Abstract
Macrophages play essential roles in innate immune responses by producing various immune mediators. Therefore, modulating macrophage function is an attractive strategy to treat immune disorders. Aralia cordata var. continentalis (AC), known as “Dokwhal” in Korea, possesses various biological and medicinal functions, including immunomodulation. The present study investigated the effect of the hot water extract of AC (HAC) on RAW264.7 murine macrophages. When these cells were treated with HAC, nitric oxide production and inducible nitric oxide synthase expression was induced dose-dependently. In addition, HAC treatment triggered the secretion of innate immune cytokines, such as TNF-α and IL-6. Phagocytosis, measured by FITC-dextran internalization showed that HAC stimulated the phagocytic activity of macrophages. Furthermore, HAC promoted the production of reactive oxygen species in RAW264.7 cells, determined by CM-H2DCFDA. In addition, the immunoblot analysis of intracellular signaling proteins revealed that NF-kB and MAPK signaling pathways, which are important signaling mediators of inflammation, are upregulated by HAC. In conclusion, these findings suggested that HAC can stimulate macrophage activity, and NF-kB and MAPK signaling pathways might be involved in the immunostimulatory effects of HAC.
Some traditional herbal medicines are thought to strengthen the body's resistance to illness by stimulating the immune system. Aralia cordata var. continentalis (AC), known as “Dokwhal” in Korea, is classified as a shrub belonging to the Araliaceae family (1). It has been used as an Asian traditional herbal medicine to treat inflammatory disorders, including rheumatism, lumbago, and lameness, and to relieve pain (2). Recent studies have highlighted the antinociceptive and anti-inflammatory effects of AC, as well as its protective effect against carbon tetrachloride-induced hepatotoxicity (3,4,5). It was also found that the hot water extract of AC (HAC) protected mice from Listeria monocytogenes infection by upregulating the innate immune response (6). The present study was designed to investigate the effect of HAC on the RAW264.7 mouse macrophage cell line and elucidate the molecular mechanisms involved in its biological function.
Macrophages can not only initiate the innate immune response but also contribute to clearing pathogens and eliciting inflammation (7). Moreover, macrophages serve as an essential interface between innate and adaptive immunity by releasing inflammatory mediators, such as nitric oxide (NO), reactive oxygen species (ROS), prostaglandins, cytokines, chemokines, and growth factors (8, 9). It is known that NO, produced by inducible nitric oxide synthase (iNOS), plays an important role in the various pathophysiological responses, including inflammation, neoplastic disease, liver cirrhosis, diabetes, and killing of infected cells, tumor cells, and microorganisms (10). In addition, ROS produced by macrophages also serve as key components to eliminate intracellular bacteria (11, 12). Upon activation, macrophages release large amounts of interleukin-6 (IL-6), tumor necrosis factor-alpha (TNF-α), and other inflammatory cytokines, which enhance the inflammatory response and orchestrate protective immune responses to pathogens (13). Immunostimulators, produced by macrophages, are mediated mainly by two well-known intracellular signaling pathways, nuclear factor-kappa B (NF-κB) and mitogen-activated protein kinase (MAPK) (14, 15).
In this study, the immunostimulatory effect of HAC was investigated in RAW264.7 macrophages. First, it was determined whether HAC treatment could induce the production of NO, ROS, and proinflammatory cytokines that are important mediators to clear pathogens and enhance inflammatory responses. Second, the phagocytic activity of macrophages was examined in the presence of HAC. Finally, the intracellular signaling pathways responsible for the stimulatory actions of HAC were determined in murine RAW264.7 macrophages.
Lipopolysaccharide (LPS) (Escherichia coli O111:B4), sulfanilamide, N-(1-naphthyl) ethylenediamine dihydrochloride, phosphoric acid, 3-(4, 5-dimethylthiazol-2-yl)-2, 5-diphenyltetrazolium bromide (MTT), and FITC–dextran (40,000 Da) were purchased from Sigma–Aldrich Co (St. Louis, MO, USA). The primary antibodies used were obtained from Cell Signaling Technology (Danvers, MA, USA), except for β-actin (AbFrontier Company, Seoul, Korea). The secondary antibodies were purchased from Thermo Scientific (Logan, UT, USA). TNF-α and IL-6 ELISA kit, and PE-conjugated anti-CD11b antibody were purchased from eBioscience Inc. (San Diego, CA, USA).
The HAC was prepared as described earlier (6). Possible endotoxin contamination was checked using the Pierce® LAL Chromogenic Endotoxin Quantitation kit (Thermo Fisher Scientific, Waltham, MA, USA). The endotoxin concentration was less than 2.5 EU/mg, which is considered to be insignificant for various immunostimulators (16, 17).
