Abstract
Objective
To determine the effects of a local injection of leukocyte-platelet-rich plasma (L-PRP) on orthodontic tooth movement in rabbits.
Methods
Twenty-three male New Zealand white rabbits were included in a split-mouth design. Tooth movement with a 100-g nickel-titanium closed-coil spring was performed on the maxillary first premolars. L-PRP was injected submucosally at the buccal and lingual areas of the first premolar in one random side of the maxilla and the other side served as the control and received normal saline. The amount of tooth movement was assessed on three-dimensional digital models on days 0, 3, 7, 14, 21, and 28. Histological findings and osteoclast numbers were examined on day 0 as the baseline and on days 7, 14, and 28.
Orthodontic tooth movement occurs as a result of remodeling of the alveolar bone and periodontal ligament (PDL) through a biphasic process of bone resorption on the compression side and bone deposition on the tension side. Orthodontic force induces an initial inflammatory response with increased vascular permeability and leukocyte migration. These migrated cells produce inflammatory cytokines that include lymphocyte-derived factors, chemotactic factors, and growth factors. Bone remodeling also occurs with increased bone turnover such as recruitment of osteoclast precursors from the circulation and enhancement of osteoclast formation and activation. Therefore, higher rates of cytokine expression on bone remodeling may accelerate the rate of tooth movement.12
Platelet-rich plasma (PRP) is an autologous concentrate of platelets in a small volume of plasma. It has been used extensively as a source of autologous growth factors and secretory cytokines provided by the concentrated platelet suspension.3 These factors are known to be involved in the regulation and stimulation of cellular processes, such as inflammation, angiogenesis, chemotaxis, proliferation, and differentiation.4 PRP has been suggested to be beneficial in promoting tissue repair and regeneration. Numerous studies56 have demonstrated that the use of PRP in bone regeneration has positive effects on stimulating and enhancing the healing process. However, there is no consensus on the efficacy of PRP due to some controversial findings. The variability in clinical outcomes may have possibly resulted from the variations in the formulations and cellular compositions of the PRP.7
Recently, leukocytes have been included in PRP formulations called leukocyte-platelet-rich plasma (L-PRP), and these formulations have received increased attention for their therapeutic effect on tissue regeneration. Previous studies reported that L-PRP could promote bone regeneration by upregulating cellular proliferation, viability, migration, angiogenesis, and osteogenesis.89 In addition, studies have revealed that the concentration of leukocytes in PRP was correlated with increased levels of catabolic cytokines, including interleukin-1 (IL-1), interleukin-6 (IL-6), and tumor necrosis factor-α (TNF-α), and resulted in the production of destructive metalloproteinases.1011 Interestingly, the presence of concentrated leukocytes in L-PRP could stimulate the nuclear factor κB (NF-κB) pathway via IL-1, TNF-α, and receptor activation of the NF-κB ligand.12 This pathway results in osteoclastogenesis and bone resorptive activity, leading to decreased bone density.
Long-term orthodontic treatment is associated with multiple adverse effects, including a higher risk of caries, root resorption, and gingival recession. A number of attempts have been made to identify new methods to accelerate tooth movement and thereby reduce treatment times and side effects. The rate of tooth movement is known to depend on the turnover rate of bone remodeling. During orthodontic tooth movement, alterations in alveolar bone density can accelerate the rate of tooth movement. Recent studies1314 suggested that administration of concentrated PRP could effectively accelerate tooth movement. Güleç et al.14 reported that rats that received PRP showed lower alveolar bone density and increased osteoclast activity. However, the limitation of the rat model was that autologous PRP was not used because the blood volume of the rats was insufficient for self-produced PRP. Additionally, an immune reaction could result in erroneous results. A literature review showed that no data are available on the use of L-PRP to accelerate orthodontic tooth movement. Therefore, the aim of this study was to determine the effect of local administration of L-PRP on accelerating orthodontic tooth movement in rabbits.
