained extract was then suspended in water (H2O) and successively partitioned with dichloromethane (CH2Cl2), ethyl acetate (EtOAc), and n-butanol (n-BuOH) to yield CH2Cl2 (34.78 g), EtOAc (13.33 g), and n-BuOH (16.63 g) fractions, as well as H2O residue (102.00 g). The CH2Cl2 fraction was chromatographed over silica gel column and eluted with n-hexane:EtOAc 25:1 to EtOAc to obtain five subfractions. Repeated chromatography of subfraction 1 yielded β-amyrenone (49.70 mg) (1), lupeol acetate (1.43 g) (2), and lupenone (23.70 mg) (4) while subfraction 2 yielded lupeol (1.22 g) (3). β-sitosterol (1.01 g) (6) and β-sitosterol glucoside (13.00 g) (7) were obtained from subfractions 3 and 5, respectively. The EtOAc fraction was subjected to silica gel column chromatography and eluted with CH2Cl2: MeOH:H2O (7:1:0.1 to 3:1:0.1) to give seven different subfractions. Apigenin (100.20 mg) (8) was obtained as a precipitate from subfraction 2. Repetitive chromatography of subfractions 3, 4, and 5 over silica gel column with CH2Cl2:MeOH:H2O (10:2:0.1, 7:1:0.1, and 5:1:0.1) yielded luteolin (19.00 mg) (9), tracheloside (53.70 mg) (5), and apigenin 7-O-glucuronide methyl ester (21.30 mg) (14), respectively.
The n-BuOH fraction was proceeded to chromatography on Sephadex LH-20 column using 100% MeOH as a mobile phase solvent to yield eight different subfractions. Continual elution of subfraction 4 using EtOAc: MeOH:H2O (24:4:3) and RP-C18 column gave apigenin 7-O-glucuronide (91.00 mg) (12) whereas subfraction 7 chromatographed over silica gel column using EtOAc: MeOH:H2O (30:2:1) gave an insoluble precipitate on addition of MeOH. This precipitate was separated through filtration and later identified as luteolin 5-O-glucoside (11.00 mg) (11). Repetitive column chromatography of the supernatant over silica gel, RP-C18, and Sephadex LH-20 yielded apigenin 5-O-glucoside (11.00 mg) (10). Quarter amount of the H2O fraction (40 g) was subjected to Diaion HP-20 column and eluted with 100% H2O and 100% MeOH. Continuous silica gel, Sephadex LH-20, and MCI CHP-20P chromatography of 100% MeOH subfraction (7 g) with EtOAc:MeOH:H2O (24:4:3), 50% MeOH, and 30% MeOH gave luteolin 7-O-glucuronide (22.00 mg) (13).
EI-MS for C30H48O m/z (rel. int.): 409 [M-CH3] (4.59%), 218.25 (100%), 203.15 (34%), 189.20 (24.21%), 147.15 (11.88%).
EI-MS for C32H52O2 m/z (rel. int.): 468 [M+] (20.99%), 408.35 [M-CH3COO]+ (8.36%), 189.15 (100%).
1H-NMR (CD3OD, 600 MHz) δH 7.06 (1H, d, J = 8.3 Hz, H-5′), 6.85 (1H, d, J = 7.6 Hz, H-5), 6.70 (1H, d, J = 2.1 Hz, H-2), 6.68 (3H, d, H-6), 6.67 (2H, d, J = 2.1 Hz, H-2′/6′), 3.98 (2H, d, J = 8.9 Hz, H-9), 3.14 (1H, d, J = 13.7 Hz, H-7′), 2.88 (1H, d, J = 13.7 Hz, H-7′), 2.79 (1H, dd, J = 13.7, 5.52 Hz, H-7), 2.51 (1H, dd, J = 13.8, 9.63 Hz, H-7), 2.43 (1H, m). 13C-NMR (CD3OD, 150 MHz) δc 180.37 (C-9′), 150.61 (C-4′), 150.58 (C-4), 149.18 (C-3), 147.17 (C-3′), 133.34 (C-1), 131.71 (C-1′), 124.13 (C-6′), 122.23 (C-6), 117.82 (C-5′), 115.94 (C-2′), 113.96 (C-2), 113.25 (C-5), 102.85 (C-1″), 77.27 (C-8′), 77.84 (C-3″), 74.89 (C-2″), 71.78 (C-9), 71.30 (C-4″), 44.60 (C-8), 41.83 (C-7′), 32.18 (C-7).
