Abstract
Purpose
Materials and Methods
Results
Conclusions
ACKNOWLEDGEMENTS
Notes
Author Contribution:
Research conception & design: Yin GN, Ock J, Ryu JK, Suh JK.
Performing the experiments: Yin GN, Ock J, Choi MJ, Song KM.
Data acquisition: Ghatak K, Minh NN.
Data analysis and interpretation: Yin GN, Ock J, Choi MJ.
Statistical analysis: Kwon MH, Jin HR.
Drafting of the manuscript: Yin GN, Ock J, Ryu JK.
Critical revision of the manuscript: Seong DH, Suh JK.
Receiving grants: Yin GN, Ryu JK.
Approval of final manuscript: all authors.
Data Sharing Statement: The data analyzed for this study have been deposited in HARVARD Dataverse and are available at https://doi.org/10.7910/DVN/Q8KIQD.
References
























Fig. 1
Localization of endothelial cells and pericytes in human corpus cavernosum tissue. Immunofluorescent staining of human penile tissue (n=3) performed with antibodies against CD34 or von Willebrand factor (vWF, endothelial cell markers, red) and platelet-derived growth factor receptor-β (PDGFR-β) or NG2 (pericyte markers, green). Scale bar=25 µm. DAPI: 4,6-diamidino-2-phenylindole (a nuclei marker, blue).
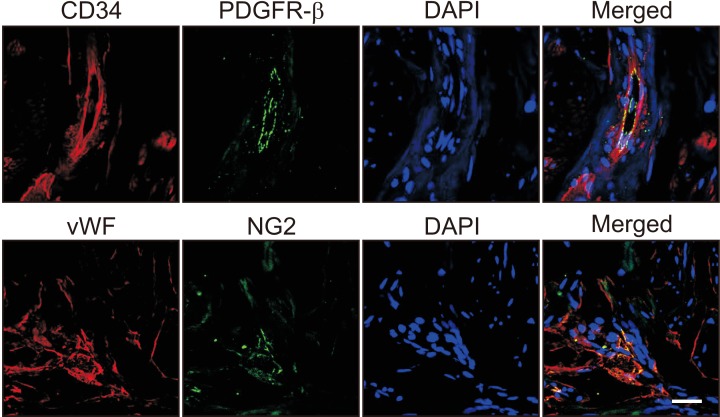
Fig. 2
Isolation and characterization of human cavernous endothelial cells and pericytes. (A) The human corpus cavernosum tissues were implanted on a Matrigel-coated 60-mm cell culture dish with endothelial cell culture medium. A representative image of the cells cultivated for 14 days. After cells were confluent and spread over the whole bottom (21 days), only sprouting cells were used for subcultivation. (B) Fluorescent immunocytochemistry of primary human cavernous endothelial cells (passage 1) with antibodies against von Willebrand factor (vWF, endothelial cell marker) and NG2 (a pericyte marker). Nuclei were labeled with the DNA dye DAPI (4,6-diamidino-2-phenylindole). Scale bar=100 µm. (C) The human corpus cavernosum tissues were implanted on a collagen I-coated 35-mm cell culture dishes with pericyte culture medium. A representative image of the cells cultivated for 14 days. After cells were confluent and spread over the whole bottom (21 days), only sprouting cells were used for subcultivation. (D) Fluorescent immunocytochemistry of primary human cavernous pericytes (passage 1) with antibodies against vWF and NG2. Scale bar=100 µm.