This animal experiment was approved by the Animal Ethics Committee, Prince of Songkla University (MOE 0521.11/074). The sample size was determined by G*Power software version 3.1.9.2 (Franz Faul; Universität Kiel, Kiel, Germany). According to the results of a previous study,15 a minimum sample size of 8 animals per group for measuring orthodontic tooth movement (effect size = 1.88) and 5 animals per group for evaluating the osteoclast numbers (effect size = 2.52) was needed to achieve a significance level of 0.05 with 90% power. This study was performed with a randomized split-mouth experimental design.
Twenty-three male New Zealand white rabbits aged 3
to 4 months and weighing 2.5 to 3 kg were included in this study. Five rabbits were used as the control without any intervention. Ten rabbits were randomly divided into 2 groups: the 7-day group (n = 5) and the 14-day group (n = 5), for histomorphometric analysis. The distance of tooth movement was measured in eight rats on days 0, 3, 7, 14, 21, and 28, after which these animals were included in the 28-day group to evaluate histomorphometry analysis (Figure 1). The rabbits were randomly assigned to receive a single injection of L-PRP on one side of the maxilla while the other side served as a control and received an injection of normal saline solution. All injections were administered only once on day 0 in equal volumes with the same technique for the injection and rehabilitation. All experimental procedures were completed under general anesthesia with an intramuscular injection of 25 mg/kg of ketamine hydrochloride (Ketamine–Hameln; Hameln Pharmaceuticals GmbH, Hameln, Germany) and 5 mg/kg xylazine hydrochloride (X-lazine; LBS Laboratory Ltd., Bangkok, Thailand).
A 10-mL sample of autologous blood was collected from each animal via the central auricular artery into a collection tube containing ethylenediaminetetraacetic acid (BD® Vacutainer; Franklin Lakes, NJ, USA). The blood samples were spun in a two-step centrifugation procedure according to a previously reported protocol.16 Briefly, the tubes were centrifuged at 250 ×g for 10 minutes to separate the sample into three fractions: the upper fraction of platelet-containing plasma, the middle fraction containing the buffy coat, and the lower fraction of red blood cells. The top two fractions were collected and centrifuged again at 1,000 ×g for 10 minutes. The platelet-poor plasma in the upper layer was discarded. Precipitated platelets and leukocytes were homogenized in the residual plasma to obtain 1 mL of L-PRP. A 0.5-mL sample of L-PRP was analyzed with a hematology analyzer to determine the concentration of platelets and leukocytes. The remaining 0.5 mL of L-PRP was injected submucosally at the buccal and lingual areas of the first maxillary premolar using a 27-gauge dental needle.
The force was applied using a light-type nickel-titanium closed-coil spring (Dentos, Daegu, Korea) with a length of 13 mm and a diameter of 1.5 mm, between the maxillary first premolar and incisor on each side. The spring was fixed in place via a ligature wire and secured with composite resin on the incisors to prevent sliding. The force was measured using a force gauge (Correx Tension Gauge; Haag-Streit, Koeniz, Switzerland) to generate 100-g of activation. The appliances were monitored at each time point to ensure that they were intact.
Tooth movement distance was measured using silicone impression material (Silagum; DMG Chemisch Pharmazeutische Fabrik GmbH, Hamburg, Germany). Impressions of the rabbits' maxillary dentition were taken at each of the observed periods. These impressions were scanned directly and used to construct three-dimensional models with a 3D scanner (Trios3; 3Shape, Copenhagen, Denmark). The amounts of tooth movement in the digital models were determined using 3Shape Ortho Analyzer™ software ver. 1.7.1.0 (3Shape). Briefly, the occlusal plane was chosen as the reference plane. This plane was created by the cusp tips of the maxillary second premolars and molars. Next, the distance of tooth movement was determined by measuring the linear distance on the 2-dimensional schematic image of the cross-section. The nearest distance between the distal surface of the crown of the first premolar and the mesial surface of the crown of the second premolar was assessed. Measurements were performed with the digital caliper function within 3Shape Ortho Analyzer software.
The rabbits were sacrificed with a lethal dose of thiopental sodium at each time point (0, 7, 14, and 28 days). The maxillae were decalcified with 10% EDTA (Fluka; Sigma-Aldrich Inc., St. Louis, MO, USA) in pH 7 at room temperature for 28 days. Then, the samples were dehydrated with graded alcohol series and embedded in paraffin. The specimens were sagittally sectioned parallel to the long axis of the maxillary first premolar at a thickness of 5 micrometers and stained with hematoxylin and eosin (H&E).
