Abstract
Purpose
To identify the paternal factors responsible for aberrant embryo development leading to loss of foetus in recurrent pregnancy loss (RPL) through proteomic analysis of ejaculated spermatozoa.
Materials and Methods
This prospective study consisted of male partners of RPL patients (n=16) experienced with two or more consecutive unexplained miscarriages and with no female factor abnormality as revealed by gynaecologic investigation including karyotyping and age matched fertile healthy volunteers (n=20). All samples were collected during 2013 to 2015 after getting institutional ethical approval and written consent from the participants. Seminal ejaculates were collected by masturbation after 2 to 3 days of sexual abstinence and analyzed according to World Health Organization 5th criteria 2010. Two-dimensional difference gel electrophoresis followed by mass spectrophotometric analysis was used to identify differentially expressed proteins (DEPs). Western blotting was used for validation of the key proteins.
Results
The data identified 36 protein spots to be differentially expressed by more than 2-fold change with p<0.05 considered as significant. Matrix-assisted laser desorption/ionization time of flight/mass spectrometry identified GPx4, JIP4, ZN248 to be overexpressed while HSPA2, GSTM5, TF3C1, CC74A was underexpressed in RPL group. Western blot analysis confirmed the differential expression of key redox associated proteins GPx4 and HSPA2 in the RPL group. Functional analysis revealed the involvement of key biological processes that includes spermatogenesis, response to oxidative stress, protein folding and metabolic process.
Recurrent pregnancy loss (RPL) is a devastating problem defined by two or more failed clinical pregnancies within the first trimester of gestation [1]. Although, the aetiopathology for RPL varies considerably [23] it is unfortunate to understand that only about 30% of these cases have identifiable etiologies [4] while in the remaining cases the cause remains unexplained. Intriguingly, owing to the close association between the mother and the developing embryo, the majority of the testing for RPL targets the woman. However, nearly 50% of the genomic material is contributed by the paternal factor to the embryo as well as to placental and embryonic development. Modifications at the genetic and epigenetic level within the male gamete may therefore be detrimental to further embryonic growth [56789]. This is significant in the context of RPL since here clinical pregnancy is though established but the problem is confounded by early abortion. To better understand the underlying molecular mechanism the focus of recent research is on sperm chromatin remodelling during spermiogenesis where sequential replacement of histones by protamines is accomplished. However, it has been observed that ~5% to 10% histones are retained at structurally and transcriptionally relevant positions within the sperm chromatin. Thus, the ability of paternal genes to control specific early embryonic gene expression with regards to RPL raises several speculations [10].
This novel study is therefore aimed to identify potential protein biomarkers in the spermatozoa obtained from seminal ejaculates of men who are partners to couples with RPL as compared to their healthy counterparts using a proteomic approach. This is important in context to our previous finding wherein an increased retention of histones with concomitant rise in oxidative stress levels was observed in the spermatozoa of RPL patients [11]. Proteins being the central part of every reproductive process and is subjected to modifications by various exo- and endogenous factors, understanding the molecular mechanism through sperm proteomic approach may shed light in identifying the paternal effects in RPL.
The study was conducted after the approval of Institutional Ethics Committee at Kar Clinic and Hospital Private Limited, Bhubaneswar, Odisha, India (Reg. No: KCHPL/P-1/2013) along with written informed consent was obtained from all sperm donors.
The RPL group includes couples who achieved pregnancy by natural conception but showed a history of ≥2 embryos losses before 20th weeks of gestation detected through ultrasonography. Age-matched controls were included in the study that comprised of healthy fertile donors (with no known medical condition) with no cases of embryo loss and achieved pregnancy within one year of intercourse.
RPL patients (both male and female partners) showing anomalies such as endocrine disorders, anatomical abnormalities, abnormal karyotype etc. were excluded from the study. Male partners who exhibited varicocele, cryptorchidism, or a history of surgery on male genital area were also excluded from the study. Since the diagnosis of male factors in RPL is inconclusive and is impractical to include all possible genetic test to screen the partners, we excluded all male partners having sperm DNA fragmentation <30%. The semen sample from these male partners were subjected to DNA fragmentation analysis by Comet assay [12] and samples with higher DNA fragmentation (>30% in comparison to fertile donors) were selected for the present study.
Semen samples from men from the two groups (control n=20 and RPL n=16) was collected by masturbation after 2 to 3 days of sexual abstinence. All samples used are aliquots of the sperm samples used in our previous study [11]. After complete liquefaction semen samples were subjected for analysis following the World Health Organization 5th ed criteria (2010) [13]. Samples with >1.0×106/mL round cell with a positive peroxidase test were excluded from the study.