Murine RAW264.7 monocytes/macrophages were purchased from the Korean Cell Line Bank (KCLB, Seoul, Korea) and maintained in Dulbecco's modified Eagle's medium (DMEM, Thermo Scientific) containing 10% FBS (Thermo Scientific) and 1% penicillin-streptomycin solution (Thermo Scientific). Cells were maintained at 37℃ in 5% CO2 humidified atmosphere, and experiments were conducted on cells at approximately 70–80% confluence.
Cell viability was measured using MTT solution. This assay is based on the reducing activity of mitochondria in living cells, which change from an oxidized form (soluble) to a reduced form (insoluble). MTT solution was added to each well, and after 3 h, the medium was discarded. The formazan formed in the wells was dissolved with dimethyl sulfoxide. The intensity of the purple color was measured, which is proportional to living cells, and expressed as a percentage of the control.
Cells were treated with HAC for 24 h, and nitrite accumulation in the culture medium was assessed as an indicator of NO production using the Griess method, as previously described (18). Briefly, the medium from treated cells was mixed with Griess reagent (equal volumes of 1% sulfanilamide containing 5% phosphoric acid with 0.1% N-(1-naphthyl) ethylenediamine dihydrochloride) and incubated at room temperature for 10 min. Absorbance at 570 nm was measured using a microplate reader (BioTek, Winooski, VT, USA), with sodium nitrite as the standard.
After treatment with HAC, RAW264.7 cells were washed with PBS twice and treated with 20 µM CM-H2DCFDA (Invitrogen, Carlsbad, CA, USA) at 37℃ for 30 min in the dark. Cells were washed, harvested, and analyzed using a flow cytometer (BD Accuri™ C6, BD Biosciences, San Jose, CA, USA).
RAW264.7 cells were treated with the indicated concentration of HAC and then incubated with DMEM containing FITC-dextran (1 mg/ml) for 6 h followed washing the cells. Uptake of FITC-dextran by RAW264.7 cells was analyzed by flow cytometry.
Protein samples of RAW264.7 cells were separated by SDS-PAGE and transferred to nitrocellulose membranes. The membrane was blocked with 5% skim milk in TBS with 0.025% Tween 20 (TBST) and probed with an appropriate primary antibody in 5% BSA in TBS overnight at 4℃. Afterward, the membrane was incubated with HRP-conjugated secondary antibody and developed by using SuperSignal West Femto Maximum Sensitivity Substrate (Thermo Scientific).
The IL-6 and TNF-α levels were determined by using a commercially available ELISA kit from eBioscience, Inc. Briefly, the samples were added into a 96-well plate coated with affinity-purified specific antibody for mouse IL-6 and TNF-α. An enzyme-linked detection antibody specific for IL-6 and TNF-α was added and left to react for 2 h, followed by washing to remove any unbound antibody reagent. The intensity of the color detected at 450 nm was measured after the addition of a substrate solution and was proportional to the amount of IL-6 and TNF-α produced.
Data are expressed as mean ± SEM. Statistical analysis was performed using GraphPad Prism, version 5.0 (GraphPad Software, San Diego, CA, USA). P-values were assessed using the unpaired, two-tailed Student's t-test (α = 0.05) at *p<0.05, **p<0.01 and ***p<0.001 levels of significance.
The cytotoxicity of HAC was first evaluated to find a suitable working concentration without detrimental effects on RAW264.7 macrophages. RAW264.7 cells were treated with various concentrations of HAC for 24 h, and the cell viability was measured by the MTT assay. As shown in Fig. 1A, HAC did not cause any cytotoxic effects up to 500 µg/ml. It is well-known that LPS-stimulated macrophages produce NO, an important molecule that participates in the early defense mechanisms against pathogens. Here, we found that HAC treatment also activated macrophages, leading to NO secretion in a dose-dependent manner (Fig. 1B). NO is synthesized by nitric oxide synthase (NOS) from L-arginine, and iNOS is the most important enzyme to trigger NO production, so we examined iNOS protein expression in LPS-or HAC-treated cell lysates. Fig. 1C shows that iNOS expression was significantly upregulated when cells were treated with LPS and, similarly, iNOS protein levels were increased with increasing HAC concentration. These results indicate that HAC stimulated macrophages to produce via iNOS induction. It was confirmed that the effect of HAC on NO production is not due to contamination of endotoxins (data not shown).
Macrophage activation is accompanied by morphological alteration. To determine whether HAC could trigger morphological changes, the RAW264.7 cells were analyzed by light microscopy. Under microscopic observation, most RAW264.7 cells cultivated without HAC appeared small and round, and some were spindle-like (Fig. 2A). When cells were activated by 1 µg/ml LPS for 24 h, the cells were expanded and showed lamellipodia extension. Cells treated with HAC showed morphological changes similar to those treated with LPS. These data provided further evidence, in addition to NO production, for the stimulatory effect of HAC on macrophages.