Osteoclasts were counted at the compression side of the maxillary first premolar. The coronal half of the root and the adjacent alveolar bone were determined for histologic analysis because of the tipping movement of the first premolar. In each animal, three sections from the alveolar crest to the middle level were examined to quantify the osteoclast numbers under a light microscope (Carl Zeiss Microscopy GmbH, Jena, Germany) with 20× magnification. An osteoclast was defined as a large multinucleated cell located on or near bone surfaces.
All sample measurements were reassessed by the same investigator at an interval of 2 weeks. Intraclass correlation coefficients were calculated to assess intraobserver reliability. All data are reported as means and standard deviations of each group. The statistical analyses were performed using SPSS ver. 17 (SPSS Inc., Chicago, IL, USA). The Shapiro-Wilk test for the distances of tooth movement and the osteoclast numbers showed that the data were normally distributed. A comparison of the results between the groups was carried out by the independent-sample t-test. A probability value less than 0.05 indicated statistical significance.
The mean concentrations of platelets and leukocytes in the whole blood were 351.14 ± 78.40 × 103 cells/µL and 3.69 ± 0.98 × 103 cells/µL, respectively. After L-PRP preparation, these concentrations increased to 2,314.44 ± 570.82 × 103 cells/µL and 6.87 ± 2.29 × 103 cells/µL (Figure 2). The results demonstrated that L-PRP showed 6.6-fold and 1.9-fold higher concentrations of platelets and leukocytes, respectively.
The intraclass correlation coefficient for the distance of tooth movement was 0.97. The reliability results indicated excellent correlation of measurements. The distance of tooth movement increased from the beginning up to 28 days in both groups, but the L-PRP group showed greater cumulative tooth movement than the control group at all observation times. The cumulative amount of tooth movement in the L-PRP group was significantly greater than that in the control group on day 3 (0.84 ± 0.05 mm vs. 0.76 ± 0.07 mm), day 7 (1.04 ± 0.05 mm vs. 0.94 ± 0.09 mm), day 14 (1.62 ± 0.09 mm vs. 1.39 ± 0.12 mm), day 21 (1.95 ± 0.2 mm vs. 1.64 ± 0.17 mm), and day 28 (2.3 ± 0.32 mm vs. 2.01 ± 0.12 mm) (Figure 3A).
The rates of tooth movement in the L-PRP and control groups increased steeply for the first 7 days. After that, there was a continuous decrease from 14 days to 21 days and then the tooth movement rate remained constant until 28 days. The L-PRP group showed a significantly greater rate of tooth movement than the control group from 0 to 7 days (1.04 ± 0.05 mm vs. 0.94 ± 0.09 mm) and from 7 to 14 days (0.58 ± 0.09 mm vs. 0.45 ± 0.12 mm). However, the rate of tooth movement between the two groups was not significantly different at the intervals of 14–21 days and 21–28 days (Figure 3B).
The osteoclast numbers increased from 7 days to 14 days and subsequently declined at 28 days. The data exhibited a peak number of osteoclasts on day 14. The L-PRP group showed significantly greater osteoclast numbers than the control group on day 7 (10.6 ± 2.07 vs. 7.4 ± 2.30) and day 14 (16.2 ± 3.03 vs. 11.6 ± 3.04), but there was no significant difference on day 28 (4.2 ± 1.78 vs. 3.8 ± 1.48) (Figure 4).
Histologic sections along the compression side of the root of the maxillary first premolar on days 7 and 14 showed hyperemia of the PDL and the adjacent alveolar bone surface. The hyperemia was more noticeable in the L-PRP group, which presented dilated blood vessels congested with numerous blood cells, in comparison with the control group. Moreover, rich cellularity in which a discrete inflammatory infiltrate adjacent to the bone surface was more obvious in the L-PRP group on day 7.
Scalloped surfaces of alveolar bone with few osteoclasts in resorption lacunae were apparent on day 7, while discrete bone resorption with an irregular surface was more observable on day 14. More osteoclasts were detected on the bone surfaces in the L-PRP group than in the control group on days 7 and 14. However, the differences in both groups were not noticeable on day 28 (Figure 5).
Local injections of L-PRP produced accelerated tooth movement. There was significantly more tooth movement in the L-PRP group than in the control group at all time periods. These findings were consistent with the results of previous studies, which reported the positive effects of PRP in promoting rapid tooth movement.1417 The accelerated tooth movement could be attributed to the high concentration of growth factors and cytokines secreted by activated platelets in PRP. The release of these molecules, including platelet-derived growth factor, transforming growth factor-β, and vascular endothelial growth factor (VEGF), influenced the process of bone remodeling to trigger cellular responses in the various cell types, which provided a favorable environment for tooth movement.2 However, our results differed to some extent from those of Rashid et al.,17 who concluded that the rate of tooth movement in the PRP group did not significantly increase in the first week. This may be explained by the presence of leukocytes in the PRP in this study. The leukocytes contributed mainly to the initial burst release of pro-inflammatory cytokines (i.e., IL-1, IL-6, and TNF-α), which could be considered the initiating factor for the cellular and molecular events in the early phase of orthodontic tooth movement.18 It was assumed that the presence of leukocytes in PRP contributed to increased orthodontic tooth movement.