Sample preparation and protein extraction was done as previously described with slight modifications [14]. After analysis of several semen parameters, sperm and seminal plasma were separated by centrifuging at 300×g for 10 minutes at 37℃. The obtained sperm pellet was further subjected to three times washing in 10 mM phosphate buffer saline (PBS), pH-7.4 at 200×g for 5 minutes and finally dissolved in 10 mM PBS, pH-7.4 and were kept frozen at −20℃ until further use. For proteomic analysis, samples were thawed, equal number of spermatozoa from each study group were homogenized in radio immuno precipitation assay (RIPA) buffer and protein was estimated according to Lowry et al [15]. After separation the spermatozoa were further processed and exposed to approximately 400 mL ice-cold lysis buffer followed by centrifugation at 10,000 rpm for 10 minutes at 4℃ and the precipitated proteins obtained were then quantified with the Ettan™ 2D Quant Kit (GE Healthcare, Chicago, IL, USA) before labelling with Cy dyes (GE Healthcare).
Aliquots of 75 µg of each of control and RPL extracts were precipitated individually using the Ettan™ 2-dimensional (2D) clean-up kit (Amersham Biosciences, Piscataway, NJ, USA) and subjected to lysis buffer prior to labelling as previously described [14]. Briefly, 50 µg of each sample (control and RPL) were minimally labelled by 400 pmol of amine-reactive cyanine dyes, Cy3 or Cy5. At the same time 50 µg of Internal Standard (Containing 25 µg each of Control and RPL) was generated and labelled with Cy2 dye for a ratio of 50 µg/400 pmol Cy2. Labelling was performed on ice in the dark for 30 minutes followed by subsequent quenching of the reaction mixture.
Protein differences between the two experimental conditions (control and RPL) was detected using 2D sodium dodecyl sulfate polyacrylamide gel electrophoresis (SDS-PAGE) following the protocol as described previously [16]. In brief, the samples were loaded on to the IEF strips 3 to 10 pH Linear, 11 cm (Bio-Rad, Hercules, CA, USA) and kept for Iso-Electric Focusing with the use of the Protean IEF Cell (Bio-Rad). Each electrofocused strips were then equilibrated for 15 minutes first with SDS-PAGE equilibration buffer containing 6 mol/L urea, 30% glycerol, 2% SDS, and 65 mmol/L dithiothreitol (DTT). This was followed by a second equilibration buffer for 15 minutes with 25 mg/mL iodoacetamide instead of DTT. Further the 11-cm equilibrated strips were fit into a Criterion 11-cm IPG gel and a standard second dimension SDS-PAGE was run. The 2D SDS-PAGE was performed on 12% polyacrylamide gels at 20 mA and 16 V on Bio-Rad Protein II (Bio-Rad) and was terminated when the bromophenol blue front had migrated to the lower end of the gels.
After performing SDS-PAGE, the Cy Dye-labelled proteins were visualised by scanning with specified excitation and emission filter wavelengths using Typhoon Trio Imager (GE Healthcare). The images for Cy2 were scanned with488/520 nm; while the images for Cy3 were scanned with 532/580 nm; and the images for Cy5 were scanned with 633/670 nm. All gels were scanned at a resolution of 100 µm. After the acquisition of gel images the images were processed using DeCyder™ 2D ver. 7.0 (Amersham Biosciences). Spots were automatically detected, matched, and normalized to the internal standard, then manually checked to guarantee correct matching across images. For qualitative analysis of differently expressed proteins gels were silver stained and spots of interest excised for further mass spectrometry analysis. Only proteins showing fold change in expression ≥2.0 with statistical significance (Student's t-test 95%, p≤0.05) between (control and RPL) groups were considered to have significant change in expression.
The protein identification by mass spectrometry was done as described previously [17]. In brief, spots of interest were excised from the preparatory gels and were washed in double-distilled water prior to dehydration with 75% acetonitrile and dried in a speed vac. Sample reduction was done with 10 mM DTT in 25 mM ammonium bicarbonate for 30 minutes at 56℃ and subsequent alkylation with 55 mM iodoacetamide in 25 mM ammonium bicarbonate for 20 minutes in the dark. Finally, gel pieces were digested overnight at 37℃ in a buffer containing 25 mM ammonium bicarbonate, 5 mM calcium chloride, and ~20 ng/µL of trypsin. The peptides generated were extracted and subjected to matrix-assisted laser desorption/ionization time of flight/mass spectrometry (MALDI-TOF/MS) analysis was performed using an Ultraflex III TOF-TOF instrument (Bruker Daltonics, Bremen, Germany). The instrument operated in reflectron positive ion mode and the peptides were spotted onto the target plate with α-cyano-4-hydroxycinnamic acid matrix (5 mg in water/acetonitrile/0.1% trifluoro acetic acid) and allowed to dry before introducing into the mass spectrometer. MALDI-TOF MS/MS analysis was performed using the LIFT device. The data obtained were reprocessed using the Flex Analysis 2.4 software (Bruker Daltonics) and optimised for protein identification using Mascot (Matrix Science, Boston, MA, USA) against the Homo sapiens proteome set from the Swissprot database. Protein identifications were accepted if they had greater than 95% probability as represented by the mascot scores in the mascot result page. Further details on the MS protocol can be found in the Supplementary Materials and Methods.