Activated macrophages secrete various cytokines and amplify the innate immune response via recruiting more immune cells to the infected area. TNF-α and IL-6 are the major inflammatory cytokines and are mainly produced by macrophages. RAW264.7 cells were treated with HAC or LPS, and the concentration of IL-6 and TNF-α in the culture supernatant was measured by using an ELISA kit after 24 h. As expected, HAC significantly increased the release of IL-6 and TNF-α dose-dependently (Fig. 2B), and the cytokine levels of HAC-treated macrophages at 500 µg/ml were comparable to those of LPS (1 µg/ml). Collectively, these findings indicated that HAC treatment induced proinflammatory cytokines, such as IL-6 and TNF-α, in RAW264.7 cells.
Phagocytosis is the essential function of macrophages, resulting in removing invading pathogens and initiating the immune response (19). Thus, phagocytic activity is commonly used in evaluating the functionality of macrophages. Here, the effect of HAC on the phagocytic activity of macrophages was evaluated by measuring the uptake of FITC-labeled dextran particles. As shown in Fig. 2C, increasing fluorescent intensity due to uptake of FITC–dextran by macrophages indicated that HAC treatment enhanced phagocytic activity in a dose-dependent manner.
Macrophages that are activated upon pathogen recognition and phagocytosis produce intracellular ROS, which, in turn, kill phagocytosed pathogens. Therefore, the cell-permeable probe CM-H2DCFDA was used to assess whether HAC triggers ROS generation. As shown in Fig. 2D, HAC significantly increased ROS production compared with the non-treated control, and the highest dose of HAC induced a similar level of ROS as LPS (1 µg/ml). These findings indicate that ROS, along with NO, produced by HAC treatment might cause efficient clearance of engulfed bacterial pathogens.
To investigate the molecular mechanism responsible for HAC-induced immunostimulatory effects, RAW264.7 cells were stimulated with HAC (200 µg/ml) at the indicated times, and analyzed by immunoblotting to detect the level of phosphorylation of signaling molecules of the NF-κB and MAPK pathways. As shown in Fig. 3A, LPS treatment promoted phosphorylation of p65 and IκBα at 5–10 min, while phosphorylation of ERK, JNK, and p38 occurred at 10–30 min. HAC treatment also induced phosphorylation of these signaling molecules but the responses were delayed and slightly weaker when compared with LPS treatment (Fig. 3). In summary, these results suggest that HAC may activate the MAPK signaling pathway, as well as the NF-κB signaling pathway, leading to activation of macrophages.
AC is a representative herbal plant prescribed to relieve pain, lumbago, rheumatism, and lameness, in Korea (20). Although various studies have examined the physiological activity of AC, such as hepatotoxicity, anti-inflammatory, and anti-cancer effects, its immunomodulatory action on the RAW264.7 murine cell line has not been investigated until now. Many herbal extracts exert immunomodulatory activity through their ability to modulate macrophage functions. Some studies demonstrated that herbal extracts have a potent immunostimulatory activity, leading to induction of inflammatory mediators, such as NO and several cytokines (21, 22). NO production has been considered as a first-line defense against invasion of microbial organisms, such as parasites, bacteria, and viruses (23, 24). Therefore, the amount of NO formation is regarded as the level of immunostimulatory activity in macrophages. Qiang et al. demonstrated that purified Ganoderma atrum polysaccharide (PGS-1) promotes production of NO, ROS, and cytokines, providing evidence that PGS-1 enhances macrophage and immunomodulatory activities (25). Our results demonstrated that HAC enhances NO production and iNOS expression, but did not show cytotoxicity.
Macrophages exposed to stimulating agents, including LPS, release several cytokines, as well as NO. These cytokines include TNF-α, IL-6, interleukin-10, and interleukin-12 (IL-12), which induce secondary immune responses, such as differentiation and proliferation of other immune cells (26). Based on the research of immunostimulatory extracts from herbal plants, the extracts enhanced NF-κB-mediated production of macrophage-related cytokines and induced activation of the adaptive immune response through production of proinflammatory cytokines (27, 28). In another study, stimulation of monocytes/macrophages led to the expression of many genes encoding immunomodulatory proteins and peptides, such as macrophage-, granulocyte-, and granulocyte/macrophage-colony stimulating factor, the inflammatory cytokines TNF-α, interleukin-1 (IL-1), IL-6, membrane receptors, the chemotactic protein MCP-1, and NOS (29). A recent study suggested that exopolysaccharides promote functional and phenotypic activation of macrophages, as evidenced by the ability of the exopolysaccharides to induce morphological changes, stimulate cell activities, and strongly upregulate the production of cytokines, such as TNF-α, IL-1β, IL-6, and IL-12 (30). In a previous study, we determined that polysaccharide isolated from HAC upregulated the innate immune response and L. monocytogenes clearance in vivo (6). In conclusion, our results indicated that HAC promotes the stimulation of monocytes/macrophages and release of proinflammatory cytokines, thereby inducing the immune response.