This study showed that tooth movement in the L-PRP group was approximately 1.2 times higher than that in the control group on day 21. According to previous studies, the magnitudes of tooth movement were 1.7-fold higher in a rat model14 and 2.13-fold higher in a dog model.17 The differences between the animal models used to investigate tooth movement can reasonably explain the different results. It is known that tooth movement involves a process of bone remodeling. Therefore, the rate of bone remodeling can be used to quantify the rate of tooth movement. In the rabbit model, the rate of bone turnover in the jaw bones is approximately 2% per year, while the bone remodeling rate in the alveolar bone of canines is estimated to be 20–36% per year.19 It was assumed that a slower tooth movement rate in this study was possibly due to lower bone turnover. Interestingly, a previous study using corticotomy to accelerate tooth movement in a rabbit model15 showed a 1.2-fold increase in tooth movement, similar to the current study. Administration of L-PRP may be an alternative procedure that offers benefits for the acceleration of orthodontic tooth movement.
This study demonstrated a significant increase in tooth movement in the L-PRP group during the first and second weeks, while the differences were not significant in the last two weeks. This may have occurred because of a reduced level of pro-inflammatory cytokines and growth factors over time. According to Schär et al.,9 L-PRP released most of the growth factors and catabolic cytokines at the beginning. They found that the release pattern of IL-1β peaked on day 3 followed by a dramatic decrease on day 7, with almost no further release on day 14. In addition, a previous study found that alveolar bone changes occurred early on day 3 and were more significant on day 7 after force application and an injection of PRP.14 Corresponding to the histological findings, osteoclasts were seen on day 3 and their number peaked on day 7. It was assumed that a single injection of L-PRP may have a transient effect in increasing the rate of tooth movement after injection.
Correspondingly, a significantly higher number of osteoclasts were found in the L-PRP group during the first and second weeks when compared with the control group. Due to the aforementioned reasons, the increased pro-inflammatory cytokines released from the leukocytes in L-PRP included IL-1, IL-6, and TNF-α. These cytokines are involved in the process of bone resorption initiated by cytokine-induced inflammation during orthodontic tooth movement.18 The in vitro study demonstrated that the pro-inflammatory cytokines in L-PRP induce the activation of the NF-κB pathway via the canonical pathway, which is involved in osteoclastogenesis and bone resorptive activity. They also found that L-PRP treatment on cells in vitro upregulated the expression of downstream inflammation-related genes, including cyclooxygenase-2 and inducible nitric oxide synthase, which are known to be important inflammatory mediators, to induce osteoclastic bone resorption.12 Moreover, the role of pro-inflammatory cytokines was related to the process of bone resorption through recruitment of osteoclast precursors from the circulation20 which is in accordance with the histologic finding that showed a higher inflammatory infiltrate in the L-PRP group on day 7.
In addition, the histologic findings in this study demonstrated that the L-PRP group showed greater vascularity than the control group in the first and second weeks. This could be explained by the released angiogenic growth factors in L-PRP, particularly VEGF, which is known as an important mediator of angiogenesis and increased vascular permeability.21 In vitro studies demonstrated that the presence of angiogenic growth factors in PRP promoted vascular growth and stimulated endothelial progenitor cells to form vessel-like structures.1222 In vivo studies also revealed that animals treated with PRP showed an increase in new vessel formation.2223 Interestingly, the VEGF-mediated angiogenesis is related to an indirect increase in bone resorption. New capillaries were formed to enhance the recruitment of osteoclast precursors to the bone surface adjacent to the resorption site.24 In accordance with our study, the numerous differentiated osteoclasts close to the blood vessel presented along the bone surface adjacent to the compressed PDL. Moreover, VEGF is essential for the recruitment, differentiation, and activation of osteoclast precursors and for enhancing osteoclastic bone resorption.2526 Thus, due to the aforementioned reasons, the results of this study could be explained by the increased osteoclast numbers in the L-PRP group.