The differentially expressed proteins of interest were verified in each group (control and RPL) using Western blotting (WB) as described previously [18]. Pooled spermatozoa as well as one individual sample from each group (control and RPL) were run to maintain biological variability. Samples were normalized for protein concentration in each group. Washed spermatozoa were subjected to homogenization in RIPA lysis buffer (Sigma-Aldrich, St. Louis, MO, USA) and kept overnight at 4℃ containing proteinase inhibitor cocktail (Roche, Indianapolis, IN, USA). Samples (n=5 per group) containing approximately 20 to 30 µg of protein in 15 µL volume per sample were separated by 12% SDS-PAGE and electroblotted onto polyvinylidenedifluoride membranes. The membranes were incubated with a rabbit polyclonal antibody to HSPA2 and GPx4 (rabbit immunoglobulin G [IgG]; Abcam, Cambridge, MA, USA) diluted at required concentration in 3% bovine serum albumin in Tris-buffered saline Triton X-100 (TBST). As an internal control, blots were deprobed, washed and reprobed with an anti glyceraldehyde 3-phosphate dehydrogenase (GAPDH) antibody (rabbit IgG; Abcam). Blots were then washed using TBST and incubated with horseradish peroxidase conjugated anti-rabbit IgG (Abcam) for 1 hour at room temperature following washes in TBST. Visualisation of protein bands was done using an enhanced chemiluminescence kit-Pierce™ ECL Western Blotting Substrate (Thermo Scientific, Rockford, IL, USA). Image J software was used for further quantification and the ratio of intensity of the band to that of the internal control GAPDH was taken for comparison among the two groups.
Shapiro–Wilk test followed by Levene's test were used to assess the data for normalisation and homogeneity of variance. Analyses were carried out using Statistical Package for the Social Sciences (SPSS) ver. 20 (IBM SPSS Statistics; IBM Corp., Armonk, NY, USA). Results of both semen parameters and WB were subjected to two-tailed unpaired Student's t-test with a significance set at p<0.05.
Since all samples used are aliquots of the sperm samples used in our previous study [11] the semen parameters already provided are reported here for reference (Supplementary Table). Variables are expressed in mean±standard error of mean.
The fluorescent and silver stained overlay images of the two gels with Cy3- and Cy5-labelled samples are shown (Fig. 1A–1C and 1D and 1E). The data revealed that 36 protein spots were differentially expressed by more than 2-fold change in the two groups. Of these 27 were overexpressed and 9 were underexpressed in the RPL group as compared to their normal counterparts (Fig. 2). The 36 protein spots per gel were subjected to further excision for in-gel digestion by trypsin, that resulted in 7 proteins to be successfully identified by MALDI-TOF/MS. Phospholipid hydroperoxide glutathione peroxidase, mitochondrial (GPx4) (spot ID-20), C-Jun-amino-terminal kinase-interacting protein 4 (JIP4) (spot ID-46), zinc finger protein 248 (ZN248) (spot ID-61) were amongst the identified proteins that were found to be overexpressed in the RPL group. While a mixture of Heat shock-related 70 kDa protein 2 and Glutathione S-transferase Mu 5 (HSPA2+GSTM5) (spot ID-147) were identified in the same spot, concomitant with the identification of General transcription factor 3C polypeptide 1 (TF3C1) (spot ID-157) and Coiled-coil domain-containing protein 74A (CC74A) (spot ID-1) that were found to be underexpressed in RPL group. The major proteins identified in each spot with its Uniprot accession number, mascot score, number of matches, coverage, molecular weight and other relevant details are documented in Table 1 along with the functional classification of the identified differentially expressed proteins.
The expression profile of two key proteins (Gpx4 and HSPA2) associated with redox regulation and protein folding that plays a pivotal role in sperm function was compared. The arbitrary intensity unit obtained from densitometric analysis of WB revealed an underexpression of the protein HSPA2 while an overexpression of Gpx4 in the spermatozoa of RPL group as compared to the control group (Fig. 2C).
Recent reports have suggested a close association between altered semen parameters and elevated level of embryo fragmentation indicating the possible involvement of paternal factors in early embryo development. Elevation in histone retention levels identified in our previous study [11] concomitant to the abnormal identification of key proteins in the present study associated with sperm chromatin remodelling signifies an aberrant epigenetic modification post-fertilsation. Thus, supporting the fact paternal effect does have a role in unusual early pregnancy loss in RPL patients and needs to be further investigated.
The pronuclear stage of zygotes experiences dynamic histone modifications that is more distinct in paternal than maternal pronuclei [19]. Histones are known to be an integral part of sperm nucleosome that aids in packaging the sperm chromatin during sperm maturation and are susceptible to covalent modifications. Proteomic as well as microscopic analysis have revealed the role of HSPA2 in elongating spermatids, with unique redistribution pattern from a dispersed intranuclear location to subacrosomal domains. That correlates with the cessation of histone acetylation and histone removal as a part of chromatin remodelling process [20]. The present study identifies HSPA2 in an underexpressed state that may attribute to unusal histone modifications within the spermatozoa leading to oxidative stress as reported in our previous study [11]. On the other hand specific sperm domains responsible for oocyte interaction established during spermatogenesis [21]. Oxidative stress results in chemical alkylation of HSPA2 protein that works in concert with SPAM1 and ARSA for the initial tethering of human spermatozoa to the zona pellucida [22]. Consequently, aberrantly expressed HSPA2 in the spermatozoa of RPL patients may lead to compromised zona-pellucida