The transcription factor NF-κB is the key regulator of the expression of macrophage-related cytokines (29). Upon cell activation, the phosphorylation of IκB leads to degradation and activation of NF-κB. Xie et al. published that in activated macrophages, the NF-κB pathway is critical in the induction of iNOS (31). According to other studies, herbal or medicinal plant extracts with immunostimulatory activities often triggered phosphorylation of IκBα and NF-κB p65 (32,33,34). Similarly, we found that HAC contributed both to activation of the NF-κB pathway and production of iNOS. In addition, MAPK is major signaling pathway for macrophage activation and macrophage-related cytokine production by various stimulation factors (35, 36). The upregulation of MAPK can activate numerous transcription factors following production of NO and cytokines (35). Not surprisingly, HAC also increased MAPKs, implying the role of this signaling in macrophage activation by HAC treatment. Consequently, we suggest that both the NF-κB and MAPK pathways, respectively, might be involved in the immunostimulatory effect of HAC on macrophages.
This study described that HAC increases the production of crucial inflammatory mediators, such as NO, ROS, and cytokines, and promotes phagocytic activity. Furthermore, we obtained results that HAC activates NF-κB, which is one of the most important transcription factors in macrophages, and also activates the MAPK signaling pathway in macrophages. From these results, we suggest that HAC could be used to enhance the innate immune system mediated by macrophages.
Figures and Tables
Figure 1
Effect of HAC on cell viability, nitrite accumulation, and iNOS expression in RAW264.7 cells. (A) Cells were treated with different concentrations of HAC for 24 h, and their viability was assessed by the MTT assay. The results of independent experiments were averaged and are expressed as the percentage of cell viability relative to the untreated control group. (B) Nitric oxide production was measured in the culture medium by the Griess reaction, and NaNO2 was used as a standard. (C) iNOS expression was determined in RAW264.7 cells treated with LPS or HAC by immunoblotting. Data are presented as the mean ± SEM of three independent experiments. **p<0.01, ***p<0.001 indicate significant difference from the untreated control group by the Student's t-test.
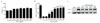
Figure 2
Effects of HAC on cell morphology, cytokine and ROS production, and phagocytosis. RAW264.7 cells were plated and then treated with the indicated concentration of HAC for 24 h. (A) Cell morphology was observed by light microscopy. Images were obtained with a 100×objective. (B) IL-6 and TNF-α levels were determined using an ELISA kit, as described in the text. (C) After incubation, the cells were washed twice with PBS and labeled with 20 µM CM-H2DCFDA for 30 min. The intracellular ROS level was analyzed by flow cytometry. (D) The cells were incubated with DMEM containing 1 mg/ml FITC-dextran, 2% FBS, and the indicated concentration of HAC for 6 h. After incubation, phagocytosis activity was measured by flow cytometry. The results are expressed as mean ± SEM of three independent experiments. *p<0.05, *p<0.01, ***p<0.001 indicate significant difference from the untreated control group by the Student's t-test.
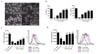
Figure 3
Effects of HAC on NF-κB and MAPK pathways. RAW264.7 cells were incubated at a density of 1×106 cells/well in a 12-well plate and stimulated with 200 µg/ml of HAC or 1 µg/ml of LPS for different times. Total cell lysates were collected and hybridized with a specific antibody detecting the phosphorylated form or total amount of NF-κB and MAPK. The results are representative of three independent experiments.
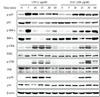
Acknowledgments
This work was carried out with the support of the Cooperative Research Program for Agriculture Science and Technology Development (Project No. PJ009787 and PJ01270402), Rural Development Administration, Republic of Korea.
References
1. Park CW. The genera of vascular plants of Korea. Seoul: Academic Publishing;2007. p. 1482.
2. Perry LM, Metzger J. Medicinal plants of east and southeast Asia: attributed properties and uses MIT press. 1980.
3. Hwang YP, Choi JH, Jeong HG. Protective effect of the Aralia continentalis root extract against carbon tetrachlorideinduced hepatotoxicity in mice. Food Chem Toxicol. 2009; 47:75–81.