1H-NMR (DMSO-d6, 600 MHz) δH 7.95 (2H, d, J = 8.9 Hz, H-2′/6′), 6.94 (2H, d, J = 8.8 Hz, H-3′/5′), 6.86 (1H, s, H-3), 6.85 (1H, d, J = 2.0 Hz, H-8), 6.47 (1H, d, J = 2.7 Hz, H-6), 5.32 (2H, d, J = 7.6 Hz, H-1″), 4.21 (1H, d, J = 9.6 Hz, H-5′), 3.67 (3H, s, OCH3). 13C-NMR (DMSO-d6, 150 MHz) δc 181.95 (C-4), 169.16 (C-6′), 164.30 (C-2), 162.39 (C-7), 161.46 (C-4′), 161.16 (C-5), 156.93 (C-9), 128.59 (C-2′/6′), 120.90 (C-1′), 116.00 (C-3′/5′), 105.96 (C-10), 103.08 (C-3), 99.28 (C-6), 94.60 (C-8), 75.38 (C-5′), 75.13 (C-2′), 72.69 (C-3′), 71.28 (C-4′), 51.95 (OCH3).
1H NMR (DMSO-d6, 600 MHz) δH 7.88 (1H, d, J = 9.0, 2.2 Hz, H-2′/6′), 6.91 (1H, d, J = 8.9 Hz, H-5′), 6.79 (1H, d, J = 2.0 Hz, H-8), 6.65 (1H, s, H-3), 6.73 (1H, d, J = 2.5 Hz, H-6), 4.70 (1H, d, J = 7.7 Hz, H-1″), 13C NMR (DMSO-d6, 150 MHz) δC 176.99 (C-4), 162.69 (C-2), 161.18 (C-7), 158.64 (C-9), 158.32 (C-5), 160.76 (C-4′), 115.87 (C-3′/5′), 128.08 (C-2′/6′), 121.16 (C-1′), 108.17 (C-10), 104.56 (C-3), 104.40 (C-6), 98.30 (C-8), 75.57 (C-3″), 77.57 (C-5″), 73.62 (C-2″), 69.66 (C-4″), 60.82 (C-6″).
1H NMR (DMSO-d6, 400 MHz) δH 7.85 (2H, d, J = 8.9 Hz, H-2′/6′), 6.88 (2H, d, J = 8.9 Hz, H-3′/5′), 6.77 (1H, d, J = 4.2 Hz, H-8), 6.40 (1H, s, H-3), 6.40 (1H, d, J = 2.0 Hz, H-6), 5.05 (1H, d, J = 6.6 Hz, H-1″). 13C NMR (DMSO-d6, 100 MHz) δC 181.92 (C-4), 172.45 (C-6″), 164.41 (C-2), 163.03 (C-7), 156.94 (C-9), 162.51 (C-5), 161.0 (C-4′), 116.16 (C-3′/5′), 128.44 (C-2′/6′), 120.16 (C-1′), 105.26 (C-10), 102.64 (C-3), 99.61 (C-6), 94.65 (C-8), 73.75 (C-3″), 76.48 (C-5″), 72.95 (C-2″), 71.98 (C-4″).