interactions that facilitates fertilisation though but prohibits further development. Studies have also revealed that induction of iHsp70 in myoblasts after heat shock strongly depends on the transient activation of JNKs signalling pathway and c-Jun activation [23]. JIP4 is a group of scaffold proteins selectively mediates JNK signaling by aggregating specific components of the MAPK cascade to form a functional JNK signaling module [24]. With the identification of JIP4 in an overexpressed state in our present study further signifies of an abrupt signal transduction pathway. During early embryonic development, a fine tuning between signal transduction, transcriptional machinery, and epigenetic factors is warranted. A large body of literature suggests the possible role of sperm transcripts encoding proteins in early embryos [25]. ZN318 is a member of zinc finger family protein that regulates androgen receptor-mediated transcriptional activation [26], while ZN185 induces fertility suppression [27]. Zinc finger proteins have a role in sperm development there exists paucity of data on ZN248 (overexpressed) identified in the present study. On the other hand, small RNAs (tRNAs and micro RNAs) are reported to be involved in sperm maturation, with TF3C1 is known to be involved in RNA polymerase III-mediated transcription while there exists negligible knowledge with its relation to sperm function. Further the detection of CC74A in altered state with unknown function raises several questions about the mechanistic role played by these proteins during early development.
Reactive oxygen species (ROS) at optimal level is essential for physiological function of spermatozoa such as disulfide bridging events of sperm chromatin while at elevated levels may lead to cellular oxidative damage. Adequate amount of antioxidants protects the spermatozoa from damage, whereas low amounts may render the spermatozoa vulnerable to ROS attack. This defensive mechanism of the spermatozoa towards oxidative stress works in concert with the glutathione system specifically controlled by the GPx family members that has been correlated with embryo morphology on day 3 [28]. GPx4 is an essential selenoenzyme that exists as a cytosolic form, mitochondrial form, and nuclear form necessary for early embryogenesis and cell viability because of its unqiue ability to prevent phospholipid oxidation. Being predominantly expressed in late spermatids and spermatozoa is also known to play a vital role in the formation of disulfide bridging intermediates [29]. While, GSTM5 is a member of the µ-class of glutathione S-transferases is abundantly expressed in the testis that protects the spermatozoa from oxidative stress through several events of phosphorylation/ dephosphorylation while its disruption leads to an increase in protein sulfhydryl oxidation [30]. The present study identified GPx4 and GSTM5 that aids in chromatin remodelling and protection against oxidative stress within the sprematozoa in an altered state (overexpressed and underexpressed respectively). Thus signifying, excessive ROS generation leads to an imbalance in antioxidant level and contribute to elevated histone retention [11] as well as to aberrant embryo formation with the potent responsible for failed pregnancy despite successful conception. Althogh, low sample size is a limitation of the present study due to the difficulty to enrol sufficient patients who are experiencing RPL and willing to participate in this study, and warrants further investigation. However, this study again gives scope to re-think and speculate that does oxidative stress in spermatozoa of RPL patients have the capacity to alter epigenetic signatures in early embryonic development (Fig. 3) - a theory that has been less investigated.
The present study is thus, a step forward in understanding the paternal role in RPL patients with the quest that pinpoints the consequences of aberrant epigenetic signatures in early embryonic development. In brief, the findings of the present study relates to the identification of specific differentially expressed proteins in the spermatozoa of RPL patients that have a pivotal role in the transmission of correct epigenetic signatures post-fertilisation. A further in depth analysis using LC-MS/MS approach is thus warranted that may lead to identification of more number of proteins involved in paternal contribution to pregnancy maintenance and embryo development.
ACKNOWLEDGEMENTS
This research was supported by University Grants Commission, government of India (No. F.15-1/2017/PDFWM-2017-18-ORI-48394 (SA-II) and No. 19/06/2016(i) EU-V), Council for Scientific and Industrial Research, government of India (No. 09/1036/0004/2016), INSPIRE Program under Department of Science and Technology, government of India (NO. DST/INSPIRE Fellowship/2010 [IF10240] and No. DST/AORC-IF/IF150007). Infrastructure assistance to the Center of Excellence in Environment and Public Health by Higher Education Department, Government of Odisha under OHEPEE is grateful acknowledged (HE-PTC-WB-02017).
Notes
Data Sharing Statement: The data analyzed for this study have been deposited in HARVARD Dataverse and are available at https://doi.org/10.7910/DVN/V6LEG0.
References
1. Practice Committee of American Society for Reproductive Medicine. Definitions of infertility and recurrent pregnancy loss: a committee opinion. Fertil Steril. 2013; 99:63. PMID: 23095139.
2. Bareh GM, Jacoby E, Binkley P, Chang TC, Schenken RS, Robinson RD. Sperm deoxyribonucleic acid fragmentation assessment in normozoospermic male partners of couples with unexplained recurrent pregnancy loss: a prospective study. Fertil Steril. 2016; 105:329–336.e1. PMID: 26607021.


