Abstract
Purpose
Materials and Methods
Results
Figures and Tables
Fig. 1
Curcumin inhibited proliferation and promoted apoptosis of SU-DHL-8 cells. SU-DHL-8 cells were treated with different concentrations (0, 5, 10, 20, 40, and 60 µmol/L) of curcumin. (A) At 0, 24, 48, 72, and 96 h treatment, cell proliferation was detected by MTT assay. (B) After 24 h treatment, cell apoptosis rate was measured by flow cytometric analysis. (C) Western blot was performed to determine the levels of Ki-67, caspase-3, and cleaved caspase-3 in curcumin-treated cells. *p<0.05 vs. control.
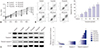
Fig. 2
Curcumin repressed the migration and invasion of SU-DHL-8 cells. SU-DHL-8 cells were treated with different concentrations (0, 5, 10, 20, 40, and 60 µmol/L) of curcumin for 24 h, followed by the measurement of cell migration (A) and invasion (B) abilities by transwell assay (×100 magnification). *p<0.05 vs. control.
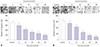
Fig. 3
Curcumin inhibited miR-21 expression of SU-DHL-8 cells. (A) MiR-21 expression was detected in 45 cases of DLBCL tissues and 23 cases of reactive lymphoid hyperplasia tissues. (B) Representative ISH of miR-21 in DLBCL tissues and reactive lymphoid hyperplasia tissues (×100 magnification). (C) MiR-21 expression was assessed in DLBCL cell lines (SU-DHL-8, OCI-LY1 and SU-DHL-10) and normal control CD19+ cells by qRT-PCR. (D) SU-DHL-8 cells were treated with different concentrations (0, 5, 10, 20, 40, and 60 µmol/L) of curcumin for 24 h, followed by the determination of miR-21 expression. *p<0.05 vs. corresponding control. DLBCL, diffuse large B-cell lymphoma; ISH, in situ hybridization.
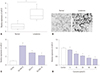
Fig. 4
The effects of curcumin on proliferation and apoptosis were mediated by miR-21 in SU-DHL-8 cells. SU-DHL-8 cells were transfected with NC mimics or miR-21 mimics, and then were treated with or without curcumin (20 µmol/L). (A) At 0, 24, 48, 72, and 96 h treatment, MTT assay was performed to detect cell proliferation ability. (B) At 24 h treatment, flow cytometric analysis was used to assess cell apoptosis. (C) Western blot was performed to determine the levels of Ki-67, caspase-3, and cleaved caspase-3 in treated cells. *p<0.05 vs. NC mimics or NC mimics+curcumin.
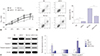
Fig. 5
Curcumin exerted its anti-migration and anti-invasion effects by miR-21 in SU-DHL-8 cells. SU-DHL-8 cells were transfected with NC mimics or miR-21 mimics, and then were treated with or without curcumin (20 µmol/L) for 24 h, followed by the determination of cell migration ability (A) and invasion capacity (B) by transwell assay. *p<0.05 vs. NC mimics or NC mimics+curcumin.
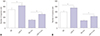
Fig. 6
VHL was a direct target of miR-21. (A) Schematic illustration of the putative binding sites between miR-21 and VHL, and mutation in binding sequence in 3′-UTR of VHL. (B) VHL wild-type or mutant-type reporter plasmids (VHL-WT or VHL-MUT) were constructed and transfected into SU-DHL-8 cells together with NC mimics, miR-21 mimics, anti-NC, or anti-miR-21. Relative luciferase activities were detected. (C) SU-DHL-8 cells were transfected with miR-21 mimics, followed by the detection of VHL mRNA enrichment with anti-Ago2 or anti-IgG. SU-DHL-8 cells were transfected with NC mimics, miR-21 mimics, anti-NC, or anti-miR-21, followed by the measurement of VHL mRNA by qRT-PCR assay (D) and VHL protein by western blot (E). The expression of VHL protein was detected by western blot in DLBCL tissues and reactive lymphoid hyperplasia tissues (F), and in DLBCL cell lines (SU-DHL-8, OCI-LY1, and SU-DHL-10) and normal control CD19+ cells (G). *p<0.05 vs. NC mimics or anti-NC. VHL, Von Hippel-Lindau; DLBCL, diffuse large Bcell lymphoma.
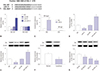
Fig. 7
Curcumin exerted its regulatory effects by VHL in SU-DHL-8 cells. (A) SU-DHL-8 cells were treated with different concentrations (0, 5, 10, 20, 40, and 60 µmol/L) of curcumin for 24 h, followed by the measurement of VHL mRNA expression. SU-DHL-8 cells were transfected with si-VHL or si-NC and then were treated with 20 µmol/L of curcumin. (B) After 24 h treatment, VHL protein expression was determined by western blot analysis. (C) At 0, 24, 48, 72, and 96 h treatment, cell proliferation ability was assessed by MTT assay. (D) At 24 h treatment, cell apoptosis was detected by flow cytometric analysis. (E) Western blot was performed to determine the levels of Ki-67, caspase-3, and cleaved caspase-3. At 24 h treatment, cell migration ability was determined by transwell assay (F) and cell invasion capacity was evaluated by transwell assay (G). *p<0.05 vs. control or si-NC+curcumin. VHL, Von Hippel-Lindau.
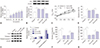
Notes
AUTHOR CONTRIBUTIONS
Conceptualization: Ling Chen, Cheng-Zhi Zhan.
Data curation: Ling Chen, Cheng-Zhi Zhan.
Formal analysis: Ling Chen, Cheng-Zhi Zhan.
Funding acquisition: Ling Chen, Cheng-Zhi Zhan.
Investigation: Rui Yao, Tao Wang.
Methodology: Ling Chen, Cheng-Zhi Zhan.
Project administration: Tao Wang.
Resources: Ling Chen, Tao Wang.
Software: Hua You, Rui Yao.
Supervision: Hua You.
Validation: Ling Chen, Tao Wang.
Visualization: Cheng-Zhi Zhan, Ling Chen.
Writing—original draft: Ling Chen, Cheng-Zhi Zhan.
Writing—review & editing: Ling Chen, Cheng-Zhi Zhan.
References







































