1H NMR (DMSO-d6, 600 MHz) δH 7.42 (1H, s, H-2′), 7.38 (1H, d, J = 8.7 Hz, H-6′), 6.86 (1H, d, J = 7.9 Hz, H-5′), 6.76 (1H, d, J = 1.4 Hz, H-8), 6.69 (1H, s, H-3), 6.40 (1H, d, J = 2.1 Hz, H-6), 5.09 (1H, d, J = 7.6 Hz, H-1″). 13C NMR (DMSO-d6, 150 MHz) δC 181.80 (C-4), 172.70 (C-6″), 162.95 (C-2), 164.46 (C-7), 160.98 (C-5), 156.89 (C-9), 150.34 (C-4′), 145.96 (C-3′), 118.96 (C-1′), 120.90 (C-6′), 116.04 (C-5′), 113.11 (C-2′), 105.23 (C-10), 102.83 (C-3), 99.54 (C-1″), 99.54 (C-6), 94.51 (C-8), 76.39 (C-3″), 77.48 (C-5″), 72.90 (C-2″), 73.80 (C-4″).
HPLC was performed on a Reverse phase JASCO HPLC system (Tokyo, Japan) equipped with a PU-1580 Intelligent HPLC pump, an LG-1580-04 quaternary gradient unit, a UV-1575 intelligent UV/Vis detector, a PG-1580-54 4-line degasser, and a CO-1560 intelligent column thermostat. Using a BORWIN chromatographic system (Le Fontanil, France), HPLC data were analyzed. Chromatography was accomplished on a Phenomenex C18 reverse phase column (Phenomenex, 4.6 × 250 mm, 5 µm) at 30 ℃ and monitored at 350 nm. An isocratic solvent system consisting of 0.2% phosphoric acid and MeOH at ratio of 55:45 was used for 80 min at the flow rate of 0.5 ml/min. For preparation of stock solutions, extracts and seven flavonoids were dissolved in 100% MeOH at concentrations of 2 mg/mL and diluted to 1, 0.5, and 0.25 µg/mL, respectively. After filtration through a centrifugal filter device (0.45 µm, Millipore Co., Bedford, MA, USA), 10 µL of each sample was injected. Calibration curves of all seven compounds were drawn with three standards at concentrations ranging from 62.5 to 500 µg/mL. Regression equations were calculated in the form of y = ax + b, where y and x corresponded to peak area and concentration, respectively. Relative quantities of seven flavonoids in the MeOH extract, EtOAc fraction, and n-BuOH fraction (mg/g of the extract) were calculated from each equation.
BACE1 inhibitory assay was carried out according to the supplied manual with selected modifications. Briefly, mixtures of 10 µL of assay buffer (50 mM sodium acetate, pH 4.5), 10 µL of BACE1 (1.0 U/mL), and 10 µL of the substrate (750 nM Rh-EVNLDAEFK-Quencher in 50mM, ammonium bicarbonate), and 10 µL of samples (final concentration, 250 µg/mL for the extracts/fractions) were incubated at 25 ℃ for 60 min in the dark. The proteolysis of two fluorophores (Rh-EVNLDAEFK-Quencher) by BACE1 was observed by exciting the mixtures at 545 nm and collecting the emitted light at 585 nm. Fluorescence was recorded with a microplate spectrofluorometer (Molecular Devices, Sunnyvale, CA, USA). The BACE1 inhibitory activity was expressed in terms of the IC50 value (µg/mL). Quercetin was used as a positive control.
Initially, the anti-AD potential of MeOH extract of C. maackii showed dose-dependent inhibition of BACE1 (16.50, 43.45, and 59.48% inhibition at 20, 50, and 100 µg/mL, respectively). Since its MeOH extract showed modest inhibition, it was further portioned into different fractions. BACE1 inhibitory potentials of different fractions were then determined. Results are summarized in Table 1 and Fig. 2. CH2Cl2 (86.58% at 50 µg/mL), EtOAc (65.45% at 25 µg/mL), and n-BuOH (59.63% at 100 µg/mL) fractions showed dose-dependent inhibition against BACE1, with IC50 values of 22.98 ± 1.45, 8.65 ± 0.63, and 72.47 ± 3.04 µg/mL, respectively, while IC50 value of quercetin, the standard, was 4.51 ± 3.63 µg/mL. Its H2O fraction did not show BACE1 inhibition at tested concentration (4 - 100 µg/mL), indicating the absence of BACE1 inhibitory potential. The inhibition order was EtOAc > CH2Cl2 > n-BuOH, demonstrating that EtOAc and CH2Cl2 fractions were remarkable BACE1 inhibitors.