3. Rogenhofer N, Ott J, Pilatz A, Wolf J, Thaler CJ, Windischbauer L, et al. Unexplained recurrent miscarriages are associated with an aberrant sperm protamine mRNA content. Hum Reprod. 2017; 32:1574–1582. PMID: 28854581.


4. Dewan S, Puscheck EE, Coulam CB, Wilcox AJ, Jeyendran RS. Y-chromosome microdeletions and recurrent pregnancy loss. Fertil Steril. 2006; 85:441–445. PMID: 16595224.


5. Gil-Villa AM, Cardona-Maya W, Agarwal A, Sharma R, Cadavid A. Assessment of sperm factors possibly involved in early recurrent pregnancy loss. Fertil Steril. 2010; 94:1465–1472. PMID: 19540481.


6. Coughlan C, Clarke H, Cutting R, Saxton J, Waite S, Ledger W, et al. Sperm DNA fragmentation, recurrent implantation failure and recurrent miscarriage. Asian J Androl. 2015; 17:681–685. PMID: 25814156.


7. Gil-Villa AM, Cardona-Maya W, Agarwal A, Sharma R, Cadavid A. Role of male factor in early recurrent embryo loss: do antioxidants have any effect. Fertil Steril. 2009; 92:565–571. PMID: 18829003.


8. Ramasamy R, Scovell JM, Kovac JR, Cook PJ, Lamb DJ, Lipshultz LI. Fluorescence in situ hybridization detects increased sperm aneuploidy in men with recurrent pregnancy loss. Fertil Steril. 2015; 103:906–909.e1. PMID: 25707335.


9. Zidi-Jrah I, Hajlaoui A, Mougou-Zerelli S, Kammoun M, Meniaoui I, Sallem A, et al. Relationship between sperm aneuploidy, sperm DNA integrity, chromatin packaging, traditional semen parameters, and recurrent pregnancy loss. Fertil Steril. 2016; 105:58–64. PMID: 26493117.


10. Meyer RG, Ketchum CC, Meyer-Ficca ML. Heritable sperm chromatin epigenetics: a break to remember. Biol Reprod. 2017; 97:784–797. PMID: 29099948.


11. Mohanty G, Swain N, Goswami C, Kar S, Samanta L. Histone retention, protein carbonylation, and lipid peroxidation in spermatozoa: possible role in recurrent pregnancy loss. Syst Biol Reprod Med. 2016; 62:201–212. PMID: 26980262.


