Abstract
Purpose
Materials and Methods
Results
Figures and Tables
Fig. 1
Expression of miR-338-3p in ovarian cancer tissues and cell clines. (A) Real-time quantitative polymerase chain reaction (RT-qPCR) assay was performed to examine the expression of miR-338-3p in 54 ovarian cancer tissues (divided into two groups according to resistance to cisplatin) and adjacent normal tissues. (B) The expression levels of miR-338-3p in human normal ovarian cancer cells (IOSE-80) and ovarian cancer cells (A2780 and SKOV3), as well as cisplatin resistant ovarian cancer cells (A2780/DDP and SKOV3/DDP), were assessed by RT-qPCR. (C) The overall survival curve of ovarian cancer patients was analyzed with Kaplan-Meier analysis. *p<0.05; **p<0.01; ***p<0.001.
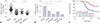
Fig. 2
Increased expression of miR-338-3p enhances sensitivity to cisplatin among ovarian cancer cells. A2780/DDP and SKOV3/DDP cells were transfected with miR-NC or miR-338-3p. (A) The relative expression levels of miR-338-3p in transfected cisplatin-resistant ovarian cancer cells was evaluated via real-time quantitative polymerase chain reaction (RT-qPCR). (B–E) IC50 values of cisplatin, as well as cell viability in A2780/DDP and SKOV3/DDP cells, were measured by MTT assay. (F) Apoptotic rate was assessed by flow cytometry in A2780/DDP and SKOV3/DDP cells after transfection. (G and H) Protein levels of cleaved-caspase-3, Bcl-2, and Bax were determined in A2780/DDP and SKOV3/DDP cells by Western blot. (I and J) Cell migration and invasion were evaluated in transfected A2780/DDP and SKOV3/DDP by transwell assay (×100, stained with 0.1% crystal violet). (K and L) Western blot assay was used to assess the expression levels of epithelial-mesenchymal transition-related proteins (E-cadherin, N-cadherin and Vimentin). *p<0.05. GAPDH, glyceraldehyde-3-phosphate dehydrogenase.
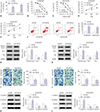
Fig. 3
WNT2B is a target of miR-338-3p. (A) The binding sites of miR-338-3p on the 3′UTR of WNT2B was predicted with bioinformatics and its mutant are shown. (B and C) Luciferase activity was estimated in A2780/DDP and SKOV3/DDP co-transfected with WNT2B 3′UTR-WT/MUT luciferase reporters and miR-338-3p/miR-NC. (D–F) The protein and mRNA levels of WNT2B were measured in treatment-responsive patient tissues or cells (A2780 and SKOV3) and treatment-resistant patients tissues and cells (A2780/DDP and SKOV3/DDP), as well as matched normal controls, utilizing real-time quantitative polymerase chain reaction (RT-qPCR) and Western blot assay. (G) The relationship between miR-338-3p and WNT2B was analyzed. (H–K) Gain-of-function experiments were performed to detect mRNA and protein expression levels of WNT2B in A2780/DDP and SKOV3/DDP cells transfected with miR-NC, miR-338-3p, miR-338-3p+pcDNAN or miR-338-3p+WNT2B. *p<0.05; ***p<0.001. GAPDH, glyceraldehyde-3-phosphate dehydrogenase.
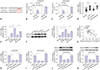
Fig. 4
WNT2B knockdown weakens resistance to cisplatin in ovarian cancer cells. A2780/DDP and SKOV3/DDP cells were transfected with sh-NC or sh-WNT2B. (A and B) real-time quantitative polymerase chain reaction (RT-qPCR) and Western blot assays were carried out to evaluate expression levels of WNT2B in transfected ovarian cancer cells. (C–F) MTT analysis was utilized to assess IC50 values for cisplatin and cell viability in ovarian cancer cells post-transfection. (G) Apoptosis of transfected ovarian cancer cells was determined by flow cytometry. (H and I) Levels of apoptosis-related proteins (cleaved-caspase-3, Bcl-2, and Bax) were measured with Western blot. (J and K) Transwell assay was performed to detect the motility of ovarian cancer cells. (L and M) Western blot was used to assess the expression levels of E-cadherin, N-cadherin, and Vimentin. *p<0.05. GAPDH, glyceraldehyde-3-phosphate dehydrogenase.
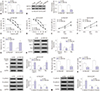
Fig. 5
Upregulation of miR-338-3p increases cisplatin sensitivity in resistant ovarian cancer cells by negatively regulating WNT2B. A2780/DDP and SKOV3/DDP were transfected with miR-NC, miR-338-3p, miR-338-3p+pcDNA, or miR-338-3p+WNT2B. (A and B) IC50 values for cisplatin was measured by MTT assay in transfected A2780/DDP and SKOV3/DDP cells treated with various doses of cisplatin for 48 h. (C and D) MTT analysis was used to measure the viability of transfected A2780/DDP and SKOV3/DDP cells. (E) Apoptosis rates of 2780/DDP and SKOV3/DDP cells were detected by flow cytometry assay. (F and G) Western blot assay was conducted to test the protein expression levels of apoptosis-related proteins in transfected cells. (H and I) The migration and invasion capabilities in transfected ovarian cancer cells were measured by transwell assay. (J and K) The protein expression levels of epithelial-mesenchymal transition-related in transfected A2780/DDP and SKOV3/DDP cells were detected by Western blot assay. *p<0.05. GAPDH, glyceraldehyde-3-phosphate dehydrogenase.
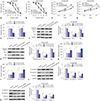
Fig. 6
Elevated expression of miR-338-3p suppresses tumor growth and enhances cisplatin sensitivity by affecting WNT2B expression in vivo. (A and B) Growth curves for tumor volume and weight were measured in xenograft tumors from nude mice treated with PBS or cisplatin (5.0 mg/kg, once every three days). (C and D) RT-qPCR and Western blot assay were performed to measure the expression levels of miR-338-3p and WNT2B in resected tumor tissues, respectively. *p<0.05. GAPDH, glyceraldehyde-3-phosphate dehydrogenase.
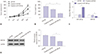
Notes
AUTHOR CONTRIBUTIONS
Conceptualization: Zhenghong Liu.
Data curation: Jia Gao.
Formal analysis: Qin Niu.
Funding acquisition: Qiao Wang.
Investigation: Zhenghong Liu.
Methodology: Qin Niu.
Project administration: Zhenghong Liu.
Resources: Jia Gao and Qiao Wang.
Software: Qiao Wang.
Supervision: Zhenghong Liu and Qin Niu.
Validation: Qin Niu and Jia Gao.
Visualization: Qin Niu and Qiao Wang.
Writing—original draft: Qin Niu and Zhenghong Liu.
Writing—review & editing: Qin Niu, Zhenghong Liu, and Qiao Wang.
References



