Isolated compounds including β-amyrenone (1),23 lupeol acetate (2),24 lupeol (3), lupenone (4), β-sitosterol (6), β-sitosterol glucoside (7), apigenin (8), luteolin (9), tracheloside (5),25 apigenin 7-O-glucuronide methyl ester (14),26 apigenin 5-O-glucoside (10),27 luteolin 5-O-glucoside (11), apigenin 7-glucuronide (12),28 and luteolin 7-O-glucuronide (13)28 were identified via 1H and 13C NMR analysis as well as thin layer chromatographic analysis with standard. Data were compared with reported data. Structures of compounds are shown in Fig. 1.
In order to determine the part-specific preponderance of all the isolated flavonoids, HPLC quantitative analysis of MeOH extract, EtOAc fraction, and n-BuOH of C. maackii flowers was performed. Chromatograms, calibration curve, and quantification data are shown in Fig. 2 and Tables 2 and 3, respectively. The MeOH extract had the highest amount of apigenin (8) and luteolin (9) followed by apigenin 7-O-glucuronide (12). The calibration curve of for all seven compounds luteolin 5-O-glucoside (11), apigenin 5-O-glucoside (10), apigenin 7-O-glucuronide (12), apigenin 7-O-glucuronide methyl ester (14), luteolin (9), and apigenin (8) were drawn at 0.5, 0.25 and 0.125 mg/mL as shown in Table 2. Using linear regression equation obtained from the calibration curve, the number of individual compounds in 2 mg/mL extract and fractions were calculated. The relative amount of apigenin 7-O-glucuronide (12), apigenin 7-O-glucuronide methyl ester (14), luteolin (9), and apigenin (8) in the MeOH extracts from C. maackii flowers were 16.89 ± 0.33 mg/g, 6.66 ± 0.01 mg/g, 31.44 ± 0.17 mg/g, and 33.07 ± 0.07 mg/g, respectively. The yield of the EtOAc fraction for luteolin 5-O-glucoside (11), apigenin 5-O-glucoside (10), apigenin 7-O-glucuronide (12), apigenin 7-O-glucuronide methyl ester (14), luteolin (9), and apigenin (8) were 16.80 ± 1.01 mg/g, 8.26 ± 0.07 mg/g, 73.38 ± 0.06 mg/g, 27.03 ± 1.94 mg/g, 96.93 ± 0.34mg/g, and 161.01 ± 1.78mg/g indicating that apigenin (8) is abundant in EtOAc fraction. The contents of luteolin 7-O-glucuronide (13), apigenin 5-O-glucoside (10), apigenin 7-O-glucuronide (12), apigenin 7-O-glucuronide methyl ester (14), luteolin (9), and apigenin (8) in the n-BuOH fraction of flowers were 60.62 ± 0.83, 22.39 ± 0.84, 105.94 ± 0.36, 13.42 ± 0.10, 44.31 ± 0.32, and 32.18 ± 0.33 mg/g respectively, showing that the presence of apigenin 7-O-glucuronide (12) is abundant in n-BuOH fraction.
AD is one of detrimental neurodegenerative diseases in this aging society. It demands instant attention. Since some plant flowers have been reported to possess effect against inhibition of BACE1,29 this study was undertaken to evaluate the anti-AD potential of C. maackii flower together with quantification of its major compounds. Our results demonstrated the potential of C. maackii flower MeOH extract and its CH2Cl2 and EtOAc fractions in alleviating AD by inhibiting BACE1. In urge to identify the key compounds in our extract and fraction, we conducted HPLC and column chromatography analysis which revealed the presence of significant amounts of BACE1 inhibiting compounds lupeol (3) and luteolin (9) in CH2Cl2 and EtOAc fraction, respectively.