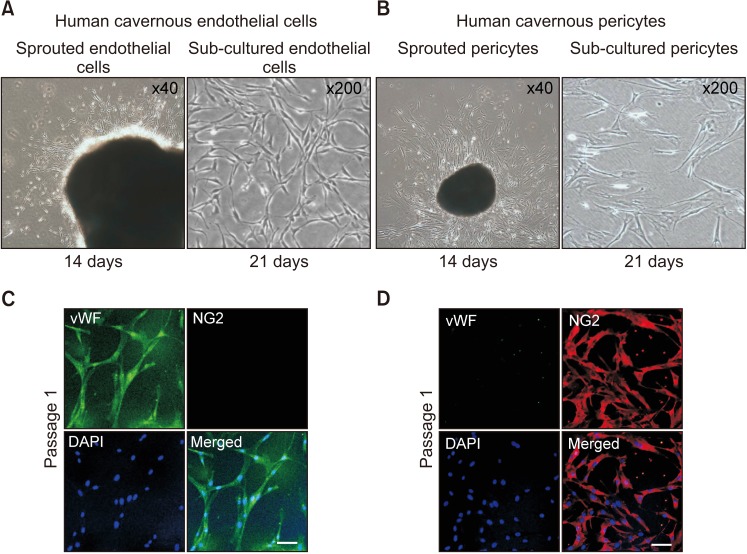
Fig. 3
Morphologic and phenotypic changes of human cavernous endothelial cells (ECs) according to the passages (Ps). (A) Phase image of human cavernous ECs from P1 to P5. (B) Fluorescent immunocytochemistry of human cavernous ECs with antibody against CD34 or von Willebrand factor (vWF, EC markers) and antibody against platelet-derived growth factor receptor-β (PDGFR-β) or NG2 (pericyte markers). Nuclei were labeled with the DNA dye DAPI (4,6-diamidino-2-phenylindole). Scale bar=100 µm. (C) The percentage of CD34- or vWF-positive cells was quantified by Image J. *p<0.05 compared with P1 to P3 groups. (D) Representative Western blot for CD34. (E) Data are presented as the relative density of CD34 to β-actin. The relative ratio measured in the P1 group is arbitrarily presented as 1. *p<0.05 compared with P1 group. **p<0.01 compared with P2 to P3 group. Each bar depicts the mean values (±standard error) from four experiments per group.
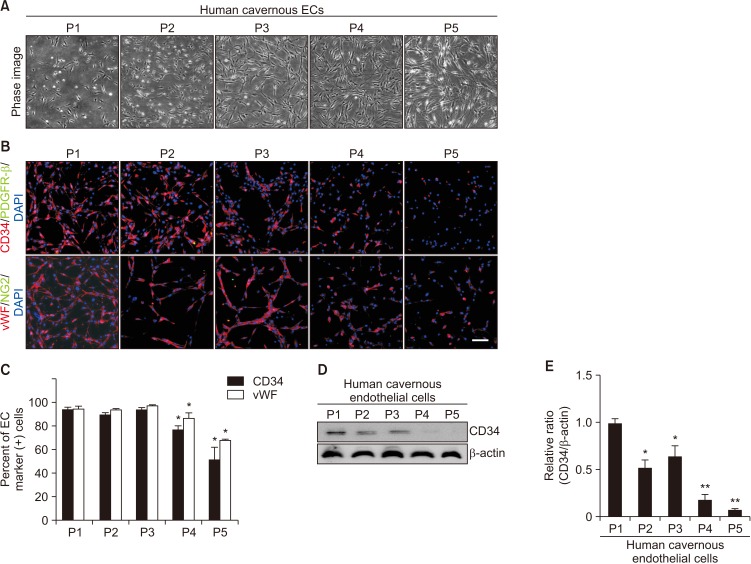
Fig. 4
Morphologic and phenotypic changes of human cavernous pericytes according to the passages (Ps). (A) Phase image of human cavernous pericytes from P1 to P5. (B) Fluorescent immunocytochemistry of human cavernous pericytes with antibody against platelet-derived growth factor receptor-β (PDGFR-β) or NG2 (pericyte markers) and antibody against CD34 or von Willebrand factor (vWF, endothelial cell markers). Nuclei were labeled with the DNA dye DAPI (4,6-diamidino-2-phenylindole). Scale bar=100 µm. (C) The percentage of PDGFR-β or NG2-positive pericytes was quantified by Image J. *p<0.05 compared with P1 to P3 groups. (D) Representative Western blot for PDGFR-β. (E) Data are presented as the relative density of PDGFR-β to β-actin. The relative ratio measured in the P1 group is arbitrarily presented as 1. *p<0.05 compared with P1 to P2 groups. **p<0.01 compared with P3 groups. Each bar depicts the mean values (±standard error) from four experiments per group.