The peak number of osteoclasts in this study was seen on day 14. These histologic findings were similar to those reported in a rabbit study by Chen et al.15 who found higher osteoclast numbers in the second week. However, their results also showed an initial peak of osteoclast numbers on day 3. They suggested that the first peak of osteoclasts was derived from the local PDL or the bone marrow of the alveolar bone, while the second peak originated from the differentiation of mononuclear cells in peripheral blood.27 However, this study did not investigate histologic findings on day 3.
Although our findings are consistent with those reported by Rashid et al.,17 in that the received PRP group exhibited an increased number of osteoclasts, they did not examine the early stages of histologic findings. However, these results were in contrast with previous findings, in which PRP did not increase the osteoclastic activity. Güleç et al.14 reported that the osteoclast cell counts in the PRP group were less than those in the control group at the first, second, and third weeks despite a higher rate of tooth movement in the PRP group. Akbulut et al.28 also demonstrated that the tartrate-resistant acid phosphatase expressions between the groups were not different at all observation times. One reason for this is that a possible immune reaction from usage of an allogenic PRP injection led to different results. Another possible reason is the absence of leukocytes in PRP, unlike this study.
The inability to characterize the release characteristics of growth factors and cytokines in this study was a limitation because measurements of these molecules were not performed. A better understanding of the expression of various biological molecules may be needed to understand how long these factors maintain biologic activity. In addition, the results from this animal experiment cannot be directly extrapolated to a clinical situation. Thus, further studies are needed to investigate the efficacy of L-PRP administration for accelerated orthodontic tooth movement in clinical trials as well as to determine the frequency of application for optimal effectiveness to enhance orthodontic tooth movement.
From these results, a single submucosal injection of L-PRP may have a positive influence on orthodontically induced tooth movement and may result in transient increases in the rate of tooth movement and higher osteoclast numbers for 14 days.
Cumulative orthodontic tooth movement in the group that received L-PRP was significantly greater in all time periods.
The injection of L-PRP significantly increased the rate of tooth movement during the first 14 days, but there was no significant difference in the rate of tooth movement after day 14.
The injection of L-PRP significantly increased the osteoclast numbers on days 7 and 14.
Figures and Tables
Figure 1
Schematic of the study design and timeline.
L-PRP, Leukocyte-platelet-rich plasma; NSS, normal saline solution.
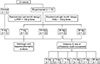
Figure 2
(A) Platelet and (B) leukocyte concentrations in the whole blood and leukocyte-platelet-rich plasma (L-PRP).
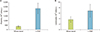
Figure 3
A, Cumulative distance of tooth movement in the leukocyte-platelet-rich plasma (L-PRP) and control groups. B, Rate of tooth movement in the L-PRP and control groups.
*p < 0.05; **p < 0.01; ***p = 0.001.
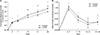
Figure 4
The osteoclast numbers in the leukocyte-platelet-rich plasma (L-PRP) and control groups.
*p < 0.05.
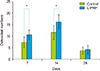
Figure 5
Histological sections showing the compression side of the maxillary first premolar in the leukocyte-platelet-rich plasma (L-PRP) (A, C, E, G) and control groups (B, D, F, H) on days 0, 7, 14 and 28, respectively (H&E; ×20). The inflammatory cells (black arrows) and osteoclasts (arrowheads) were magnified in the images (C, E) at the bottom right corner (40-times magnification).
PDL, Periodontal ligament; Alv, alveolar bone.
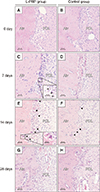
Notes
References
1. Teixeira CC, Khoo E, Tran J, Chartres I, Liu Y, Thant LM, et al. Cytokine expression and accelerated tooth movement. J Dent Res. 2010; 89:1135–1141.