Our results showed that the EtOAc fraction had the highest BACE1 inhibiting activity. HPLC analysis revealed that two flavonoids, apigenin (8) and luteolin (9), had the highest amounts in the EtOAc fraction. These two compounds in the EtOAc fraction might have the highest contribution to the anti-AD property. As evidenced in our previous work,30 luteolin (9) has incredible BACE1 inhibiting effect, with IC50 of 1.95 ± 0.02 µM, which is higher even than the positive control, quercetin (3.17 ± 0.12 µM). Similarly, in the cerebral cortex of a mouse model, luteolin has been reported to exert neurovascular protection against Aβ25–35-induced amnesia, improve spatial learning and memory capabilities, modulate microvascular function, increase regional cerebral blood flow values, combat reactive oxygen species, improve cholinergic neuronal system, and increase brain-derived neurotrophic factor level and its receptor tyrosine kinase B expression.31 Wang et al. have also highlighted the potential effect of luteolin (9) on learning defects and hippocampal structure in AD.32 Moreover, apigenin (8) possesses scavenging effects against superoxide anion. It can improve anti-oxidative enzyme activity of superoxide dismutase and glutathione peroxidase and restore the neurotrophic ERK/CREB/BDNF pathway in the cerebral cortex, suggesting its ability to ameliorate AD-associated learning and memory impairment by relieving Aβ burden.33 Since contents of luteolin (9) and apigenin (8) in EtOAc fraction were the highest, apigenin (8) might be able to synergize the BACE1 inhibitory activity of luteolin in the EtOAc fraction of C. maackii flower.
CH2Cl2 fraction also showed a notable degree of inhibition against BACE1. Column chromatography of C. maackii flower revealed that this fraction was rich in lupeol (3) and lupeol acetate (2). Our previous report has revealed that lupeol (3) could effectively inhibit BACE1 enzyme, with IC50 of 5.12 ± 0.30 µM.34 In addition, it has been reported that lupeol (3) can restore Aβ1–42 induced behavioral and biochemical abnormalities in rat brain.35 Thus, the activity of CH2Cl2 fraction of C. maackii flower against BACE1 might be correlated with the inhibitory activity of lupeol (3).
Emerging evidences suggest a close association between oxidative stress and neurotoxicity of amyloid fibrils.36 Jung et al. have shown convincing activity of C. maackii flower against oxidative mediators including 2,2-diphenyl-1-picrylhydrazyl (DPPH), peroxynitrite (ONOO−), reactive oxygen species (ROS), and rat lens aldose reductase (RLAR) inhibition, thereby suggesting its anti-oxidative potential.8 Furthermore, its EtOAc fraction was highly active in all tested parameters while CH2Cl2 fraction was active against ONOO− only.8 Since luteolin (9) has been reported to possess potent RLAR and DPPH inhibiting activity,37 the anti-BACE1 activity of EtOAc fraction of C. maackii flower might be associated with the anti-oxidative potential of luteolin (9) which had the highest content in the EtOAc fraction.
Conversely, n-BuOH fraction of C. maackii flower had the modest activity compared to the two other nonpolar fractions. HPLC analysis showed that two glucuronides (apigenin 7-O-β-D-glucuronide (12) and luteolin 7-O-β-Dglucuronide (13)) had high contents. Iakovleva et al. have reported that luteolin, but not luteolin 7-O-β-D-glucoside (13), can attenuate cytotoxic response of cultured neuronal cells to transthyretin, a plasma protein with amyloidogenic property, and rescue the phenotype of a Drosophila melanogaster model.38 The presence of glucoronidated forms of apigenin (8) and luteolin (9) in a large amount in the n-BuOH fraction might have contributed to the lower activity of this fraction.