12. Simon L, Carrell DT. Sperm DNA damage measured by comet assay. Methods Mol Biol. 2013; 927:137–146. PMID: 22992910.


13. World Health Organization. WHO laboratory manual for the examination and processing of human semen. 5th ed. Geneva: World Health Organization;2010.
14. Baker MA, Witherdin R, Hetherington L, Cunningham-Smith K, Aitken RJ. Identification of post-translational modifications that occur during sperm maturation using difference in two-dimensional gel electrophoresis. Proteomics. 2005; 5:1003–1012. PMID: 15712234.


15. Lowry OH, Rosebrough NJ, Farr AL, Randall RJ. Protein measurement with the Folin phenol reagent. J Biol Chem. 1951; 193:265–275. PMID: 14907713.


16. Hamada A, Sharma R, du Plessis SS, Willard B, Yadav SP, Sabanegh E, et al. Two-dimensional differential in-gel electrophoresis-based proteomics of male gametes in relation to oxidative stress. Fertil Steril. 2013; 99:1216–1226. PMID: 23312230.
17. Frapsauce C, Pionneau C, Bouley J, Delarouziere V, Berthaut I, Ravel C, et al. Proteomic identification of target proteins in normal but nonfertilizing sperm. Fertil Steril. 2014; 102:372–380. PMID: 24882558.


18. Samanta L, Agarwal A, Swain N, Sharma R, Gopalan B, Esteves SC, et al. Proteomic signatures of sperm mitochondria in varicocele: clinical use as biomarkers of varicocele associated infertility. J Urol. 2018; 200:414–422. PMID: 29530785.


19. Aoshima K, Inoue E, Sawa H, Okada Y. Paternal H3K4 methylation is required for minor zygotic gene activation and early mouse embryonic development. EMBO Rep. 2015; 16:803–812. PMID: 25925669.


20. Govin J, Caron C, Escoffier E, Ferro M, Kuhn L, Rousseaux S, et al. Post-meiotic shifts in HSPA2/HSP70.2 chaperone activity during mouse spermatogenesis. J Biol Chem. 2006; 281:37888–37892. PMID: 17035236.


21. Redgrove KA, Anderson AL, McLaughlin EA, O'Bryan MK, Aitken RJ, Nixon B. Investigation of the mechanisms by which the molecular chaperone HSPA2 regulates the expression of sperm surface receptors involved in human sperm-oocyte recognition. Mol Hum Reprod. 2013; 19:120–135. PMID: 23247813.


22. Bromfield EG, Aitken RJ, Anderson AL, McLaughlin EA, Nixon B. The impact of oxidative stress on chaperone-mediated human sperm-egg interaction. Hum Reprod. 2015; 30:2597–2613. PMID: 26345691.


23. Bironaite D, Pivoriunas A, Venalis A. Upregulation of iHsp70 by mild heat shock protects rabbit myogenic stem cells: involvement of JNK signalling and c-Jun. Cell Biol Int. 2012; 36:1089–1096. PMID: 22946646.


24. Jagadish N, Rana R, Selvi R, Mishra D, Garg M, Yadav S, et al. Characterization of a novel human sperm-associated antigen 9 (SPAG9) having structural homology with c-Jun N-terminal kinase-interacting protein. Biochem J. 2005; 389:73–82. PMID: 15693750.


25. Barroso G, Valdespin C, Vega E, Kershenovich R, Avila R, Avendaño C, et al. Developmental sperm contributions: fertilization and beyond. Fertil Steril. 2009; 92:835–848. PMID: 19631936.


26. Ishizuka M, Ohtsuka E, Inoue A, Odaka M, Ohshima H, Tamura N, et al. Abnormal spermatogenesis and male infertility in testicular zinc finger protein Zfp318-knockout mice. Dev Growth Differ. 2016; 58:600–608. PMID: 27385512.
27. Fan S, Zhao Y, Pan Z, Gao Z, Liang Z, Pan Z, et al. ZNF185-derived peptide induces fertility suppression in mice. J Pept Sci. 2018; 24:e3121. PMID: 30270484.


28. Meseguer M, de los Santos MJ, Simón C, Pellicer A, Remohí J, Garrido N. Effect of sperm glutathione peroxidases 1 and 4 on embryo asymmetry and blastocyst quality in oocyte donation cycles. Fertil Steril. 2006; 86:1376–1385. PMID: 16979635.


29. Puglisi R, Maccari I, Pipolo S, Mangia F, Boitani C. The nuclear form of glutathione peroxidase 4 colocalizes and directly interacts with protamines in the nuclear matrix during mouse sperm chromatin assembly. Spermatogenesis. 2014; 4:e28460. PMID: 25225625.


30. Ijiri TW, Merdiushev T, Cao W, Gerton GL. Identification and validation of mouse sperm proteins correlated with epididymal maturation. Proteomics. 2011; 11:4047–4062. PMID: 21805633.