2. Andrade I Jr, Taddei SRA, Souza PEA. Inflammation and tooth movement: the role of cytokines, chemokines, and growth factors. Semin Orthod. 2012; 18:257–269.


3. Sánchez AR, Sheridan PJ, Kupp LI. Is platelet-rich plasma the perfect enhancement factor? A current review. Int J Oral Maxillofac Implants. 2003; 18:93–103.
4. Fernandez-Moure JS, Van Eps JL, Cabrera FJ, Barbosa Z, Medrano Del Rosal G, Weiner BK, et al. Platelet-rich plasma: a biomimetic approach to enhancement of surgical wound healing. J Surg Res. 2017; 207:33–44.


5. Oryan A, Alidadi S, Moshiri A. Platelet-rich plasma for bone healing and regeneration. Expert Opin Biol Ther. 2016; 16:213–232.


6. Rodriguez IA, Growney Kalaf EA, Bowlin GL, Sell SA. Platelet-rich plasma in bone regeneration: engineering the delivery for improved clinical efficacy. Biomed Res Int. 2014; 2014:392398.


7. Sundman EA, Cole BJ, Fortier LA. Growth factor and catabolic cytokine concentrations are influenced by the cellular composition of platelet-rich plasma. Am J Sports Med. 2011; 39:2135–2140.


8. Yuan T, Guo SC, Han P, Zhang CQ, Zeng BF. Applications of leukocyte- and platelet-rich plasma (L-PRP) in trauma surgery. Curr Pharm Biotechnol. 2012; 13:1173–1184.


9. Schär MO, Diaz-Romero J, Kohl S, Zumstein MA, Nesic D. Platelet-rich concentrates differentially release growth factors and induce cell migration in vitro. Clin Orthop Relat Res. 2015; 473:1635–1643.


10. Anitua E, Zalduendo M, Troya M, Padilla S, Orive G. Leukocyte inclusion within a platelet rich plasmaderived fibrin scaffold stimulates a more pro-inflammatory environment and alters fibrin properties. PLoS One. 2015; 10:e0121713.