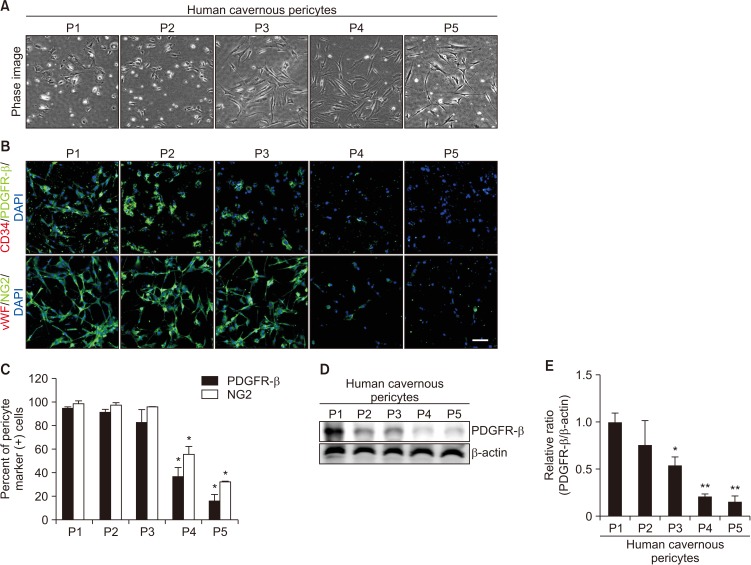
Fig. 5
Differentiation of human cavernous endothelial cells and pericytes into fibroblast-like cells at passage (P) 5. (A, B) Fluorescent immunocytochemistry of human cavernous endothelial cells with antibody against fibroblast-specific protein 1 (FSP1, a fibroblast marker) or von Willebrand factor (vWF, an endothelial cell marker) and antibody against FSP1 or platelet-derived growth factor receptor-β (PDGFR-β). Nuclei were labeled with the DNA dye DAPI (4,6-diamidino-2-phenylindole). Scale bar=100 µm. (C, D) The percentage of FSP1 positive cells in P1 and P5 were quantified by Image J. ***p<0.001 compared with P1 groups. Each bar depicts the mean values (±standard error) from four experiments per group.
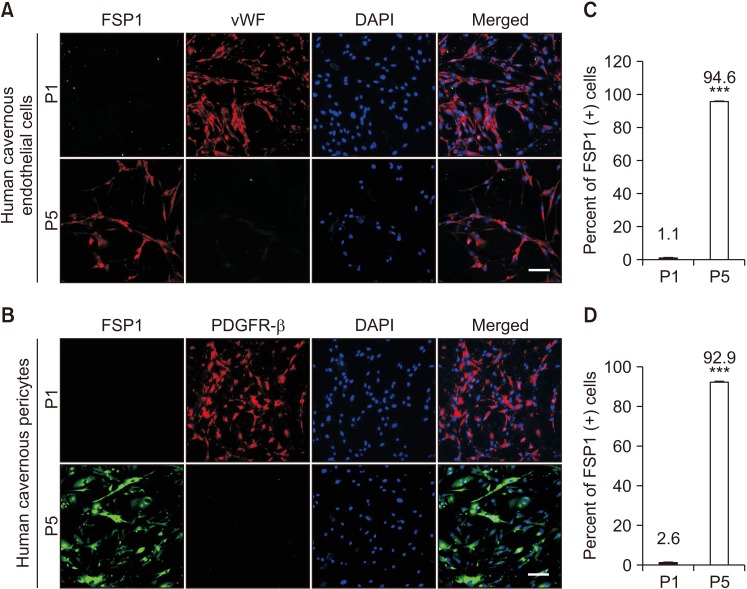
Fig. 6
Decrease in the number of tubes formed in human cavernous endothelial cells or pericytes exposed to the high-glucose (HG) condition. Phase-contrast microscopy of human cavernous endothelial cells (A) and human cavernous pericytes (B). After serum starvation for 24 hours, human cavernous endothelial cells and pericytes were incubated in the normal-glucose (NG, 5 mM) or the HG (30 mM) condition for 48 hours. Then, the tube formation assay on Matrigel was performed in 96-well dishes (×40 magnification). (C, D) Number of branch points per field. Each bar depicts the mean values (±standard error) from four separate wells per group. **p<0.01 compared with the NG group.
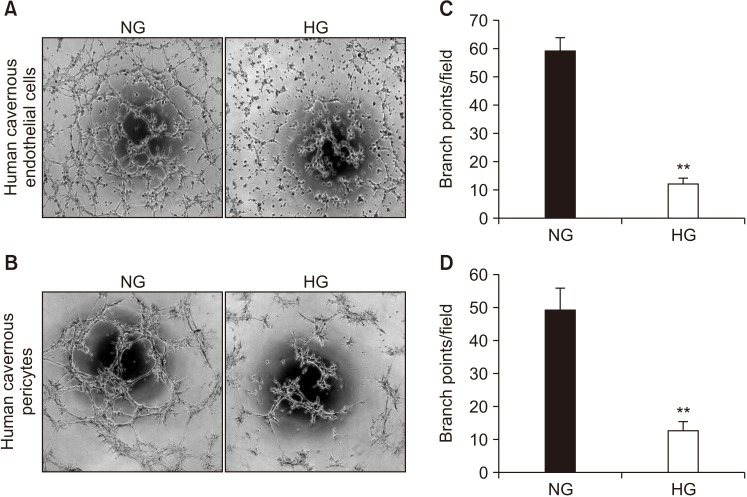