Supplementary Materials
Supplementary materials can be found via https://doi.org/10.5534/wjmh.190034.
Supplementary Table
Demographic details and attributes of semen parameters of the 36 individuals that participated in the study
Fig. 1
Two-dimensional (2D)-difference gel electrophoresis of proteins isolated from sperm. (A) Control (labelled with Cy3). (B) Recurrent pregnancy loss group (labelled with Cy5). (C) Internal control (labelled with Cy2). (D) Overlay image. (E) Silver stained image showing the identification of 6 spots circled on the 2D gel and marked with their respective spot numbers as designated by DeCyder™ software.
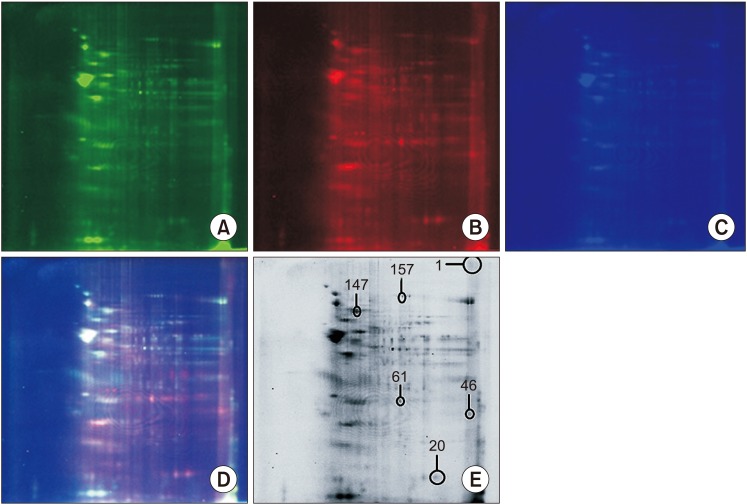
Fig. 2
(A) Venn diagram showing the distribution pattern of the proteins identified through two-dimensional-difference gel electrophoresis. (B) Distribution pattern of differentially expressed proteins