To the best of our knowledge, this is the first study to isolate secondary metabolites from C. maackii flowers through column chromatography. This is the first report on the isolation of β-amyrenone (1), lupeol acetate (2), lupeol (3), lupenone (4), β-sitosterol (6), and β-sitosterol glucoside (7) as non-polar constituents of C. maackii flower. Previously, Jung et al. have performed HPLC analysis of C. maackii flower and showed the presence of apigenin (8), luteolin (9), apigenin 7-O-glucuronide (12), and luteolin 5-O-glucoside (11) in its MeOH extract.8 In the present study, we were able to isolate these compounds together with apigenin 7-O-glucuronide methyl ester (14), tracheloside (5), apigenin 5-O-glucoside (10), and luteolin 7-O-glucuronide (13) from C. maackii flowers. Although the presence of a rare flavonoid, apigenin 5-O-glucoside (10), has been long described by Iwashina et al. in Japanese Cirsium species by principal component and UV spectra analysis, this is the first chromatographic separation and quantification of this compound from C. maackii.39 Additionally, using HPLC, we were able to quantify amounts of compounds in the MeOH extract as well as in EtOAc and n-BuOH fractions.
Flowering part of a plant is the most underexploited part compared to other plant parts such as leaves, root, and stem. During cultivation of C. maackii plant, the flowering part might be used as a source of important bioactive molecules or to be developed as a nutraceutical. Flowers of C. maackii are available plenteously during the flowering season at low cost or free of cost, suggesting that the thistle flower not only has aesthetic property, but also therapeutic potential to ameliorate AD.
In conclusion, the presence of major reference compounds, lupeol (3) and luteolin (9), from C. maackii flowers have potential in the treatment of AD as the fraction containing them exhibited anti-BACE1 activity. Our current findings suggest that flowers of C. maackii might have therapeutic potential for treating patients with AD. Since these results were based on in vitro tests, further experiments are needed to verify considering the mechanism of compounds isolated from C. maackii flowers.
Figures and Tables
Fig. 2
HPLC chromatograms of MeOH extract and different fractions of Cirsium maackii flower. (A) MeOH extract, (B) EtOAc fraction, (C) n-BuOH fraction along with (D) standard compounds: peak 1 (RT: 9.84 min, compound 11), peak 2 (RT: 12.57 min, compound 13), peak 3 (RT: 13.60 min, compound 10), peak 4 (RT: 17.74 min, compound 12), peak 5 (RT: 33.87 min, compound 14), peak 6 (RT: 42.38 min, compound 9), peak 7 (RT: 67.93 min, compound 8).
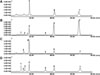
Acknowledgments
This research was supported by Research Grant of Pukyong National University (Granted in 2019).
References
1. Reitz C. Int J Alzheimers Dis. 2012; 2012:369808.
2. Singh SK, Srivastav S, Yadav AK, Srikrishna S, Perry G. Oxid Med Cell Longev. 2016; 2016:7361613.
3. Fedele E, Rivera D, Marengo B, Pronzato MA, Ricciarelli R. Mech Ageing Dev. 2015; 152:1–4.
4. Kumar A, Singh A. Ekavali Pharmacol Rep. 2015; 67:195–203.
5. Das B, Yan R. CNS Drugs. 2019; 33:251–263.
6. Yan R, Vassar R. Lancet Neurol. 2014; 13:319–329.
7. Shin MS, Park JY, Lee J, Yoo HH, Hahm DH, Lee SC, Lee S, Hwang GS, Jung K, Kang KS. Bioorg Med Chem Lett. 2017; 27:3076–3080.
8. Jung HA, Kim YS, Choi JS. Food Chem Toxicol. 2009; 47:2790–2797.
9. Nazaruk J, Jakoniuk P. J Ethnopharmacol. 2005; 102:208–212.
10. Sahli R, Rivière C, Dufloer C, Beaufay C, Neut C, Bero J, Hennebelle T, Roumy V, Ksouri R, Quetin-Leclercq J, Sahpaz S. T. Evid Based Complement Alternat Med. 2017; 2017:7247016.
