Abbreviations
AIM2
ASC
BMDM
DAMP
MOI
NLR
NLRP3
NS
PI
ZIKV
INTRODUCTION
MATERIALS AND METHODS
Mice
Cell cultures
Reagents and Abs
Expression constructs
Virus infection
Assay of inflammasome activation
Immunoblot analysis
Quantification of mRNA production
RESULTS
ZIKV infection induces NF-κB-dependent production of IL-1β mRNA but not the secretion of IL-1β in BMDMs and mixed glial cells
Figure 1
ZIKV infection induces NF-κB-dependent mRNA production of IL-1β but not the secretion of IL-1β from bone marrow-derived macrophages and brain mixed glial cells. (A) Quantification of IFN-β in culture supernatants of BMDMs infected with mock or ZIKV (MOI=10) at 4-h post-infection (PI) (n=2). (B, C) Quantification of IL-1β (B) or IL-6 (C) in culture supernatants of BMDMs infected with mock or ZIKV (MOI=10) at 24-h PI (n=3). (D, E) Quantification of ZIKV (D) or IL-1β (E) mRNA levels in the mock- or ZIKV-infected (MOI=10) at 24-h PI (n=3). (F-H) Quantification of IL-1β (F) or IL-6 (G) in culture supernatants or IL-1β mRNA levels (H) of mixed glial cells infected with ZIKV as in (B-E) (n=3). (I, J) Immunoblots of cellular lysates from BMDMs (I) or mixed glial cells (J) infected with mock or ZIKV (MOI=10) at 20-h PI.
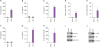
ZIKV infection does not promote caspase-1 activation in macrophages and glial cells
Figure 2
ZIKV infection fails to induce inflammasome activation in bone marrow-derived macrophages and brain mixed glial cells. (A) Quantification of IL-1β in culture supernatants of BMDMs primed with LPS (0.25 µg/ml, 3 h) and further infected with mock or ZIKV (MOI=10) for 24 h (n=3). (B, C) Quantification of IL-1β in culture supernatants (B) or immunoblots in culture supernatants (Sup) or cellular lysates (Lys) (C) of BMDMs infected with mock or ZIKV (MOI=10) for 20 h or treated with LPS (0.25 µg/ml, 3 h), followed by ATP treatments (2.5 mM, 30 min). (D) Quantification of IL-1β in culture supernatants of LPS-primed mixed glial cells infected with mock or ZIKV (MOI=10) for 24 h (n=3). (E, F) Quantification of IL-1β in culture supernatants (E) or immunoblots in culture supernatants (Sup) or cellular lysates (Lys) (F) of mixed glial cells treated as in (B, C) (n=3).
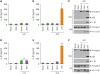
ZIKV infection inhibits NLRP3-dependent caspase-1 activation and ASC oligomerization
Figure 3
ZIKV infection attenuates NLRP3-dependent inflammasome activation in bone marrow-derived macrophages and brain mixed glial cells. (A-D) Quantification of IL-1β or IL-6 in culture supernatants of BMDMs (A-C) or mixed glial cells (D) infected with mock or ZIKV (MOI=10, 20 h), washed and treated with LPS (0.25 µg/ml, 3 h), followed by the treatment with ATP (1.5 mM, A, B, D) or nigericin (Niger, 1 µM, C) for 30 min. (n=4, A-C; n=3, D). (E-H) Representative immunoblots from BMDMs (E, F, left panel of H) or mixed glial cells (G, right panel of H) treated as in (A-D). Culture supernatants (Sup), disuccinimidyl suberate (DSS)-crosslinked pellets (Pel+DSS) or cellular lysates (Lys) were immunoblotted with the indicated Abs.
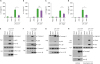
ZIKV infection impairs NLRP3 inflammasome activation in an NS3-dependent manner
Figure 4
ZIKV NS3 inhibits NLRP3-dependent caspase-1 activation and IL-1β secretion from bone marrow-derived macrophages and brain mixed glial cells. (A, B) Immunoblots from BMDMs (A) or mixed glial cells (B) infected with mock or ZIKV (MOI=10, 20 h), washed, and then transfected with poly dA:dT (1 μg/ml, 6 h). (C-E) Quantification of IL-1β in culture supernatants of BMDMs transfected with GFP control, ZIKV NS1 (C), ZIKV NS3 (D) or ZIKV NS5 (E) expression plasmid (0.5 µg) for 40 h, then treated with LPS (0.25 µg/ml, 3 h) and ATP (2 mM, 1 h) (n=5, C; n=6, D-E). (F-H) Immunoblots in culture supernatants (Sup) or RT-PCR for the mRNA expression of target genes in the cellular extracts from BMDMs treated as in (C-E). (I) Immunoblots from BMDMs transfected with GFP or ZIKV NS3 expression plasmid for 6 h, washed, incubated for additional 40 h, and then transfected with poly dA:dT (1 μg/ml, 6 h). (J) Immunoblots of the cell lysates of 293T cells transfected with NLRP3 or NS3 plasmids for 6 h, washed, and further incubated for 40 h with or without serine protease inhibitor (0.5 µM, PMSF).
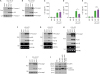