4. Park HJ, Hong MS, Lee JS, Leem KH, Kim CJ, Kim JW, et al. Effects of Aralia continentalis on hyperalgesia with peripheral inflammation. Phytother Res. 2005; 19:511–513.


5. Han GJ, Shin DS, Jang MS. A study of the nutritional composition of Aralia continentalis Kitagawa and Aralia continentalis Kitagawa leaf. Korean J Food Sci Technol. 2008; 40:680–685.
6. Seo DW, Cho YI, Gu S, Kim DH, Park JH, Yi YJ, et al. A hot water extract of Aralia cordata activates bone marrow-derived macrophages via a myeloid differentiation protein 88-dependent pathway and protects mice from bacterial infection. Microbiol Immunol. 2016; 60:343–355.


8. Fujiwara N, Kobayashi K. Macrophages in inflammation. Curr Drug Targets Inflamm Allergy. 2005; 4:281–286.


10. Clancy RM, Amin AR, Abramson SB. The role of nitric oxide in inflammation and immunity. Arthritis Rheum. 1998; 41:1141–1151.


11. Chan J, Tanaka K, Carroll D, Flynn J, Bloom BR. Effects of nitric oxide synthase inhibitors on murine infection with Mycobacterium tuberculosis. Infect Immun. 1995; 63:736–740.


12. Ambs S, Hussain SP, Harris CC. Interactive effects of nitric oxide and the p53 tumor suppressor gene in carcinogenesis and tumor progression. FASEB J. 1997; 11:443–448.


13. Mantovani A, Sica A, Sozzani S, Allavena P, Vecchi A, Locati M. The chemokine system in diverse forms of macrophage activation and polarization. Trends Immunol. 2004; 25:677–686.


14. Lee J, Choi JW, Sohng JK, Pandey RP, Park YI. The immunostimulating activity of quercetin 3-O-xyloside in murine macrophages via activation of the ASK1/MAPK/NF-kappaB signaling pathway. Int Immunopharmacol. 2016; 31:88–97.


15. Li J, Qian W, Xu Y, Chen G, Wang G, Nie S, et al. Activation of RAW 264.7 cells by a polysaccharide isolated from Antarctic bacterium Pseudoaltermonas sp. S-5. Carbohydr Polym. 2015; 130:97–103.