based on protein abundance. (C) Expression profile and densitometric analysis of two key proteins (GPx4 and HSPA2) in the spermatozoa of RPL patients compared to fertile donor. RPL: recurrent pregnancy loss, GAPDH: glyceraldehyde 3-phosphate dehydrogenase. *p<0.05 with respect to control.
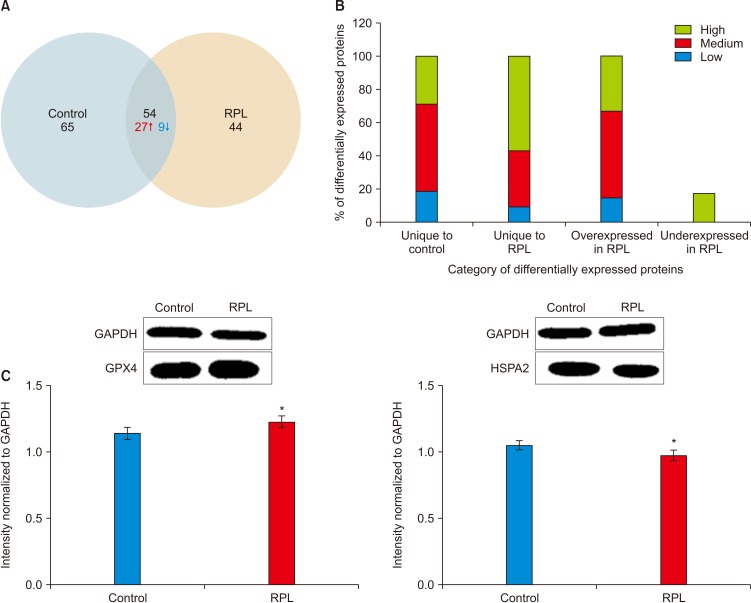
Fig. 3
Schematic representation of proposed hypothesis of involvement of oxidative stress in spermatozoa as epigenetic regulator of paternal factors in recurrent pregnancy loss (RPL). GAPDH: glyceraldehyde 3-phosphate dehydrogenase, 2D-DIGE: two-dimensional difference gel electrophoresis, MS: mass spectrometry.
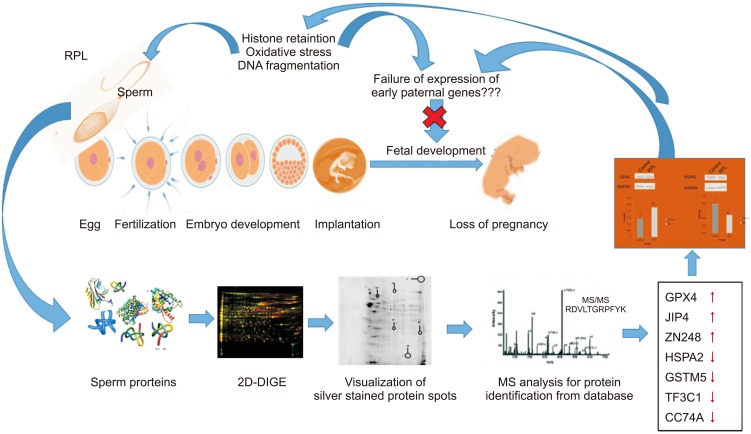
Table 1
Differentially expressed proteins in the spermatozoa of recurrent pregnancy loss as compared to control identified using in-gel digestion of proteins
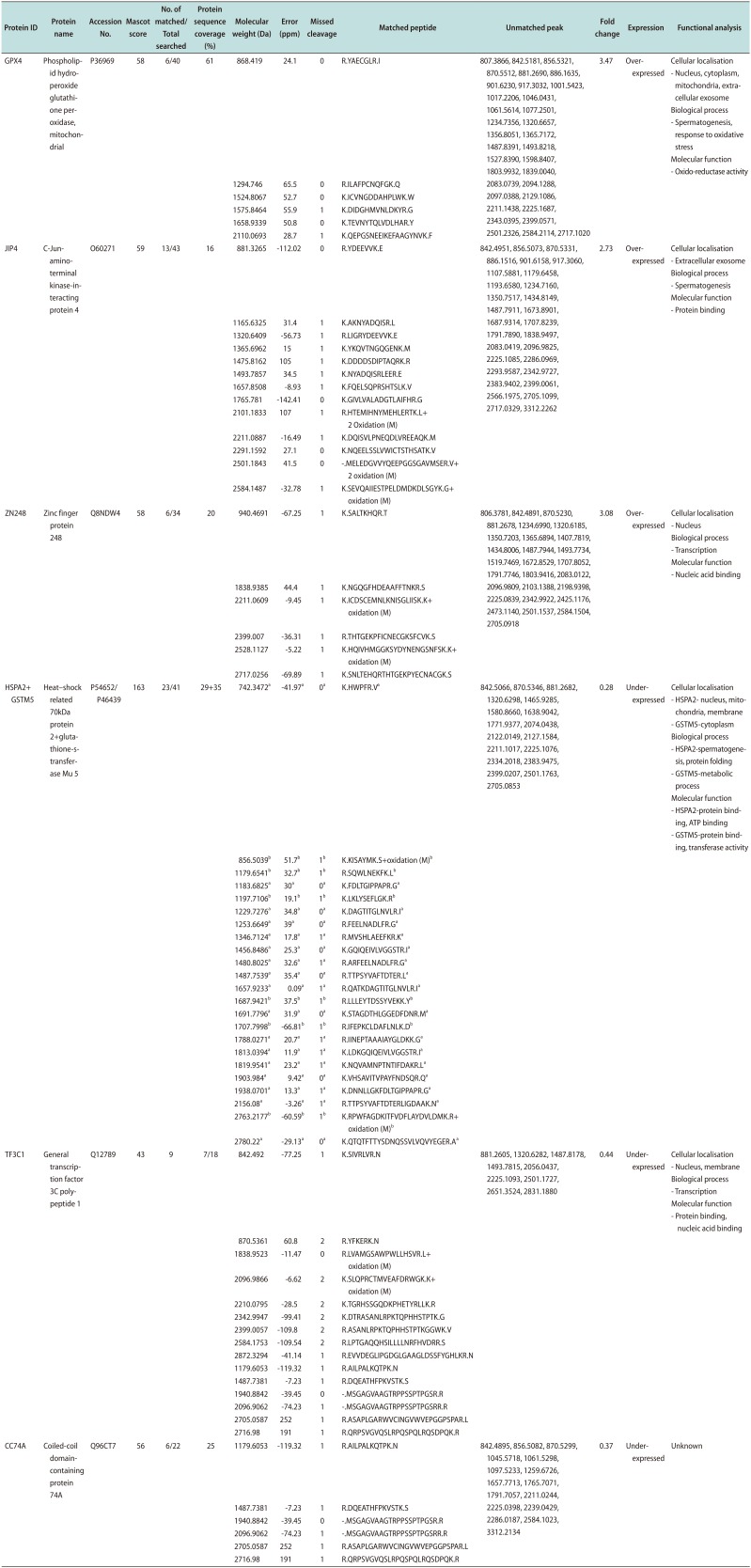