11. Pifer MA, Maerz T, Baker KC, Anderson K. Matrix metalloproteinase content and activity in low-platelet, low-leukocyte and high-platelet, high-leukocyte platelet rich plasma (PRP) and the biologic response to PRP by human ligament fibroblasts. Am J Sports Med. 2014; 42:1211–1218.


12. Yin W, Qi X, Zhang Y, Sheng J, Xu Z, Tao S, et al. Advantages of pure platelet-rich plasma compared with leukocyte- and platelet-rich plasma in promoting repair of bone defects. J Transl Med. 2016; 14:73.


13. Liou EJW. The development of submucosal injection of platelet rich plasma for accelerating orthodontic tooth movement and preserving pressure side alveolar bone. APOS Trends Orthod. 2016; 6:5–11.


14. Güleç A, Bakkalbaşı BÇ, Cumbul A, Uslu Ü, Alev B, Yarat A. Effects of local platelet-rich plasma injection on the rate of orthodontic tooth movement in a rat model: a histomorphometric study. Am J Orthod Dentofacial Orthop. 2017; 151:92–104.


15. Chen YW, Wang HC, Gao LH, Liu C, Jiang YX, Qu H, et al. Osteoclastogenesis in local alveolar bone in early decortication-facilitated orthodontic tooth movement. PLoS One. 2016; 11:e0153937.


16. Jia WT, Zhang CQ, Wang JQ, Feng Y, Ai ZS. The prophylactic effects of platelet-leucocyte gel in osteomyelitis: an experimental study in a rabbit model. J Bone Joint Surg Br. 2010; 92:304–310.
17. Rashid A, ElSharaby FA, Nassef EM, Mehanni S, Mostafa YA. Effect of platelet-rich plasma on orthodontic tooth movement in dogs. Orthod Craniofac Res. 2017; 20:102–110.


18. Alhashimi N, Frithiof L, Brudvik P, Bakhiet M. Orthodontic tooth movement and de novo synthesis of proinflammatory cytokines. Am J Orthod Dentofacial Orthop. 2001; 119:307–312.


19. Ibrahim AY, Gudhimella S, Pandruvada SN, Huja SS. Resolving differences between animal models for expedited orthodontic tooth movement. Orthod Craniofac Res. 2017; 20 Suppl 1:72–76.


20. Kaplanski G, Marin V, Montero-Julian F, Mantovani A, Farnarier C. IL-6: a regulator of the transition from neutrophil to monocyte recruitment during inflammation. Trends Immunol. 2003; 24:25–29.


21. Dai J, Rabie AB. VEGF: an essential mediator of both angiogenesis and endochondral ossification. J Dent Res. 2007; 86:937–950.


22. Mammoto T, Jiang A, Jiang E, Mammoto A. Platelet rich plasma extract promotes angiogenesis through the angiopoietin1-Tie2 pathway. Microvasc Res. 2013; 89:15–24.


23. Lyras DN, Kazakos K, Verettas D, Polychronidis A, Tryfonidis M, Botaitis S, et al. The influence of platelet-rich plasma on angiogenesis during the early phase of tendon healing. Foot Ankle Int. 2009; 30:1101–1106.


24. Cheung WY, Liu C, Tonelli-Zasarsky RM, Simmons CA, You L. Osteocyte apoptosis is mechanically regulated and induces angiogenesis in vitro. J Orthop Res. 2011; 29:523–530.


25. Nakagawa M, Kaneda T, Arakawa T, Morita S, Sato T, Yomada T, et al. Vascular endothelial growth factor (VEGF) directly enhances osteoclastic bone resorption and survival of mature osteoclasts. FEBS Lett. 2000; 473:161–164.


26. Kohno S, Kaku M, Tsutsui K, Motokawa M, Ohtani J, Tenjo K, et al. Expression of vascular endothelial growth factor and the effects on bone remodeling during experimental tooth movement. J Dent Res. 2003; 82:177–182.