16. Catchpole B, Hamblin AS, Staines NA. Autologous mixed lymphocyte responses in experimentally-induced arthritis of the Lewis rat. Autoimmunity. 2002; 35:111–117.


17. Brito LA, Singh M. Acceptable levels of endotoxin in vaccine formulations during preclinical research. J Pharm Sci. 2011; 100:34–37.


18. Foresti R, Clark JE, Green CJ, Motterlini R. Thiol compounds interact with nitric oxide in regulating heme oxygenase-1 induction in endothelial cells. Involvement of superoxide and peroxynitrite anions. J Biol Chem. 1997; 272:18411–18417.


19. Aderem A, Underhill DM. Mechanisms of phagocytosis in macrophages. Annu Rev Immunol. 1999; 17:593–623.


20. Kim JS, Kang SS. Saponins from the aerial parts of Aralia continentalis. Nat Prod Sci. 1998; 4:45–50.
21. Adachi Y, Okazaki M, Ohno N, Yadomae T. Enhancement of cytokine production by macrophages stimulated with (1-->3)-beta-D-glucan, grifolan (GRN), isolated from Grifola frondosa. Biol Pharm Bull. 1994; 17:1554–1560.


22. Wang ZM, Peng X, Lee KLD, Tang JC, Cheung PCC, Wu JY. Structural characterisation and immunomodulatory property of an acidic polysaccharide from mycelial culture of Cordyceps sinensis fungus Cs-HK1. Food Chem. 2011; 125:637–643.


24. Gorbunov N, Esposito E. Nitric oxide as a mediator of inflammation. Int J Immunopathol Pharmaool. 1993; 6:67–75.
25. Yu Q, Nie SP, Li WJ, Zheng WY, Yin PF, Gong DM, et al. Macrophage immunomodulatory activity of a purified polysaccharide isolated from Ganoderma atrum. Phytother Res. 2013; 27:186–191.


27. Han EH, Choi JH, Hwang YP, Park HJ, Choi CY, Chung YC, et al. Immunostimulatory activity of aqueous extract isolated from Prunella vulgaris. Food Chem Toxicol. 2009; 47:62–69.


28. Yue GG, Chan BC, Hon PM, Kennelly EJ, Yeung SK, Cassileth BR, et al. Immunostimulatory activities of polysaccharide extract isolated from Curcuma longa. Int J Biol Macromol. 2010; 47:342–347.


29. Baeuerle PA, Henkel T. Function and activation of NF-kappaB in the immune system. Annu Rev Immunol. 1994; 12:141–179.


30. Lee JY, Kim JY, Lee YG, Rhee MH, Hong EK, Cho JY. Molecular mechanism of macrophage activation by Exopolysaccharides from liquid culture of Lentinus edodes. J Microbiol Biotechnol. 2008; 18:355–364.
31. Xie QW, Kashiwabara Y, Nathan C. Role of transcription factor NF-kappa B/Rel in induction of nitric oxide synthase. J Biol Chem. 1994; 269:4705–4708.


32. Sun H, Zhang J, Chen F, Chen X, Zhou Z, Wang H. Activation of RAW264. 7 macrophages by the polysaccharide from the roots of Actinidia eriantha and its molecular mechanisms. Carbohydr Polym. 2015; 121:388–402.


33. Kim YS, Kim EK, Nawarathna WPAS, Dong X, Shin WB, Park JS, et al. Immune-Stimulatory Effects of Althaea rosea Flower Extracts through the MAPK Signaling Pathway in RAW264.7 Cells. Molecules. 2017; 22.


34. Bai Y, Jiang Y, Liu T, Li F, Zhang J, Luo Y, et al. Xinjiang herbal tea exerts immunomodulatory activity via TLR2/4-mediated MAPK signaling pathways in RAW264.7 cells and prevents cyclophosphamide-induced immunosuppression in mice. J Ethnopharmacol. 2019; 228:179–187.


35. Johnson GL, Lapadat R. Mitogen-activated protein kinase pathways mediated by ERK, JNK, and p38 protein kinases. Science. 2002; 298:1911–1912.


36. Rao KM. MAP kinase activation in macrophages. J Leukoc Biol. 2001; 69:3–10.