11. Yin J, Heo SI, Wang MH. Nutr Res Pract. 2008; 2:247–251.
12. Nazaruk J, Wajs-Bonikowska A, Bonikowski R. Chem Nat Compd. 2012; 48:8–10.
13. Kim EY, Jho HK, Kim DI, Rhyu MR. J Ethnopharmacol. 2008; 116:223–227.
14. Liu S, Zhang J, Li D, Liu W, Luo X, Zhang R, Li L, Zhao J. Nat Prod Res. 2007; 21:915–922.
15. Ma Q, Guo Y, Luo B, Liu W, Wei R, Yang C, Ding C, Xu X, He M. Nat Prod Res. 2016; 30:1824–1829.
16. Jung HA, Park JJ, Min BS, Jung HJ, Islam MN, Choi JS. Asian Pac J Trop Med. 2015; 8:1–5.
17. Könye R, Tóth G, Sólyomváry A, Mervai Z, Zürn M, Baghy K, Kovalszky I, Horváth P, Molnár-Peri I, Noszál B, Béni S, Boldizsár I. Fitoterapia. 2018; 127:413–419.
18. Lai WC, Wu YC, Dankó B, Cheng YB, Hsieh TJ, Hsieh CT, Tsai YC, El-Shazly M, Martins A, Hohmann J, Hunyadi A, Chang FR. J Nat Prod. 2014; 77:1624–1631.
19. Chung MJ, Lee S, Park YI, Lee J, Kwon KH. Life Sci. 2016; 148:173–182.
20. Luan N, Wei WD, Wang A, Wu XL, Qi Y, Li JJ, Zheng JQ, Shang XY. J Asian Nat Prod Res. 2016; 18:1015–1023.
21. Walesiuk A, Nazaruk J, Braszko JJ. J Ethnopharmacol. 2010; 129:261–266.
22. Kim SJ, Kim GH. J Food Sci Nutr. 2003; 8:330–335.
23. Quintao NL, Rocha LW, Silva GF, Reichert S, Claudino VD, Lucinda-Silva RM, Malheiros A, De Souza MM, Filho VC, Bresolin TM, Machado M, Wagner TM, Silva CM. BioMed Res Int. 2014; 2014:636839.
24. Fernández-Martínez E, Jiménez-Santana M, Centeno-Álvarez M, Torres-Valencia JM, Shibayama M, Cariño-Cortés R. Pharmacogn Mag. 2018; 13:S860–S867.
25. Johansen KT, Wubshet SG, Nyberg NT, Jaroszewski JW. J Nat Prod. 2011; 74:2454–2461.
26. Mostafa NM, Eldahshan OA, El-Beshbishy HA, Singab AN. Med Aromat Plant (Los Angles). 2017; 6:1000297.
27. Veit M, Geiger H, Czygan FC, Markham KR. Phytochemistry. 1990; 29:2555–2560.
28. Ozgen U, Sevindik H, Kazaz C, Yigit D, Kandemir A, Secen H, Calis I. Molecules. 2010; 15:2593–2599.
29. Liu Q, Fang J, Wang P, Du Z, Li Y, Wang S, Ding K. Int J Biol Macromol. 2018; 107:112–120.
30. Choi JS, Islam MN, Ali MY, Kim YM, Park HJ, Sohn HS, Jung HA. Arch Pharm Res. 2014; 37:1354–1363.
31. Liu R, Gao M, Qiang GF, Zhang TT, Lan X, Ying J, Du GH. Neuroscience. 2009; 162:1232–1243.
32. Wang H, Wang H, Cheng H, Che Z. Mol Med Rep. 2016; 13:4215–4220.
33. Zhao L, Wang JL, Liu R, Li XX, Li JF, Zhang L. Molecules. 2013; 18:9949–9965.
34. Koirala P, Seong SH, Jung HA, Choi JS. Asian Pac J Trop Med. 2017; 10:1117–1122.
35. Kaundal M, Akhtar M, Deshmukh R. J Neurol Neurosci. 2017; 8:195.
36. Chakraborty S, Basu S. Int J Biol Macromol. 2017; 103:733–743.
37. Hwang SH, Wang Z, Guillen Quispe YN, Lim SS, Yu JM. J Diabetes Res. 2018; 2018:3276162.
38. Iakovleva I, Begum A, Pokrzywa M, Walfridsson M, Sauer-Eriksson AE, Olofsson A. T. PLoS One. 2015; 10:e0128222.
39. Iwashina T, Ito T, Ootani S. Ann Tsukuba Bot Gard. 1989; 8:15–19.